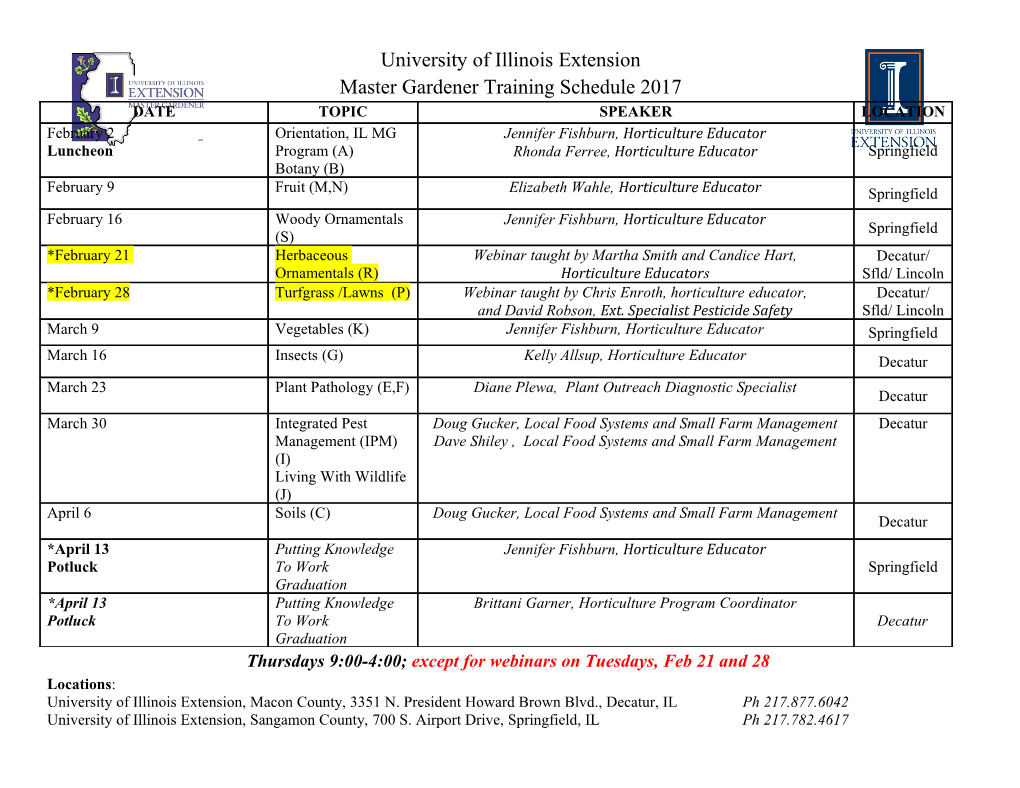
<p>Supplementary Information:</p><p>Ultrasensitive Broadband Phototransistors Based on Perovskite/Organic-</p><p>Semiconductor Vertical Heterojunctions</p><p>Chao Xie,1 Peng You,1Zhike Liu,1 Li Li2 Feng Yan1*</p><p>1 Department of Applied Physics, The Hong Kong Polytechnic University, Hong Kong, China 2 Institute of Textiles & Clothing, The Hong Kong Polytechnic University, Hong Kong, China *Corresponding authors. E-mail:[email protected] </p><p>Content:</p><p>1. Preparation and characterization of CH3NH3PbI3-xClx/PEDOT:PSS phototransistors</p><p>2. Characterization of the hole mobility in PEDOT:PSS</p><p>3. Characterization of current noise of photodetectors</p><p>4. The effect of perovskite thickness on the response speeds of phototransistors</p><p>5. Fabrication and characterization of other phototransistors</p><p>6. Supporting Figures </p><p>7. References</p><p>1. Preparation and characterization of CH3NH3PbI3-xClx/PEDOT:PSS phototransistors</p><p>1 Mechanisms for the improved conductivity of PEDOT:PSS after spin-coating of perovskite. Prior to spin-coating of perovskite, the electrical characteristic of the device (only PEDOT:PSS in the channel) was measured and a typical I-V curve recorded in dark is shown in Figure S6(a). It can be found that the channel current is increased by almost two orders of magnitude from ~0.2 mA to ~22 mA at an applied bias voltage of ± 0.5 V after the spin-coating of perovskite. It is notable that the solvent of the perovskite precursor is N,N-Dimethylformamide (DMF), which plays a key role in improving the conductivity of the PEDOT:PSS layer as explained below.</p><p>In order to clarify the mechanisms for the improved channel current, control experiments were further performed. The device with perovskite/PEDOT:PSS film in the channel was rinsed by DMF solvent to completely remove the perovskite and then its electrical property was measured again. As shown in Figure S6(a), the channel current exhibited ~50% further increase after the removal of perovskite. So the high channel current originates from PEDOT:PSS layer only and the perovskite layer shows little contribution. Actually, the perovskite layer may diffuse into the PEDOT:PSS layer to inhibit carrier transport and slightly decease the channel current. So the removal of the perovskite can increase the current across PEDOT:PSS for a certain value.</p><p>Another control device with only PEDOT:PSS in the channel was spin-coated with DMF solvent and annealed at 100 ºC for 45 min in an glovebox. The electrical characteristics of the device were recorded before and after spin-coating, as shown in Figure S6(b). We can find that the channel current is increased by ~250 times after spin-coating DMF, which shows the effect similar to that induced by the spin-coating of perovskite precursor. These results undoubtedly indicate that the improved conductivity of PEDOT:PSS is due to the effect of DMF coated on the surface.</p><p>The increase of the conductivity of PEDOT:PSS after the treatment of organic solvent has been</p><p>2 reported before1, which is mainly attributed to the component/morphology changes of the</p><p>PEDOT:PSS surface after the treatment of polar solvent (DMF in our case). Thespeculation can be validated by the X-ray photoelectron spectroscopy (XPS) and atomic force microscope (AFM) tests.</p><p>Figure S7 shows the XPS spectra of a pure PEDOT:PSS film and a DMF-treated PEDOT:PSS film.</p><p>The S (2p) peaks observedat the binding energy of 167.7 eV correspond to the sulfur signalfrom</p><p>PSS, and the peaks at the 164.5 eV and 163.4 eV correspond to the sulfur signal from PEDOT 2. The surface ratio of PSS to PEDOT, which can be calculated by using the integral area ratio of the peaks assigned to PSS and PEDOT, decreases from 3.82:1 for the former to 2.87:1 for the latter, indicating a remarkable reduction of PSS by ~ 25% in the PEDOT:PSS film treated by DMF on the surface 3. It is reasonable that partial hygroscopic PSS on PEDOT:PSS surface will be dissolved in polar DMF solvent when the precursor perovskite solution was dropped onto the PEDOT:PSS and subsequently be removed during spin-coating.</p><p>AFM phase images shown in FigureS8 disclose clear structural changes. Pristine PEDOT:PSS before the treatment shows continuous PEDOT and PSS domains with weak phase separation, while</p><p>DMF treated PEDOT:PSS film shows much higher surface roughness, indicating the removal of</p><p>PSS on the surface. This change is consistent with the previously reported work1,3. It is well known that the PEDOT:PSS surface contains more PSS than its bulk4. The removal of the insulator PSS from the film surface is beneficial to the improvement of the film conductivity. Normally, the conductivity of PEDOT:PSS film can be increased for 2-3 orders of magnitude by immersing the film in the polar solvents or dropping polar solvents on the film1,3.Therefore, the dramatic increase of the conductivity of the PEDOT:PSS film is due to the effect of DMF coated on the surface.</p><p>2. Characterization of the hole mobility in PEDOT:PSS</p><p>3 The hole mobility in the PEDOT:PSS cannot be directly measured by a field effect transistor because the DMF-treated PEDOT:PSS may have a much higher carrier mobility on the top surface than on the bottom. We find that the hole mobility (μ) of the DMF-treated PEDOT:PSS used in the perovskite photodetector can be characterized by constructing an organic electrochemical transistor</p><p>(OECT) consisting of DMF treated PEDOT:PSS in the channel as active layer, Ag/AgCl electrode as gate electrode and phosphate-buffered saline (PBS) solution as electrolyte (Inset in Figure S9(a) displays the schematic illustration of the OECT device)5, according to the following equation6:</p><p>I DS W CiVDS (S1) VGS L</p><p>Where IDS is the channel current flowing through the PEDOT:PSS layer, VGS is the applied gate voltage, W and L are the width and length of the channel, respectively (W and L are 0.6 cm and 0.2</p><p> mm, respectively), Ci is the effective gate capacitance per unit area, and VDS is the applied source-</p><p> drain voltage (VDS = 0.05 V). Based on the slope of IDS-VGS curve (Figure S9(a)) at VGS = 0 V and VDS</p><p>-3 -1 = 0.05 V, =1.006 ×10 AV is obtained. The Ci can be estimated by employing electrochemical impedance spectroscopy (EIS) measurement in the PBS solution (Inset in Figure S9(b) shows the schematic illustration of the EIS test). In this test, the PEDOT:PSS film served as working electrode, while Pt and Ag/AgCl electrodes were used as the counter electrode and reference</p><p>-2 electrode, respectively. As shown in Figure S9(b), the Ci is ~ 118.88 μFcm at the working frequency of 0.1 Hz, which is at the same frequency of the OECT test. Then, according to equation</p><p>(1), the hole mobility (μ) of the DMF-treated PEDOT:PSS is estimated to be ~ 5.643 cm2/Vs.</p><p>3. Characterization of current noise of the photodetectors</p><p>In this study, a low noise current preamplifier (Stanford Research System, Model SR570) connected</p><p>4 with an oscilloscope (Tektronix, TDS 2012C) was employed to analysis the noise in the dark current of the perovskite photodetector. The channel current of the photodetector was converted into voltage signal through the low noise current preamplifier (1 mA vs. 1 V here) and directly detected by the oscilloscope. This current can be offset by carefully setting the input offset of the preamplifier. The filter of the preamplifier was set to ‘LOWPASS’ mode with frequency at 1000 Hz in order to filter the ambient high frequency noisein this measurement. The offset dark current acquired at sampling rate of 50 Hz is shown in Figure S10 (a). The noise spectral density was obtained by doing a Fourier transform (FFT) of the measured dark current, as shown in Figure S10</p><p>(b), according to a method used in a previous work.7 Careful analysis of the data indicates that the noise spectral density is ~60 nAHz-1/2 at the bandwidth of 1 Hz, which sets the lower threshold limit of noise equivalent power (NEP) at 3.14×10-17 W. Therefore, the highest specific detectivity (D*) of the photodetector (~598 nm) is ~1.4×1014 Jones at the applied voltage of 0.5 V.</p><p>4. The effect of perovskite thickness on the response speeds of phototransistors</p><p>In our phototransistors, the response speeds might be influenced by the thickness of perovskite layer. We studied time-dependent photoresponse of two perovskite/PEDOT:PSS phototransistors on</p><p>SiO2/Si substrates with the thickness of ~180 nm and ~300 nm, respectively. We found that the device with ~180 nm-thick perovskite layer exhibited faster response than its counterpart with ~300 nm-thick perovskite (Figure S12).</p><p>5. Fabrication and characterization of other phototransistors</p><p>CH3NH3PbI3/PEDOT:PSS: The PEDOT:PSS film was deposited onto a cleaned SiO2/Si substrate with pre-defined Cr (10 nm)/Au (100 nm) electrodes (channel: W/L = 1000 µm/2 µm) by spin- coating PEDOT:PSS aqueous solution (Clevios™ PH 500) at 4000 rpm for 40 s, followed by</p><p>5 annealing at 150 ºC for 1 h in a N2-filled glovebox. Then the CH3NH3PbI3 perovskite was formed by</p><p> spin-coating a mixed halide perovskite precursor (CH3NH3I and PbI2 dissolved in a mixed solvent of DMF and Dimethyl sulfoxide (DMSO) (7:3 volume ratio) at molar ratio of 3:1) onto the substrate at 4000 rpm for 1 min and followed by annealing at 90 ºC for 45 min.</p><p>The photoresponse of the device is shown in Figure S13. The solution processed CH3NH3PbI3</p><p> film shows much shorter carrier lifetime in comparison with CH3NH3PbI3-xClx. So the density of the accumulated photo-generated electrons in the perovskite film is not very high and the photogating</p><p> effect is not as strong as that in a CH3NH3PbI3-xClx/PEDOT:PSS phototransistor. In Figure S12 (b- d), we can find the persistent photocurrent (PPC) of the device after switching off the light presumably due to the capture of charges in deep trap states. These deep trap states may increase</p><p> electron-hole recombination rate in the CH3NH3PbI3 film, which further confirm the short carrier lifetime in the perovskite film.</p><p>CH3NH3PbI3-xClx/P3HT: P3HT film was deposited onto a cleaned SiO2/Si substrate modified with hexamethyldisilazane (HMDS) by spin-coating P3HT solution (5 mg/ml in CB) at 2500 rpm for 1</p><p> min in a N2-filled glovebox, followed by annealing at 120 ºC for 1 h. After O2 plasma treatment on</p><p>P3HT for 90 s at low power mode, a mixed halide precursor solution was spin-coated onto the</p><p>P3HT film at 2500 rpm for 1 min and annealed at 100 ºC for 45 min to form CH 3NH3PbI3-xClx perovskite film. After that, Au electrodes were deposited on top of the perovskite film through a shadow mask by thermal evaporation and formed a channel of width/length =2 mm/0.1 mm.</p><p>The photoresponse of the device is shown in Figure S14 and Figure S15. The carrier mobility</p><p>-2 2 of the P3HT is only ~2.7×10 cm /Vs. So the responsivity of the device is very low even when VDS</p><p>=5 V.</p><p>6 6. Supporting Figures</p><p>Figure S1 Typical (a) plane view and (b) cross-sectional view SEM images of CH3NH3PbI3-xClx perovskite/PEDOT:PSS films on a SiO2/Si substrate.</p><p>Figure S2 Transfer characteristics of a CH3NH3PbI3-xClx perovskite field-effect transistor on a SiO2/n+Si substrate measured in dark. The transistor channel width/length = 1000 µm/2 µm. The thickness of the SiO2 layer is 300 nm. </p><p>7 Figure S3 Photocurrent of the CH3NH3PbI3-xClx/PEDOT:PSS photodetector as a function of applied voltage characterized under (a) 370 nm and (b) 598 nm light with different light intensities, respectively. Responsivity of the photodetector as a function of applied voltage characterized under (c) 370 nm and (d) 598 nm light with different light intensities, respectively.</p><p>Figure S4 (a) I-V curves of a CH3NH3PbI3-xClx perovskite film in dark and under light illumination with the wavelength of 598 nm. (b) Typical plane view SEM images of a CH3NH3PbI3-xClx perovskite film on a SiO2/Si substrate.</p><p>8 Figure S5 (a) I-V curves of a control device based on pure PEDOT:PSS film characterized in dark and under light with wavelengths of 370 nm, 598 nm and 895 nm, respectively. (b)-(d) Time-dependent photoresponse of the</p><p>PEDOT:PSS film to periodical on/off illuminations with wavelength of 370 nm, 598 nm and 895 nm, respectively.</p><p>Figure S6 (a) I-V curves of the PEDOT:PSS film, CH3NH3PbI3-xClx perovskite/PEDOT:PSS films before and after rinsing perovskite with DMF in dark, respectively. (b) I-V curves of the PEDOT:PSS film before and after treatment with DMF in dark, respectively.</p><p>9 Figure S7 XPS spectra of the pure PEDOT:PSS film and PEDOT:PSS film treated by coating DMF, indicating the reduction of PSS on PEDOT:PSS surface after the DMF treatment. </p><p>Figure S8 AFM phase images of (a) the pure PEDOT:PSS film and (b) the DMF treated PEDOT:PSS film. </p><p>10 Figure S9 (a) The transfer characteristics of the PEDOT:PSS based OECT measured in PBS solution. VDS=0.05 V.</p><p>Inset shows the schematic illustration of the PEDOT:PSS based OECT. (b) The Ci as a function of the working frequency. Inset displays the schematic illustration of the EIS measurement.</p><p>Figure S10 (a) The noise of the dark current of a perovskite/PEDOT:PSS phototransistor under the bias voltage of</p><p>0.5 V. (b) Analysis of noise spectral density of the perovskite photodetector based on the Fourier transform of the dark current noise.</p><p>Figure S11 (a) Typical current density-voltage (J-V) curves of aCH3NH3PbI3-xClx perovskite solar cell with power</p><p>11 conversion efficiency (PCE) of ~13%. Inset shows the schematic illustration of the solar cell. (b) EQE spectrum of the solar cell, indicating no photocurrent contribution at wavelength larger than 800 nm.[11]</p><p>Figure S12 Time-dependent photoresponse of two perovskite/PEDOT:PSS phototransistors on SiO2/Si substrates with different perovskite thicknesses under 895 nm periodical on/off illumination.</p><p>Figure S13 (a) Photocurrent of a CH3NH3PbI3 perovskite/PEDOT:PSS photodetector under the light illumination</p><p>12 with wavelengths of 370 nm, 598 nm and 895 nm, respectively. (b)-(d) Time-dependent photoresponse of the device to periodical on/off illuminations with wavelength of 370 nm, 598 nm and 895 nm, respectively.</p><p>Figure S14 (a) Transfer curves of a CH3NH3PbI3-xClx/P3HT phototransistor measured in dark and under light illumination with wavelengths of 370 nm, 598 nm and 895 nm, respectively. The hole mobility of P3HT in dark is</p><p>2.7×10-2 cm2/Vs. (b)-(d) Time-dependent photoresponse of the phototransistor to periodical on/off light with wavelengths of 370 nm, 598 nm and 895 nm, respectively. (e) Time-dependent photoresponse of another</p><p>CH3NH3PbI3-xClx/P3HT phototransistor to 598 nm periodical on/off light, indicating good long-term stability of the device.</p><p>13 Figure S15 Light intensity-dependent responsivities of a perovskite/P3HT phototransistor under the light illumination with the wavelength of (a) 598 nm and (b) 895 nm. </p><p>Figure S16 Bend bending of the heterojunction (a) perovskite/PEDOT:PSS and (b) perovskite/P3HT heterojunctions under light illumination. </p><p>14 Figure S17 XRD spectra of CH3NH3PbI3-xClx perovskite/PEDOT:PSS on SiO2/Si and PI substrates. Inset shows the normalized main peaks of the spectra.</p><p>15 7. References</p><p>1 Kim YH, Sachse C, MacHala ML, May C, Müller-Meskamp L, Leo K. Highly conductive PEDOT:PSS electrode with optimized solvent and thermal post-treatment for ITO-free organic solar cells. Adv Funct Mater 2011; 21: 1076– 1081. 2 Crispin X, Jakobsson FLE, Crispin A, Grim PCM, Andersson P, Volodina et al. The origin of the high conductivity of poly(3,4-ethylenedioxythiophene)-poly(styrenesulfonate) (PEDOT- PSS) plastic electrodes. Chem Mater 2006; 18: 4354–4360. 3 Alemu D, Wei H-Y, Ho K-C, Chu C-W. Highly conductive PEDOT:PSS electrode by simple film treatment with methanol for ITO-free polymer solar cells. Energy Environ Sci 2012; 5: 9662. 4 Na S-I, Wang G, Kim S-S, Kim T-W, Oh S-H, Yu B-K et al. Evolution of nanomorphology and anisotropic conductivity in solvent-modified PEDOT:PSS films for polymeric anodes of polymer solar cells. J Mater Chem 2009; 19: 9045. 5 Xia Y, Cho JH, Lee J, Ruden PP, Frisbie CD. Comparison of the mobility-carrier density relation in polymer and single-crystal organic transistors employing vacuum and liquid gate dielectrics. Adv Mater 2009; 21: 2174–2179. 6 Horowitz G. Organic Field-Effect Transistors. Adv Mater 1998; 10: 365–377. 7 Liu C-H, Chang Y-C, Norris TB, Zhong Z. Graphene photodetectors with ultra-broadband and high responsivity at room temperature. Nat Nanotechnol 2014; 9: 273–278.</p><p>16</p>
Details
-
File Typepdf
-
Upload Time-
-
Content LanguagesEnglish
-
Upload UserAnonymous/Not logged-in
-
File Pages16 Page
-
File Size-