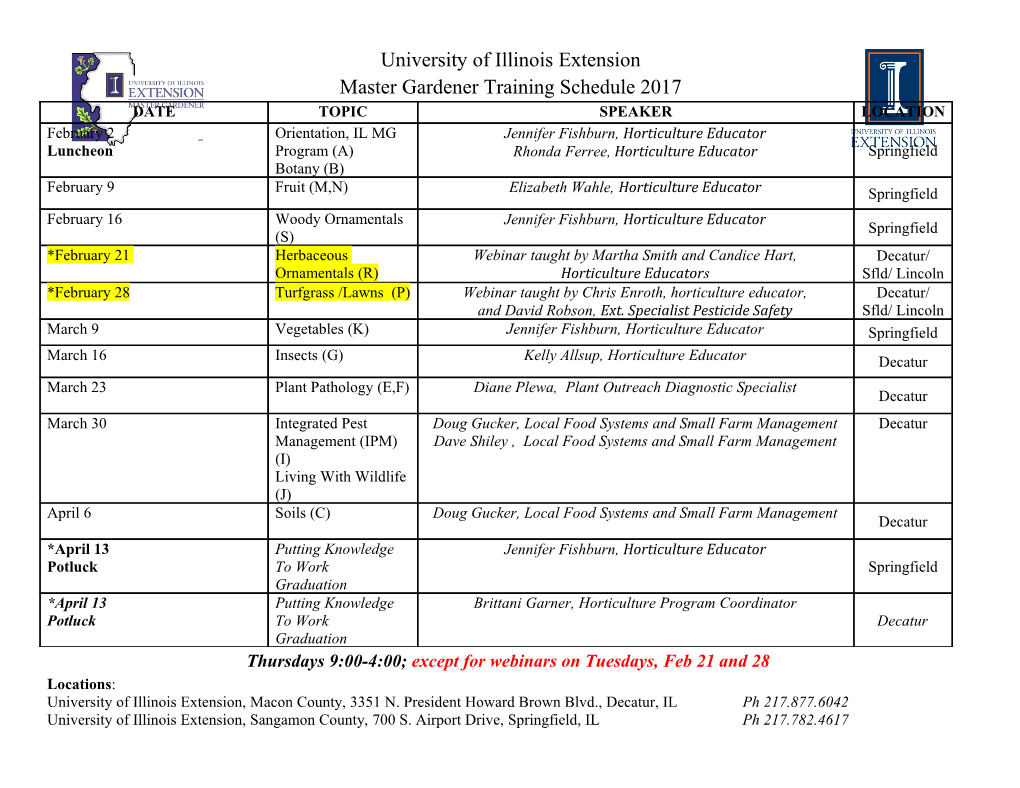
<p>ELECTRONIC SUPPLEMENT TO METHODS Role of Structural Barriers in the Mechanism of Alternans- Induced Reentry</p><p>Joseph M. Pastore PhD, David S. Rosenbaum MD</p><p>The Heart and Vascular Research Center and The Departments of Medicine and Biomedical Engineering, Case Western Reserve University, Cleveland, Ohio</p><p>Short Title: Alternans-Induced Reentry</p><p>Presented in part at the 20th annual scientific sessions of the North American Society of Pacing and Electrophysiology, Toronto, 1999</p><p>Address correspondence to: David S. Rosenbaum, M.D. Director, Heart and Vascular Research Center MetroHealth Campus, Case Western Reserve University Rammelkamp, 6th floor 2500 MetroHealth Drive Cleveland, Ohio 44109-1998</p><p>TEL: (216) 778-7342 FAX: (216) 778-1261 e-mail: [email protected] Pastore et al Alternans Induced Reentry 2</p><p>METHODS</p><p>Experimental Preparation</p><p>Hearts from 26 male retired breeder guinea pigs were rapidly excised and perfused as</p><p>Langendorff preparations with oxygenated (95% O2, 5% CO2) Tyrode’s solution containing (mmol/L) NaCl</p><p>130, NaHCO3 25.0, MgSO4 1.2, KCl 4.75, dextrose 5.0, and CaCl2 1.25 (pH 7.40, 27C). During all experiments, the left ventricular endocardial surface was eliminated using a cryoablation procedure described previously1. This procedure produces a thin (800 m depth) viable rim of left ventricular epicardium having normal electrophysiological properties1 and served to restrict propagation to the surface from which action potentials were recorded. In contrast to earlier studies where the right ventricle was completely (i.e. transmurally) cryoablated, the endocardial surface of RV and LV were ablated similarly so as to allow a thin viable rim of epicardial tissue throughout the mapping field. Hearts were stained with 100 ml of the voltage-sensitive dye di-4-ANEPPS (20 M) by direct coronary perfusion for 10 minutes.</p><p>Beating and perfused hearts were placed in a custom built imaging chamber. To avoid surface cooling and the formation of intracardiac temperature gradients, the heart was immersed in the coronary effluent which was maintained at a constant temperature (equal to the perfusion temperature) with a heat exchanger. Gentle pressure was applied to the posterior surface of the heart with a movable piston to stabilize the anterior surface of the ventricle against an imaging window so as to eliminate motion artifacts without altering action potential properties1,2. Three silver disk electrodes fixed to the chamber in positions corresponding to ECG limb leads I, II, and III were used to measure ECG signals that were filtered (0.05 Hz-1000 Hz), amplified (1000X), and calibrated with a 1 mV square pulse to enable quantitative measurement of T-wave alternans. ECG signals were recorded simultaneously with action potential maps and were displayed on a digital oscilloscope to monitor cardiac rhythm.</p><p>To electrotonically uncouple neighboring regions of cells having known differences in cellular restitution kinetics3, a well-defined insulating SB was produced using an argon ion laser as described previously1 (Figure 1). Laser light (wavelength=488 nm, power=1.1 W) was coupled to a fiberoptic cable Pastore et al Alternans Induced Reentry 3 and focused through a gradient lens so that energy could be applied to the heart through the chamber imaging window without moving the heart. Therefore, action potentials were recorded from identical regions of cells before (i.e. control) and after the introduction of the SB. The laser was positioned initially below the bifurcation of the left anterior descending coronary artery and was moved at a 45 angle</p><p>(perpendicular to orientation of cardiac fibers1) by a computer-driven stepping motor controller to produce a 2x10 mm SB spanning from epicardial surface transmurally to the endocardial cryoablation (Figure 1).</p><p>Following each experiment, preparations were examined under light microscopy after staining with 2,3,5- triphenyl tetrazolium chloride4 to confirm that the epicardial SB fully extended to the zone of endocardial necrosis.</p><p>Optical Mapping System</p><p>Previously, we have developed an optical action potential mapping system that is capable of resolving membrane potential changes as small as 0.5 mV simultaneously from 128 sites across the anterior epicardial surface of the intact guinea pig ventricle (see 1-3 for details). In order to map ventricular action potentials from sites around the entire periphery of the SB, an optical magnification of 1.2X was used which corresponded to a total mapping area of 15 x 15 mm, 1.25 mm inter-pixel spatial resolution, and 1 ms temporal resolution.</p><p>Measurement of Electrical Alternans</p><p>A previously validated experimental model of pacing-induced steady-state T-wave alternans5 was used because, as is the case in patients, electrical alternans in this model persists over time (i.e. alternans are not transient), does not require ischemic damage, and principally involves the T-wave rather than the QRS complex6. Alternans of cellular repolarization time (RT) was determined by measuring differences in local RT (relative to the stimulus artifact) on consecutive beats. RT and activation times</p><p>(AT) were determined from each action potential using previously described algorithms1,3, and all analyses were visually verified by one investigator (JMP). Action potential duration (APD) was defined as the difference between RT and AT. Since, in a series of preliminary studies, we found that the error associated with measurement of RT was 34 ms, only RT alternans greater than 10 ms was considered significant. A previously validated spectral analysis technique5 was used to confirm that beat-to-beat Pastore et al Alternans Induced Reentry 4 fluctuations in the action potential amplitude were occurring exclusively at the alternans frequency (i.e. every other beat) and were stable during each recording.</p><p>To measure alternations of the ECG T-wave, 32 consecutive ECG complexes recorded from each lead were aligned by the stimulus artifact. For every sample point within the ECG complex, a time series was generated from beat-to-beat changes in amplitude of that point, and a power spectrum was calculated using previously validated techniques6,7. The resulting spectra were then averaged over the extent of the T-wave to obtain a single value of alternans (in V) for each lead. </p><p>Measurement of Conduction Velocity and Repolarization Gradients Using Optical Mapping</p><p>An algorithm that was developed previously to calculate the direction and magnitude of conduction velocity vectors from extracellular electrograms 8 was applied in this investigation to optically recorded action potential maps to objectively and accurately quantify to conduction velocity and repolarization gradients. Briefly, following assignment of ATs (or RTs) at each site within the mapping array, the surface created by the ATs (or RTs) was fit to a polynomial. At each site, the local gradient vector of the surface was calculated, the direction of which corresponded to the direction of propagation</p><p>(or gradient of repolarization), and magnitude represented the conduction velocity (or the inverse of the repolarization gradient). </p><p>Stimulation Protocol</p><p>As shown previously in the experimental model used in this study, T-wave alternans can be elicited above a critical heart rate threshold by steady-state pacing and can create an electrophysiological substrate for reentrant VF 5. Therefore, a similar stimulation protocol was performed to obtain alternans and initiate arrhythmias in this investigation. Briefly, the epicardial surface was stimulated at 5X diastolic threshold current using a bipolar needle electrode (1.5 mm inter-electrode spacing) gently inserted into the anterior portion of the left ventricle. Action potentials were recorded simultaneously from 128 sites</p><p>(Figure 1) during steady-state pacing starting at a cycle length (CL) of 400 ms, and then at progressively shorter CLs: 350 ms, 300 ms, 250 ms, and CLs of 220 ms and lower by 10 ms decrements until one-to- one capture was lost or an arrhythmia was initiated. Following loss of one-to-one capture or cessation of the arrhythmia (by pacing or defibrillatory shock), action potentials were again recorded after approximately one minute of pacing at CL=400 ms to ensure that baseline conditions were comparable Pastore et al Alternans Induced Reentry 5 before and after the protocol. To assure consistent and steady-state conditions, all measurements were obtained after 45 sec of pacing at each CL. </p><p>Effect of Structural Barriers on Cellular Electrophysiology</p><p>To ensure that the application of laser energy did not substantially alter the baseline electrophysiology of cells outside of the precisely demarcated SB, validation studies were performed in 15 hearts. During each experiment, each heart served as its own control, and the identical stimulation protocol (described above) was performed before (i.e. control) and after a SB was introduced. The myocardium was stimulated on the anterior surface of the left ventricular free wall near the AV groove</p><p>(Figure 1) to ensure that the sequence of epicardial activation was nearly identical before and after the introduction of the SB. The stimulation protocol was performed only after the heart equilibrated for at least fifteen minutes following both the endocardial cryoablation procedure and the application of the SB.</p><p>Action potential shape and duration, conduction velocity, and ECG morphology were compared at the baseline stimulation CL of 400 ms in the absence and presence of a SB to ensure consistency in baseline cellular electrophysiology throughout the experiment. </p><p>To investigate the effects of a SB on cellular alternans, in a subset of 5 experiments, detailed quantitative analysis of the magnitude and phase of cellular alternans was compared at each steady-state</p><p>CL in the stimulation protocol both before and after the introduction of a SB. Preliminary experiments performed over long time periods (> 3 hrs) without introduction of a SB (i.e. time controls) demonstrated stable electrophysiological properties of this preparation and, importantly, no change in the heart rate dependence or distribution of cellular alternans. Therefore, changes in magnitude or phase of cellular alternans that followed the application of the SB could not be attributed to degradation of the preparation over time.</p><p>Effect of Discordant Alternans on Susceptibility to Arrhythmias</p><p>In the pacing-induced T-wave alternans model, ventricular arrhythmias are consistently initiated in the presence of discordant alternans. To determine the effect of the SB on the resultant arrhythmia induced in the presence of discordant alternans, in 26 hearts, discordant alternans and arrhythmias were initiated in all 26 hearts as described in Stimulation Protocol. In 15 hearts (same 15 as previous section), the stimulation protocol was performed in the absence and presence of a SB; whereas, in 11 hearts, the Pastore et al Alternans Induced Reentry 6 protocol was performed only in the absence of a SB. In all cases, the resultant ventricular arrhythmia was assessed as either VF, SMVT, or no arrhythmia. SMVT was defined as a rapid self-sustaining ventricular rhythm having similar ECG morphology from beat to beat in all leads which persisted for more than 5 seconds. In this model, SMVT typically lasted indefinitely unless actively terminated by pacing1. VF was defined as a rapid polymorphic ventricular rhythm lasting longer than 5 seconds. To ascertain the role of repolarization gradients during discordant alternans in forming an arrhythmogenic substrate, the maximum repolarization gradient within the mapping array was measured at baseline (CL=400) and during discordant alternans at the CL immediately preceding the initiation of either reentrant VF or SMVT. </p><p>Reference </p><p>1. Girouard SD, Pastore JM, Laurita KR, Gregory KW, Rosenbaum DS. Optical mapping in a new</p><p> guinea pig model of ventricular tachycardia reveals mechanisms for multiple wavelengths in a</p><p> single reentrant circuit. Circulation. 1996;93:603-613.</p><p>2. Girouard SD, Laurita KR, Rosenbaum DS. Unique properties of cardiac action potentials recorded</p><p> with voltage-sensitive dyes. J.Cardiovasc.Electrophysiol. 1996;7:1024-1038.</p><p>3. Laurita KR, Girouard SD, Rosenbaum DS. Modulation of ventricular repolarization by a premature</p><p> stimulus: Role of epicardial dispersion of repolarization kinetics demonstrated by optical mapping of</p><p> the intact guinea pig heart. Circ.Res. 1996;79:493-503.</p><p>4. Fishbein MC, Meerbaum S, Rit J. Early phase acute myocardial infarct size quantification:</p><p>Validation of the triphenyl tetrazolium chloride tissue enzyme staining technique. Am.Heart.J.</p><p>1981;101:593-600.</p><p>5. Pastore JM, Girouard SD, Laurita KR, Akar FG, Rosenbaum DS. Mechanism linking T wave</p><p> alternans to the genesis of cardiac fibrillation. Circulation. 1999;99:1385-1394.</p><p>6. Rosenbaum DS, Jackson LE, Smith JM, Garan H, Ruskin JN, Cohen RJ. Electrical alternans and</p><p> vulnerability to ventricular arrhythmias. N.Engl.J.Med. 1994;330:235-241. Pastore et al Alternans Induced Reentry 7</p><p>7. Smith JM, Clancy EA, Valeri R, Ruskin JN, Cohen RJ. Electrical alternans and cardiac electrical</p><p> instability. Circulation. 1988;77:110-121.</p><p>8. Bayly PV, KenKnight BH, Rogers JM, Hillsley RE, Ideker RE, Smith WM. Estimation of conduction</p><p> velocity vector fields from epicardial mapping data. IEEE Trans.Biomed.Eng. 1998;45:563-571.</p>
Details
-
File Typepdf
-
Upload Time-
-
Content LanguagesEnglish
-
Upload UserAnonymous/Not logged-in
-
File Pages7 Page
-
File Size-