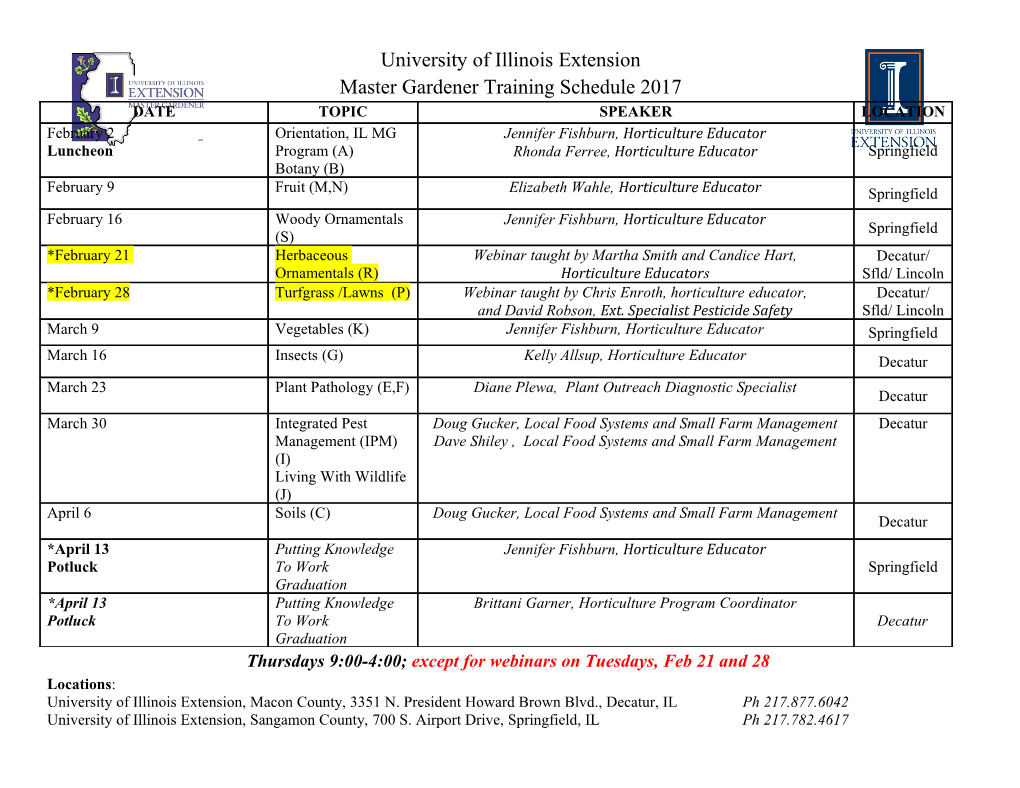
<p>September 2004 doc.: IEEE 802.11-04-0941-00-000n</p><p>IEEE 802.11 Wireless LANs HNS Proposal for 802.11n Physical Layer Design </p><p>Date: August 13, 2004</p><p>Authors: M. Eroz, F. Sun, Y. Jiang, J. Song, K. Karimullah and L. Lee</p><p>Hughes Network Systems 11717 Exploration Lane, Germantown MD 20876 301-428-5500 Fax: 301-601-4211 [email protected], [email protected], [email protected] </p><p>Abstract</p><p>Hughes Network Systems PHY proposal for IEEE 802.11n is based on using the physical media dependent (PMD) layer of the standard 802.11a at 5 GHz. This would easily extend to the 2.4 GHz band application. The main approach of this proposal is to employ LDPC coding over short frame lengths and yet achieve superior performance over convolutional or Turbo codes. Further in the spatial multiplexed high throughput (HT) mode, good performance is achieved without the channel information known at the transmitter where equal power is distributed over the antennas. Lack of feedback also makes the overall throughput more efficient. The PLCP preamble is identical in duration to the 802.11a Standard, but spreads over multiple antennas, providing a 16- microsecond interval for channel matrix measurement, along with the usual coarse and fine frequency acquisition functions. In the single antenna mode the system would be identical to the 802.11a Standard. Similarly, the pilots during data transmission are identical but are scrambled by the same 127-state long PN codes, with different initial offset for each additional antenna. Here also the system becomes identical to Standard for a single antenna transmission. HNS believes that this proposal will provide HT modes that will exceed the spectral efficiency criteria and deliver 100Mbps MSDU throughput in a 20MHz channel. It will also inherit key features of 802.11a which are crucial to backward compatibility requirement. </p><p>Submission page 1 M. Eroz, et al, Hughes Network Systems September 2004 doc.: IEEE 802.11-04-0941-00-000n</p><p>Proposed IEEE 802.11n Air Interface:</p><p>The physical media dependent layer (PMD) is built upon 802.11a. Some of the main characteristics are summarized here. For other related details refer to IEEE Std. 802.11a-1999. </p><p> OFDM Modulation with combination of PSK and QAM types 20 MHz Wide Channel 0.3125 MHz (20MHz/64) channel spacing based on a 64 point FFT 48 sub-carriers for data, 4 sub-carriers for Pilots 0.8 microsecond cyclic-prefix 4 usec for OFDM symbol </p><p>In the absence of HT MAC design, we have assessed below that a new MAC should be able to deliver 55-60 percent PHY-MAC efficiency in an AP-STA, 1500B MPDU throughput test. See [“Goodput Enhancement of IEEE 802.11a Wireless LAN via Link Adaptation”, Daji Qiao and Sunghyun Choi, IEEE Proceedings of ICC 2001, Helsinki, Finland.</p><p>Preamble H MPDU SI Preamble H Ack SI FS FS</p><p>Figure 1. Timing relationship for an AP-STA throughput test.</p><p>The time between the two markers is for one good frame transmission, including the acknowledgement from the receiver. The PLCP preamble is 16 usec long, the header (H) is 4 usec, MPDU is 1500B, Acknowledgement data is one OFDM symbol long, and a Short Inter- frame Space (SIFS) is assumed to be 8 usec long (reduced from16 usec). With good SNR conditions and continuous offered load this will deliver a throughput efficiency of 58 percent, when the 72 Bytes/ OFDM symbol, 180Mbps PHY mode is selected. Consequently > 100Mbps throughput could be achieved at top of MAC. </p><p>Preamble and Pilot Approaches:</p><p>In 802.11a OFDM modulation there are 53 frequency bins. These are labeled from –26, … -1,0,1, … 26. The zero location will not be used for transmission. There are two flavors to the above overheads, namely the pilot and the preamble also called the Physical Layer Control Protocol (PLCP) preamble. We build the 802.11n preamble on the same principals. Refer to section 17.3.3 “PLCP Preamble” of 802.11a Std-1999. </p><p>The 802.11a preamble, which provides means for initial frequency acquisition, coarse and fine, and timing synchronization, channel estimation, as well as diversity, is of 16 usec. It is divided into two portions, 8 usec each. </p><p>For 802.11n, in the first portion, instead of transmitting only 12 carriers as in 802.11a, we transmit 12 carriers per TX antenna. For each TX path, IFFT process and the Guard time application remains identical to the 11a Standard. For the first antenna, the 12 carriers assume </p><p>Submission page 2 M. Eroz, et al, Hughes Network Systems September 2004 doc.: IEEE 802.11-04-0941-00-000n the same frequency as the 802.11a. As shown in Figure 2, each of the other antennas will use frequencies which are one, two, or three offset from the first antenna. The spectrum of the resulting TX signal will therefore have 12.Ntx sub-carriers during this time, Ntx being the number of TX antennas. Similarly, during the long training sequence portion, the long sequence bits are spread equally over Ntx antennas, alternating. In this manner, the receiver will be able to make channel estimation measurements during the 16 usec preamble, along with the usual acquisition procedures. Since the details of preamble design are given in the IEEE 802.11a Std-1999 section 17.3.3 and Annex G.3, these details are withheld at this time.</p><p>Section 17.3.5.8 and 9 of the 11a Standard define the Pilot tones embedded in the data portion. These tones aid in keeping the coherent detection process robust against frequency offsets and phase noise. In our proposal we transmit the same frequency set from each of the TX antenna, however different initial offsets are used in the 127 bit long scrambling sequence are used such that they are practically orthogonal to each other. This prevents transmitting coherently at the source eliminating formation of beam nulls due to multipath, and preserves each TX antenna transmission independent of other, hence omni-directional beams, as would be the case for the data portion. Measurements made with these pilots will have diversity advantage. </p><p>Note that when only one TX antenna is used, the above approaches to preamble and pilots will become identical to the 11a standard and fully backward compatible. </p><p>Proposed Modulation and Coding Schemes</p><p>The proposed physical layer approach is based on the same OFDM construction used in 802.11a/g. The modulation on the individual carriers can be BPSK, QPSK, 8-PSK, 16-QAM, 32-QAM, and 64-QAM, depending on the antenna configuration and operating speed as specified in the proposed rate set table. The forward Error Correction (FEC) coding scheme is based on Low-Density Parity Check (LDPC) codes of rate either 1/2 or 2/3. The LDPC codes have a minimum block length of 192 bits, which is 4 times the number of information carrying subcarriers in an OFDM symbol. For longer bursts, the LDPC codes with length integer multiple of the 192 bits are derived from the minimum length codes, to exploit the common architecture of the encoder and decoder.</p><p>LDPC encoded bits fill a two-dimensional interleaver matrix column by column. The matrix has 3 columns for 8-PSK modulation, 4 columns for 16-QAM modulation, 5 columns for 32-QAM modulation and 6 columns for 64-QAM modulation. As shown in Figure 3, each row of the matrix is mapped to a constellation symbol according to the bit labelings below. With QPSK no interleaving is performed. The span of the interleaving is one LDPC frame length.</p><p>At the receiver, LDPC decoder and detector iterate soft information back and forth to improve the performance. One detector iteration is performed after every three LDPC decoder iteration.</p><p>The parity check matrices for the rate 1/2 and rate 2/3 code for the 192 block length are shown in th th Tables 1 and 2, respectively. The i row in the tables gives the check nodes connected to the i bit node.</p><p>Submission page 3 M. Eroz, et al, Hughes Network Systems c e s u</p><p>8</p><p>=</p><p> n o i t a</p><p> r September 2004 doc.: IEEE 802.11-04-0941-00-000n u D 1+ j -1- j -1- j e 1+ j -1- j 1+ j -1- j -1- j 1+ j 1+ j 1+ j 1+ j l b m a e r P</p><p> c - 26 e Δf + 26 s u</p><p>IEEE 802.11a Standard Short Training Preamble (i.e. first 8 usec) from one TX Antenna 8</p><p>=</p><p> n o i t a First Antenna (s0) Other Antennas (s1, s2 and s3) r u D e l b 1+ j -1- j 1+ j -1- j -1- j 1+ j 1.0 -1- j -1- j 1+ j 1+ j 1+ j 1+ j m a e r P Δf c e Proposed: Short Training Preamble (First 8 usec) over one ‘First’ and Three ‘Other’ Antennas s u</p><p>8</p><p>=</p><p>First Antenna (s0) Other Antennas (s1, s2 and s3) n o i t a r u</p><p> d 1 1 -1 -1 1 1 ...... </p><p>L-26,26 per section 17.3.3 Std 802.11a -1999 ...... -1 1 1 1 1 e</p><p> l . b m a e r (-26) P 0.3125 MHz Δf (26) Proposed: Long Preamble Sequence Spread Sequentially over the Four TX Antennas</p><p>Figure 2. HNS Preamble Design Approach </p><p>Q Q 100 . . . . 0010 1010 1000 0000 10 110 00 000 . . . . 010 0110 1110 1100 0100 I 001 I . . . . 0111 1111 1101 0101 011 11 01 101 . . . . 111 0011 1011 1001 0001 ...... 000000 000001 000011 000010 000110 000111 000101 000100 11011 11001 01001 01011 ...... 010000 010001 010011 010010 010110 010111 010101 010100 10001 10000 11000 01000 00000 00001 ...... 011000 011001 011011 011010 011110 011111 011101 011100 ...... 10011 10010 11010 01010 00010 00011 001000 001001 001011 001010 001110 001111 001101 001100</p><p>...... 101000. 101001. 101011. 101010. 101110. 101111. 101101. 101100. 10111 10110 11110 01110 00110 00111 ...... 111000 111001 111011 111010 111110 111111 111101 111100 10101 10100 11100 01100 00100 00101 ...... 110000 110001 110011 110010 110110 110111 110101 110100 11111 11101 01101 01111 ...... 100000 100001 100011 100010 100110 100111 100101 100100</p><p>Figure 3. Constellation Bit Labeling</p><p>Submission page 4 M. Eroz, et al, Hughes Network Systems September 2004 doc.: IEEE 802.11-04-0941-00-000n</p><p>Table 1. Parity Check Matrix for the Rate ½ code (N=192)</p><p>20 33 77 2 31 27 32 33 17 83 48 41 59 40 33 34 89 4 92 55 46 78 34 35 58 82 27 42 88 22 35 36 32 26 67 69 71 51 36 37 86 40 24 31 76 46 37 38 24 0 33 51 84 7 38 39 58 23 84 12 37 64 39 40 64 5 62 73 17 43 40 41 81 40 60 35 79 68 41 42 59 9 82 7 11 93 42 43 53 82 54 62 60 52 43 44 29 59 79 18 21 65 44 45 46 54 45 43 66 47 45 46 91 78 28 1 41 74 46 47 90 58 87 42 16 48 47 48 5 35 34 14 76 10 48 49 68 45 37 42 52 77 49 50 31 38 63 47 30 63 50 51 83 55 84 29 30 69 51 52 16 30 75 6 4 25 52 53 89 77 48 9 94 3 53 54 6 3 65 66 49 71 54 55 70 8 87 39 45 95 55 56 90 41 29 86 12 22 56 57 17 56 95 19 18 74 57 58 52 34 21 22 26 13 58 59 86 60 0 14 13 23 59 60 3 38 93 68 54 81 60 61 28 61 55 15 33 72 61 62 12 70 19 66 14 53 62 63 5 36 92 65 92 57 63 64 73 72 56 0 1 64 65 36 47 64 1 2 65 66 78 8 2 2 3 66 67 83 76 85 3 4 67 68 43 85 24 4 5 68 69 70 23 27 5 6 69 70 2 15 67 6 7 70 71 1 91 39 7 8 71 72 38 71 20 8 9 72 73 28 67 80 9 10 73 74 9 49 32 10 11 74 75 36 8 50 11 12 75 76 44 19 94 12 13 76 77 4 1 50 13 14 77 78 75 10 7 14 15 78 79 51 49 25 15 16 79 80 13 81 88 16 17 80 81 34 50 25 17 18 81 82 11 10 57 18 19 82 83 69 75 95 19 20 83 84 44 57 91 20 21 84 85 16 74 26 21 22 85 86 89 32 62 22 23 86 87 80 6 61 23 24 87 88 63 73 79 24 25 88 89 72 39 0 25 26 89 90 21 61 15 26 27 90 91 85 88 94 27 28 91 92 11 20 87 28 29 92 93 18 53 56 29 30 93 94 93 90 80 30 31 94 95 35 37 44 31 32 95</p><p>Submission page 5 M. Eroz, et al, Hughes Network Systems September 2004 doc.: IEEE 802.11-04-0941-00-000n</p><p>Table 2. Parity Check Matrix for rate 2/3 code (N = 192)</p><p>13 22 51 31 43 50 14 15 11 55 32 12 29 25 15 16 59 3 61 54 47 7 16 17 38 54 18 62 43 36 17 18 21 17 45 13 15 44 18 19 57 26 16 30 44 34 19 20 16 0 21 0 28 51 20 21 39 15 56 29 13 33 21 22 43 3 41 11 10 51 22 23 54 27 40 7 29 35 23 24 39 6 55 52 27 41 24 25 35 55 36 25 27 47 25 26 19 39 52 4 2 21 26 27 31 36 30 4 61 44 27 28 61 52 60 32 46 30 28 29 39 58 3 32 63 58 29 30 23 22 45 2 18 15 30 31 30 25 20 14 53 16 31 32 25 42 55 22 9 4 32 33 37 56 11 10 43 21 33 34 20 50 59 53 12 45 34 35 52 32 4 48 7 10 35 36 2 43 47 34 59 8 36 37 5 57 60 3 40 1 37 38 27 20 11 9 55 17 38 39 38 63 35 46 31 59 39 40 23 15 57 9 16 18 40 41 40 0 2 35 34 51 41 42 25 62 19 15 28 48 42 43 41 37 8 53 3 37 43 44 47 13 3 8 19 24 44 45 24 61 49 33 44 42 45 46 48 37 24 20 1 12 46 47 31 42 52 36 24 32 47 48 5 1 56 42 18 12 48 49 51 57 29 26 43 20 49 50 57 17 47 49 28 9 50 51 16 19 1 35 44 46 51 52 5 10 45 9 61 40 52 53 1 61 26 14 50 39 53 54 25 48 14 49 17 14 54 55 19 45 54 12 6 36 55 56 6 32 22 7 20 21 56 57 24 5 33 11 28 62 57 58 29 14 62 8 6 48 58 59 2 1 34 53 63 36 59 60 50 6 4 62 46 0 60 61 34 33 17 13 38 5 61 62 8 55 58 51 49 8 62 63 23 33 16 28 44 63 63 7 6 37 46 2 10 46 50 63 60 23 42 29 38 60 18 17 30 11 50 18 38 9 58 60 22 41 21 56 52 54 4 41 22 27 57 42 49 53 33 45 40 49 26 0 0 1 14 41 10 1 2 56 59 63 2 3 7 13 58 3 4 12 37 39 4 5 62 60 56 5 6 23 24 30 6 7 0 19 27 7 8 40 26 38 8 9 31 54 28 9 10 59 15 47 10 11 48 35 23 11 12 53 31 34 12 13 58 5 26 13 14</p><p>Submission page 6 M. Eroz, et al, Hughes Network Systems September 2004 doc.: IEEE 802.11-04-0941-00-000n</p><p>Summary of the MIMO Transmission System Configuration</p><p>The system configuration include n = 2, 3, or 4 TX antenna cases, and the m = 2, 3, or 4 for the receive antennas. </p><p>NLOS Model for B, D and E are used in simulation with the Florescent light effects for Model D&E. </p><p>There are three sections to the simulation module:</p><p>• Coding and BB TX module – Applies appropriate padding to info bits at encoder input as needed – Fixed 192 Transmission (coded) bit blocks into LDPC encoder – Perform row-column interleaving – generate PSK/QAM modulation symbols • OFDM and Channel Model – Arranges into transmission vector for 3 or 4 TX antennas – Converts modulation symbol stream into OFDM symbols with cyclic prefix, 4 usec/OFDM Symbol – Runs through channel model and impairments as applicable (IM) – Detects OFDM signals on each of the RX antenna – Performs MIMO Channel estimation from the preamble – Delivers demodulated samples from each RX antenna to MAP detector – Delivers the Channel estimation results to the MAP detector • MAP Detector/LDPC Decoding – Performs iterative detection /LDPC decoding on the received symbols – Outputs LDPC decoded bits after N iterations, N = 30 for the results shown below</p><p>The block diagram of the simulation model for a general n transmit, m receive configuration is shown in Figure 4 below.</p><p>Submission page 7 M. Eroz, et al, Hughes Network Systems September 2004 doc.: IEEE 802.11-04-0941-00-000n</p><p>Information bits </p><p>In x1 x2 x T x = [ … n] PA = Rapp’s model, p=3 MIMO LDPC Block Formatter MIMO Preambles IFFT Prefix Digital -RF-PA x1 Preamble LDPC Insert Attachment & Encoder Pilots IFFT Prefix Digital -RF-PA 1:31:n OFDM y2 Symbol . OFDM Demux . PSK/QAM Symbol . Modulator Generator IFFT Prefix Digital -RF-PA y = Ax + n, x (frequency domain) n ‘A’ per 802.11n Channel Model T y = [y1 y2… ym ] LNA NF = 10dB LDPC DECODER RF-Digital MAP PSK/QAM FFT Remove P/fix y1 (LLR) OFDM ...... Iterative Symbol . . .. Detector DETECTION .. Reconstruct . PSDU FFT Remove P/fix RF-Digital ym</p><p> out iteration Prefix Timing/Channel Estimation/Symbol Timing/ Frequency/PhaseAcquisition/Tracking Information bits Channel Estimates</p><p>Figure 4. Block Diagram of Simulation Model</p><p>Proposed Rate Sets</p><p>The proposed rate sets depends on number of transmit antennas. In the single antenna case, the rate set is identical to the 802.11a standard. Rate sets for the 2, 3 and TX antennas cases are listed in the table. The physical layer information rates shown are the net information rate supported by the modulation, coding and MIMO system. The MAC layer information rates are obtained by assuming 60 percent the MAC layer efficiency. It is shown that for 4 TX antenna case, the maximum MAC layer speed can be as high as 144 Mbps and that for 3 TX antenna case, the MAC layer speed can reach 108 Mbps, both meeting the requirements. For the 2 TX antenna case, the maximum MAC speed is 72 Mbps, while the physical layer speed is 108 Mbps, or twice of the 802.11a standard.</p><p>Submission page 8 M. Eroz, et al, Hughes Network Systems September 2004 doc.: IEEE 802.11-04-0941-00-000n</p><p>No. of TX Modulation Transmit bits Code Rate Info. Bytes per PHY Info MAC Info Rate Antennas Type per channel use channel. use Rate (Mbps) (Mbps) @60% of PHY Rate</p><p>4 BPSK 192 1/2 12 30 18</p><p>2/3 18 45 27</p><p>QPSK 384 1/2 24 60 36</p><p>8-PSK 576 1/2 36 90 54</p><p>16-QAM 768 1/2 48 120 72</p><p>32-QAM 960 1/2 60 150 90</p><p>64-QAM 1152 1/2 72 180 108</p><p>2/3 96 240 144</p><p>3 QPSK 288 1/2 18 45 27</p><p>2/3 24 60 36</p><p>16-QAM 576 1/2 36 90 54</p><p>2/3 48 120 72</p><p>64-QAM 864 1/2 56 140 84</p><p>2/3 72 180 108</p><p>2 BPSK 96 1/2 6 15 9</p><p>QPSK 192 1/2 12 30 18</p><p>8-PSK 288 1/2 18 45 27</p><p>16-QAM 384 1/2 24 60 36</p><p>32-QAM 480 1/2 30 75 45</p><p>64-QAM 576 1/2 36 90 54</p><p>2/3 48 120 72</p><p>PER-vs-SNR Performance: </p><p>The packet error rate performance for the the HNS proposal for the Channel Models B. D. and E are shown in the Figures 5, 6 and 7 below, respectively, for selected rates with 4x4 and 3x3 antenna configurations. Only the performance of LDPC codes of length 192 bits are presented, since they are the worst case. As a reference, the packet error rate performance of selected modulation/coding in AWGN channel are also presented in a separate Figure 8. Here, hyperthetical 2x2, 3x3, and 4x4 channel matrices are specified per disclosure document.</p><p>Conclusions</p><p>From these figures, we can conclude that </p><p> The proposed scheme is not very sensitive to the channel model variation. The rough range of 18-19 dB adaptation range between the 144 Mbps (64-QAM, rate 2/3) and 36 Mbps (QPSK, rate ½) implies that a range improvement of about 3.5 in a Non-LOS propogation environment can be achieved by data rate reduction factor of 4. The 36 Mbps (60 Mbps PHY) case is better than the maximum speed of 802.11a standard.</p><p>Submission page 9 M. Eroz, et al, Hughes Network Systems September 2004 doc.: IEEE 802.11-04-0941-00-000n</p><p>Channel Model B 1.0E+00</p><p>4x4, QPSK 3x3, QPSK R=1/2 R=2/3 e</p><p> t 3x3, 16QAM</p><p> a 1.0E-01</p><p>R R=1/2</p><p> r o r r E</p><p> t e k c</p><p> a 1.0E-02 P 4x4, 8PSK R=1/2 3x3, 16QAM 4x4, 64QAM R=2/3 R=2/3</p><p>1.0E-03 2.0 6.0 10.0 14.0 18.0 22.0 26.0 30.0 Es/No (dB) Figures 4. Performance of selected rate sets in Channel Model B.</p><p>Channel Model D 1.0E+00 3x3, QPSK 4x4, QPSK R=2/3 R=1/2 3x3, 16QAM R=1/2 e t</p><p> a 1.0E-01 R</p><p> r o r r E</p><p> t e k c</p><p> a 1.0E-02 P</p><p>3x3, 16QAM 4x4, 64QAM 4x4, 8PSK R=2/3 R=2/3 R=1/2</p><p>1.0E-03 2.0 6.0 10.0 14.0 18.0 22.0 26.0 Es/No (dB) Figure 5. Performance of selected rate sets in Channel Model D.</p><p>Submission page 10 M. Eroz, et al, Hughes Network Systems September 2004 doc.: IEEE 802.11-04-0941-00-000n</p><p>Channel Model E 1.0E+00 4x4, QPSK 3x3, QPSK R=1/2 R=2/3 e</p><p> t 3x3, 16QAM a 1.0E-01</p><p>R R=1/2</p><p> r o r r E</p><p> t e k c</p><p> a 1.0E-02</p><p>P 4x4, 8PSK R=1/2 3x3, 16QAM 4x4, 64QAM R=2/3 R=2/3 1.0E-03 2.0 6.0 10.0 14.0 18.0 22.0 26.0 Es/No (dB) Figure7. Performance of selected rate sets in Channel Model E.</p><p>AWGN, 1x1, 2x2, 3x3, 4x4 1.0E+00</p><p>8PSK,R=1/2 16QAM,R=2/3 QPSK,R=1/2 64QAM,R=2/3 e t</p><p> a 1.0E-01 R</p><p> r o r r E</p><p> t e k c</p><p> a 1.0E-02 P QPSK,R=2/3</p><p>16QAM,R=1/2</p><p>1.0E-03 2.0 4.0 6.0 8.0 10.0 12.0 14.0 16.0 18.0 Es/No (dB) Figure 8. Performance of selected rate sets in AWGN channel, with channel matrices as specified by the disclosure document.</p><p>Submission page 11 M. Eroz, et al, Hughes Network Systems</p>
Details
-
File Typepdf
-
Upload Time-
-
Content LanguagesEnglish
-
Upload UserAnonymous/Not logged-in
-
File Pages11 Page
-
File Size-