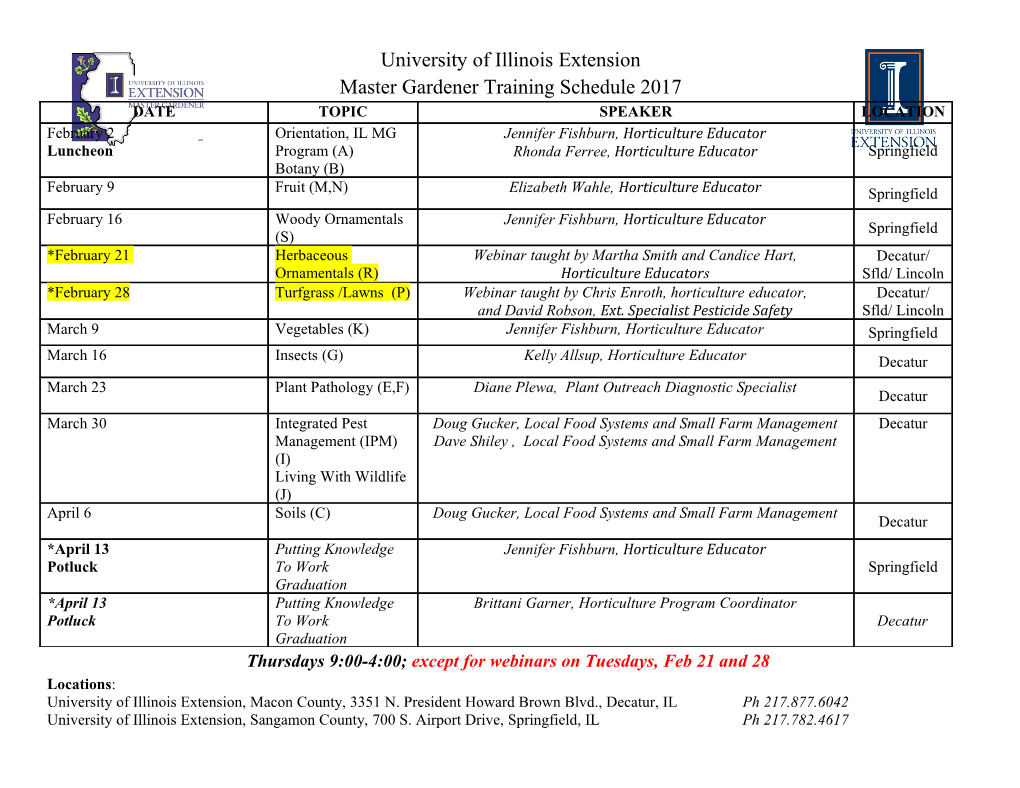
<p> 1</p><p>2</p><p>3</p><p>4</p><p>5</p><p>6</p><p>7 Comparative allelopathic effects of three invasive species (Alliaria petiolata,</p><p>8 Lonicera maackii and Ranunculus ficaria) in the Midwestern United States:</p><p>9 Variation in response with experimental venue and target species</p><p>10</p><p>11 Kendra Cipollini, Kyle Titus, and Crystal Wagner</p><p>12</p><p>13 Kendra Cipollini1, Kyle Titus2, and Crystal Wagner3. Wilmington College, 1870</p><p>14 Quaker Way, Wilmington, OH 45177, USA</p><p>15 1Corresponding author (e-mail: [email protected]). </p><p>16 2Current address: (e-mail: [email protected])</p><p>17 3Current address: (e-mail: [email protected])</p><p>18</p><p>19 Phone: 937-382-6661 x367 (w), 937-532-6128 (cell)</p><p>20 FAX: 937-383-8530</p><p>1 1 21Abstract: Garlic mustard (Alliaria petiolata), Amur honeysuckle (Lonicera maackii) </p><p>22and lesser celandine (Ranunculus ficaria) are three species that invade Midwestern </p><p>23forests in the US and exhibit allelopathy. There is little known about their comparative </p><p>24allelopathic effects on multiple test species. In three experiments, the comparative </p><p>25allelopathy of these species was investigated by making leaf extracts of each species and </p><p>26measuring their effects on test species. In potting soil, there were fewer siliques of A. </p><p>27thaliana when plants were treated with extracts of L. maackii compared to plants treated </p><p>28with no extract and with A. petiolata extracts. In field soil, there were significantly fewer</p><p>29siliques in A. thaliana treated with extracts of L. maackii and R. ficaria compared to with </p><p>30extracts of A. petiolata. The effect of these treatment solutions on germination of three </p><p>31species (Broccoli - Brassica oleracea, Lettuce - Lactuca sativa, and Basil - Ocimum </p><p>32basilicum) was studied. Across all test species, R. ficaria and L. maackii extracts affected</p><p>33germination the least, while A. petiolata extracts affected germination the most. </p><p>34However, the extracts impacted species differentially; L. sativa and O. basilicum were </p><p>35more sensitive to A. petiolata and R. ficaria extracts and B. oleracea was more sensitive </p><p>36to L. maackii extracts. These results provide evidence of differential allelopathic effects </p><p>37of three invasive species, as well as the importance of experimental venue and test </p><p>38species.</p><p>39</p><p>40Key words: allelopathy, exotic species, germination inhibition, leaf extracts, </p><p>41phytotoxicity</p><p>2 2 42Introduction</p><p>43 Invasive species pose a threat worldwide, negatively impacting biodiversity </p><p>44(Wilcove et al. 1998, McGeoch et al. 2010) and exerting significant economic costs </p><p>45(Pimentel et al. 2005). One focus in invasive species ecology is to determine factors that </p><p>46contribute to the success of invasive species (Sakai et al. 2001, Levine et al. 2003). </p><p>47These factors can range from life history traits (Kolar and Lodge 2001) to release from </p><p>48natural enemies (Keane and Crawley 2002). One hypothesis to explain invasive species </p><p>49success is the novel weapons hypothesis (Bais et al. 2003), whereby an invading species </p><p>50possesses a trait novel to the invaded ecosystem. The invasive species can then take </p><p>51advantage of this trait in its new ecosystem during interactions with native species that </p><p>52are evolutionarily-naïve to the trait. In plants, allelopathy can represent a novel weapon </p><p>53(Hierro and Callaway 2003, Callaway and Ridenour 2004). Allelopathy is simply the </p><p>54release of a chemical from the roots or leaves that affects germination, growth and/or </p><p>55reproduction of surrounding species (Rice 1974). Plants that exude these chemicals may </p><p>56be more likely to dominate in a new environment because their neighbors in their native </p><p>57range have evolved resistance or tolerance to their allelochemicals, while neighbors in </p><p>58their invaded range have not (Callaway and Aschehoug 2000). Allelopathy can have </p><p>59direct plant-to-plant effects, whereby allelochemicals directly impact another species </p><p>60(Dorning and Cipollini 2006). Alternatively, allelopathy may have indirect effects on </p><p>61other plants, such as through changing soil ecology or mutualisms (Stinson et al., 2006; </p><p>62Callaway et al. 2008, Zhang et al. 2009). Allelopathic effects may vary depending on </p><p>63target species (Cipollini et al. 2008a) or may vary by conditions such as life stage (Barto </p><p>64et al. 2010a) and nutrients (Cipollini et al. 2008a). </p><p>3 3 65 Allelopathy can be studied in a variety of experimental ways (Inderjit and </p><p>66Callaway 2003), with varying degrees of realism and control. Experiments with the </p><p>67greatest amount of experimental control, yet lowest amount of realism, are simple </p><p>68germination and growth experiments involving the application of specific chemicals or </p><p>69plant extracts with putative allelochemicals, usually in Petri dishes with a paper substrate </p><p>70(e.g., Dorning and Cipollini 2006, Cipollini et al. 2008b, McEwan et al. 2010). Other </p><p>71studies seek to increase the degree of realism at the cost of experimental control in </p><p>72greenhouse studies and field experiments, many times with the use of activated carbon as </p><p>73a manipulative tool (Ridenour and Callaway 2001, Cipollini et al. 2008, Cipollini and </p><p>74Schradin 2011). Field experiments show the greatest amount of realism and ecological </p><p>75relevance yet can be difficult in teasing out exact mechanism due to low amounts of </p><p>76experimental control. Generally, studies of allelopathy start with simple, controlled </p><p>77laboratory experiments before scaling up to field experiments.</p><p>78 Three important invasive species in forests and riparian areas in the Midwestern </p><p>79United States that have evidence of allelopathy are garlic mustard (Alliaria petiolata </p><p>80(Bieb.) Cavara & Grand – Brassicaceae), Amur honeysuckle (Lonicera maackii (Rupr.) </p><p>81Maxim - Caprifoliaceae) and lesser celandine (Ranunculus ficaria L. - Ranunculaceae). </p><p>82Lonicera maackii, native to Asia, in found in the eastern half of the United States (USDA</p><p>832011) and negatively affects trees and understory plants (Gould and Gorchov 2000, </p><p>84Collier et al. 2002, Hartman and McCarthy 2004). Leaf extracts of L. maackii inhibit </p><p>85germination of several test species in the laboratory (Dorning and Cipollini 2006, </p><p>86Cipollini et al. 2008b) and affect growth of Arabidopsis thaliana in the greenhouse </p><p>87(Cipollini et al. 2008a). Field soils collected from areas infested with L. maackii </p><p>4 4 88negatively impacted growth of A. thaliana (Cipollini and Dorning 2008). Cipollini et al. </p><p>89(2008) were unable to demonstrate any allelopathic effects of L. maackii on Impatiens </p><p>90capensis in the field with the use of activated carbon, though sample size issues limited </p><p>91the conclusions of the study. </p><p>92 Alliaria petiolata, native to Europe, is found from coast-to-coast in the United </p><p>93States in nearly all but the southern-most states (USDA 2011). Alliaria petiolata </p><p>94negatively affects understory plants (McCarthy 1997, Meekins and McCarthy 1999, </p><p>95Carlson and Gorchov 2004). Alliaria petiolata has been shown to exhibit allelopathic </p><p>96effects on germination of Geum species (Prati and Bossdorf 2004), though at least one </p><p>97study has shown negligible effects (McCarthy and Hanson 1998) Garlic mustard has </p><p>98several candidate compounds that may be responsible for allelopathic effects (Vaughn </p><p>99and Berhow 1999, Cipollini et al. 2005, Cipollini and Gruner 2007), though exact </p><p>100compounds responsible have not been identified (Barto and Cipollini 2009). Indirect </p><p>101allelopathic effects mediated through mychorrhizae have been demonstrated in the </p><p>102greenhouse, (Stinson et al. 2008, Callaway et al. 2008), though the effect may vary with </p><p>103species or life stage (Barto et al. 2010a). Allelopathic effects of A. petiolata have been </p><p>104shown in the field (Cipollini et al. 2008a). </p><p>105 Ranunculus ficaria, native to Europe, is found in the Northeast, Midwest, and </p><p>106Pacific Northwest regions of the United States (USDA 2011). Ranunculus ficaria is </p><p>107considered an invasive species (Axtell et al. 2010), though there is only one published </p><p>108information confirming its negative impact except (Cipollini and Schradin 2011). </p><p>109Because R. ficaria has purported medicinal effects (Chevallier 1996), it likely exhibits </p><p>110allelopathy (Ehrenfeld 2006). Indeed, the allelopathic effects of R. ficaria on </p><p>5 5 111reproduction of I. capensis were demonstrated in the field (Cipollini and Schradin 2011), </p><p>112but clearly more information is necessary to fully evaluate the impact of R. ficaria as an </p><p>113invasive species, let alone the mechanism for its success.</p><p>114 While there is some evidence of allelopathy for all of these species, there is no </p><p>115research that investigates their comparative allelopathic effects. Other studies have taken</p><p>116a comparative approach to studying allelopathy and allelochemicals, either comparing a </p><p>117suite of invasive species (Pisula and Meiners 2010) or comparing an invasive species to </p><p>118co-occurring similar native species (Barto et al. 2010b, McEwan et al. 2010). Because </p><p>119allelopathic effects can vary with the species on which they are tested (Cipollini et al. </p><p>1202008a, McEwan et al. 2010), we tested multiple species to have more generalizable </p><p>121results. A comparative approach using more than one test and invasive species would be </p><p>122useful in prioritizing restoration activities and possible use of mitigation treatments such </p><p>123as activated carbon (Kulmatiski and Beard 2006), particularly in areas invaded by more </p><p>124than one species. The purpose of our research was to compare allelopathic effects of the </p><p>125leaves of the three invasive species - A. petiolata, L. maackii and R. ficaria - on </p><p>126germination, growth, and/or reproduction of other test plant species, using three different </p><p>127leaf extract concentrations. We predicted that L. maackii would overall be the most </p><p>128allelopathic of the invasive species (e.g., Dorning and Cipollini, 2006, Cipollini et al. </p><p>1292008a), followed by A. petiolata (e.g., McCarthy and Hanson 1998) and R. ficaria. We </p><p>130predicted that A. petiolata would have little to no impact on other species in the </p><p>131Brassicaceae, but have impacts on species in other plant families (e.g., Cipollini et al. </p><p>1322008a). We also predicted that negative effects would increase with concentration of leaf</p><p>133extract.</p><p>6 6 134 Methods</p><p>135Extract Preparation</p><p>136 During the spring, leaf extracts were made from locally-collected leaves of L. </p><p>137maackii, A. petiolata and R. ficaria. Leaves were soaked for 48 hours in distilled water </p><p>138and then filtered. The extracts were then diluted to three different concentrations: 0.1, </p><p>1390.2, and 0.3 g fresh leaf tissue/mL distilled water. The two low concentrations used were </p><p>140similar to previous studies (Dorning and Cipollini 2006, Cipollini et al. 2008a). An </p><p>141additional higher concentration (0.3 g leaf/mL) was used in our current studies. While </p><p>142we have no information about natural concentrations of allelochemicals in the field, this </p><p>143high concentration represents approximately 30% of a mature L. maackii leaf in 1 mL of </p><p>144water (Dorning and Cipollini 2006), which is likely within field levels. Extracts were </p><p>145stored in the freezer until the start of an experiment and stored at 4ºC for the duration of </p><p>146the experiments. For all experiments, we used the fully factorial treatment combinations </p><p>147of extract type or species (A. petiolata, L. maackii or R. ficaria) and extract concentration</p><p>148(0.1, 0.2 or 0.3 g leaf/mL), for a total of 9 extract treatment combinations (3 species x 3 </p><p>149extract concentrations = 9 experimental treatment combinations).</p><p>150</p><p>151Germination and reproduction of Arabidopsis in potting soil</p><p>152 In May of 2008, we planted 10 seeds of Arabidopsis thaliana into 100 mL pots </p><p>153containing potting soil (Pro-Mix BX, Premier Horticulture, Inc., Quakertown, PA) and </p><p>1541mL of slow release fertilizer (Osmocote, The Scotts Company, Marysville, OH). </p><p>155Arabidopsis thaliana was chosen as a target species due to its sensitivity to </p><p>156allelochemicals (Pennacchio et al. 2005) and its successful use in previous allelopathy </p><p>7 7 157studies (Cipollini et al. 2008a, Cipollini and Dorning 2008). Four replicates were used </p><p>158for each treatment combination (3 species x 3 concentrations x 4 replicates = 36 </p><p>159experimental units). Additionally, there were also four replicate controls that received </p><p>160distilled water as a treatment, for a total of 40 pots in the experiment. Pots with seeds </p><p>161were immediately treated with 10mL of their specified extract (or control). The number </p><p>162of germinated plants in each pot was recorded every day for 2 weeks, at which time </p><p>163plants were thinned to one plant per pot. No plants germinated after 7 days. Each pot </p><p>164was treated with 10mL of extract every other week and water was given to the plants as </p><p>165needed. We performed the experiment in an air-conditioned growth room equipped with </p><p>166grow lights with high output fluorescent lights. Light levels were ~50 μmol/m2·s PAR </p><p>167and set on a timer for 15 h days and 9 h nights. We measured date of first flowering. </p><p>168After 13 weeks, we counted the number of siliques per plant and we collected 10 </p><p>169randomly selected siliques from each plant to assess seed mass per silique. Two plants </p><p>170died during the experiment and were therefore not included in the analysis of final </p><p>171measurements.</p><p>172 For the effect of extract concentration on germination over 7 days, we performed </p><p>173a Multivariate Analysis of Variance (MANOVA) for each species, using the number </p><p>174germinated as a separate variable in the model (Von Ende 1993). When significance was </p><p>175found in the MANOVA using Wilk’s λ, we ran separate univariate Analyses of Variance </p><p>176(ANOVAs) for each date, followed by Tukey’s test to determine significant differences </p><p>177between treatments. For the final response variables, due to constraints of the design we </p><p>178were unable to perform fully-crossed two-way ANOVAs for the two factors of species </p><p>179and extract concentration with the control treatments in the model. We first performed a </p><p>8 8 180series of three two-way ANOVAs with the factors of species and concentration and their </p><p>181interaction on the response variables of days to flowering, silique number and seed mass. </p><p>182There was a significant effect of species for the response variables of silique number and </p><p>183days to flowering (F2,25 = 3.98, p = 0.031 and F2,25 = 3.42, p = 0.049, respectively). There </p><p>184were no significant differences for the factor of concentration or the interaction between </p><p>185concentration and species for any response variable. </p><p>186 One major objective of this study was to statistically compare differences between</p><p>187species and the control. Since the effect of concentration was not significant for any </p><p>188response variable, we made a post hoc decision to remove the factor of concentration </p><p>189from the model. We then performed a MANOVA with the response variables of days to </p><p>190flowering, silique number and seed mass with the factor of extract type, either control or </p><p>191one of the three invasive species. When significance was found in the ANOVA using </p><p>192Wilk’s λ, we ran separate univariate ANOVAs for each response variable, followed by </p><p>193Tukey’s test to determine significance between means. We set α at 0.05 for all tests and </p><p>194used Type III sums of squares in this unbalanced design. Minitab was used for all </p><p>195statistical analyses (Ryan et al. 2005).</p><p>196</p><p>197Reproduction of Arabidopsis in field soil</p><p>198 In August of 2009, we planted Arabidopsis thaliana (L.) Heynh (Brassicaceae) </p><p>199into 100 mL pots containing field soil, locally-collected in a woodlot area free of invasive</p><p>200species. Four replicates were used for each treatment combination (3 species x 3 </p><p>201concentrations x 4 replicates = 36 experimental units). Because we had found with </p><p>202previous treatments that A. petiolata extracts served as a negative control for A. thaliana </p><p>9 9 203(see results above and Cipollini et al. 2008a) and because of issues with data analysis, </p><p>204using a control with our design, we did not use a control of no extract for this study. Pots</p><p>205with seeds were immediately treated with 10mL of their specified extract. Plants were </p><p>206thinned to one plant per pot one week later. Each pot was treated with 10mL of extract </p><p>207every two weeks and water was given to the plants as needed. Ten mL of 0.4g/L </p><p>208fertilizer (Peters 20-20-20 N-P-K plus micronutrients; Grace-Sierra, Milpitas, CA) </p><p>209dissolved in distilled water were added approximately every other week. We performed </p><p>210the experiment in an air-conditioned growth room equipped with grow lights with high </p><p>211output fluorescent lights. Light levels were ~50 μmol μmol/m2·s PAR and set on a timer </p><p>212for 15 h days and 9 h nights. After 10 weeks, we counted the number of siliques per </p><p>213plant. We performed an ANOVA with the response variable of silique number with the </p><p>214fully-crossed factors of species and extract concentration, followed by Tukey’s test to </p><p>215determine significance between means. We set α at 0.05 for all tests.</p><p>216</p><p>217Germination of test species on paper</p><p>218 The allelopathic potential on germination removing any soil effects was further </p><p>219explored by applying extracts to three agricultural species in three separate plant families:</p><p>220Brassica oleracea ‘Copenhagen Early Market' (Brassicaceae), Lactuca sativa ‘Grand </p><p>221Rapids, Tipburn Resistant’(Asteraceae) and Ocimum basilicum (Laminaceae). We chose </p><p>222these species since they were readily available, germinate easily and represent different </p><p>223plant families. Additionally, agricultural species such as lettuce and radish are frequently</p><p>224used in allelopathy studies (McCarthy and Hanson 1998, Pisula and Meiners 2010). Four</p><p>225replicates were used for each treatment combination (3 extract species x 3 concentrations </p><p>10 10 226x 3 test species x 4 replicates = 108 experimental units). Additionally, there were also </p><p>227four replicate controls per test species that received distilled water as a treatment, for a </p><p>228total of 120 experimental units in the experiment. Ten seeds of each appropriate species </p><p>229were placed on folded paper towels, which were watered with 10 ml of extract solution </p><p>230(or control). Paper towels were placed in plastic sandwich bags and placed under </p><p>231fluorescent lights with a daylength of 14 hours. Germination (measured as emergence of </p><p>232the radicle) was followed for 28 days. No additional seeds germinated after 14 days.</p><p>233 We analyzed the number germinated after 14 days using a fully-crossed three-way</p><p>234ANOVA with the factors of extract type (A. petiolata, L. maackii or R. ficaria), extract </p><p>235concentration (0.1, 0.2 or 0.3 g/mL) and test species (B. oleracea, L. sativa or O. </p><p>236basilicum). Data were transformed prior to analysis to meet model assumptions. We </p><p>237used Tukey’s test to determine significance between means. We set α at 0.05 for all tests.</p><p>238Because we could not use our control treatments directly in our full model and because </p><p>239we want to determine which extracts actually inhibit germination compared to the </p><p>240control, we performed a series of nine one-way ANOVAs for each test species and for </p><p>241each extract species separately with the factor of concentration (0, 0.1, 0.2 or 0.3 g </p><p>242leaf/mL) as the source of variation.</p><p>243</p><p>244Results</p><p>245Germination and reproduction of Arabidopsis in potting soil</p><p>246 For the germination over 7 days, there was a significant difference for L. maackii </p><p>247in the MANOVA (F21, 17 = 3.398, p = 0.007). In the univariate ANOVA, there was </p><p>248significant delay in germination for the first two days of the time course (F3,12 = 9.13, p = </p><p>11 11 2490.002 and F3,12 = 13.80, p < 0.001). For the first day of germination, there were less </p><p>250seeds germinated in all extract treatments compared to the control (Fig. 1). For the </p><p>251second day of germination, there were less seeds germinated in the 0.2 g/mL and 0.3 </p><p>252g/mL concentrations compared to the control and the 0.1 g/mL concentration (Fig. 1). </p><p>253For the final response variables, there was a significant effect of extract type in the </p><p>254MANOVA (F9,78 = 2.038, p = 0.046). In the ANOVA, there was significant effect of </p><p>255extract type for silique number (F3,34 = 2.89, p = 0.049) and a near significant effect of </p><p>256extract type for flowering (F3,34 = 2.56, p = 0.071). There were significantly less siliques </p><p>257in the L. maackii extract treatment compared to the control and A. petiolata extract </p><p>258treatments, with the R. ficaria extract treatment intermediate between the two groups </p><p>259(Fig. 2). Because the effect of species on days to flowering was significant in the first </p><p>260full ANOVA model, we present here the means for each extract treatment to investigate </p><p>261the nature of the effect (Fig. 3). Flowering in plants treated with R. ficaria extracts were </p><p>262slightly delayed compared to A. petiolata extract treatments at p = 0.10.</p><p>263</p><p>264Reproduction of Arabidopsis in field soil</p><p>265 In the ANOVA, there was a significant effect of extract type on silique number </p><p>266(F2, 27 = 3.55, p = 0.043) and a near-significant effect of extract concentration on silique </p><p>267number (F2, 27 = 3.31, p = 0.052). There were more siliques produced by plants treated </p><p>268with the A. petiolata extracts compared to plants treated with the L. maackii or R. ficaria </p><p>269extracts (Fig. 4).</p><p>270</p><p>12 12 271Germination of test species on paper</p><p>272 All of the seeds of L. sativa and B. oleracea germinated in each of the four control</p><p>273replicates. In the control for O. basilicum, nearly all germinated (mean ± SE = 9.3 ± 0.5).</p><p>274In the ANOVA, there was a significant effect of test species, extract species and extract </p><p>275concentration on germination (Table 1). Across all other treatments, B. oleracea (8.4 ± </p><p>2760.4) and L. sativa (7.6 ± 0.4) and had higher germination than O. basilicum (6.0 ± 0.5). </p><p>277Across all other treatments, there was significantly lower germination in A. petiolata </p><p>278extract treatments (6.3 ± 0.5) compared to L. maackii and R. ficaria extract treatments </p><p>279(8.1 ± 0.3 and 7.6 ± 0.5, respectively). Across all other treatments, with each increase in </p><p>280concentration, there was a decrease in germination (9.1 ± 0.3, 7.5 ± 0.5, 5.5 ± 0.5 for 0.1 </p><p>281g/mL, 0.2 g/mL and 0.1 g/mL, respectively). There was a significant effect of the </p><p>282interaction of extract species with test species and with extract concentration (Table 1). </p><p>283The effect of extract species varied with test species, with A. petiolata extracts having the</p><p>284strongest effects on germination of L. sativa and O. basilicum and L. maackii extracts </p><p>285having strongest effects on germination of B. oleracea (Fig. 5). Extracts of R. ficaria had</p><p>286stronger effects than extracts of L. maackii on germination of O. basilicum and L. sativa. </p><p>287The effect of extract concentration varied with extract species, with greater inhibition of </p><p>288germination with increasing concentration in extracts of A. petiolata and R. ficaria </p><p>289compared to extracts of L. maackii, which had smaller changes with increasing extract </p><p>290concentration (Fig. 6). Additionally, there was a significant three way interaction of test </p><p>291species, extract concentration and extract species (Table 1). Essentially, each test species</p><p>292responded to increasing concentration of extracts of each species in different ways. For </p><p>293example, while increasing concentrations of L. maackii extract had strong effects on </p><p>13 13 294germination of B. oleracea, increasing concentration of L. maackii had little effects on </p><p>295germination of L. sativa and O. basilicum (Fig. 7).</p><p>296</p><p>297Discussion</p><p>298 In our experiments, we confirmed the presence of allelopathy from leaves of three</p><p>299invasive Midwestern species and, more importantly, provided information on the </p><p>300comparative effect of each. Pisula and Meiners (2010) similarly used standardized </p><p>301methods to compare a suite of 10 invasive species, but they did not use either L. maackii </p><p>302or R. ficaria in their study. Pisula and Meiners (2010) found A. petiolata to be one of the </p><p>303four highest inhibitory invasive species, though only one test species, radish, was used. </p><p>304Our comparative approach was enhanced by the use of multiple test species, as previous </p><p>305work shows that allelopathic effects vary with test species (Prati and Bossdorf 2004, Orr </p><p>306and Rudgers, 2005, McEwan et al. 2010).</p><p>307 Allelopathic effects of each invasive species varied with test species. Generally, </p><p>308effects of extracts of L. maackii were greatest on species from the Brassicaceae, while </p><p>309extracts of A. petiolata and R. ficaria had the highest inhibitory effect on species in other </p><p>310families (Asteraceae and Laminaceae). Extracts of A. petiolata did not strongly affect the</p><p>311two species in the Brassicaceae, as was found in previous work (Cipollini et al. 2008a). </p><p>312This is most likely caused by the similar chemical composition of plants in the same </p><p>313family, which makes A. thaliana and B. oleracea more resistant to the effects of these </p><p>314chemicals. Effects of extracts of R. ficaria were generally weaker though still had </p><p>315allelopathic effects, particularly at the highest concentration. Ranunculus ficaria had </p><p>316strongest effects on germination of L. sativa and O. basilicum. </p><p>14 14 317 Allelopathic effects of each invasive species also varied by experimental venue. </p><p>318Extracts of R. ficaria showed a trend to reduce reproduction and to delay flowering in A. </p><p>319thaliana in potting soil, while extracts of R. ficaria significantly inhibited silique </p><p>320production of A. thaliana in field soil. There was also higher seed production in potting </p><p>321soil compared to field soils, suggesting differing growing conditions, which may have </p><p>322influenced the differential response to allelopathy (Cipollini et al. 2008a, Cipollini and </p><p>323Dorning 2008). Interestingly, we found little long-term effect of extract of L. maackii on </p><p>324germination in A. thaliana in potting soil, as germination was only delayed by 2 days. </p><p>325This contrasts previous work, which showed 50% reduction of germination of A. thaliana</p><p>326on filter paper after one week (Cipollini and Dorning 2006). There was no significant </p><p>327effect of extract concentration on response variables in potting soil and only a near-</p><p>328significant effect in field soil, in comparison to previous work that found strong effects of</p><p>329concentration in similar experimental conditions (Cipollini et al. 2008a). In comparison, </p><p>330differing concentrations did affect germination on paper. Further, the concentration </p><p>331affect varied with extract species and with test species, increasing the difficulty in finding</p><p>332a generalizable result from this study.</p><p>333 While our study provides some interesting insights into the comparative effects of</p><p>334allelopathy for these three species, there is still much research to be done to fully evaluate</p><p>335the allelopathic potential of these species in the field. In order to evaluate whether the </p><p>336allelopathic effects truly represent novel weapons to native plants, a comparative </p><p>337approach using co-occurring native species should be used (Barto et al., 2010b, McEwan </p><p>338et al. 2010). Additionally, a combination of field and laboratory experiments should seek</p><p>339to identify allelopathic compounds and determine their bioactivity and persistence in situ </p><p>15 15 340(Inderjit and Callaway 2003, Barto and Cipollini 2009). Nevertheless, our study provides</p><p>341important information on the relative allelopathic impact of each invasive species, as well</p><p>342as illustrates the importance of using multiple test species and experimental conditions to </p><p>343incorporate consideration of differing sensitivities to and conditions for allelopathic </p><p>344effects. Finally, our study also importantly provides additional information about the </p><p>345allelopathic potential of R. ficaria, a species for which there is no published information </p><p>346despite increasing interest in its role as an invasive species (Axtell et al. 2010).</p><p>347</p><p>348Acknowledgements</p><p>349 Doug Burks, Don Troike, Doug Woodmansee, and the students of BIO 440/441 </p><p>350provided valuable comments throughout the design and completion of this experiment. </p><p>351Don Cipollini also provided assistance and expertise when needed. We thank </p><p>352Wilmington College’s Instructional Development and Resources Committee for </p><p>353supporting a writing workshop during which this paper was produced. We thank Laura </p><p>354Struve and Michele Beery for creating and facilitating this workshop and all the </p><p>355participants for their support.</p><p>356</p><p>357References</p><p>358Axtell, A., DiTommaso, A., and Post, A. 2010. Lesser celandine (Ranunculus ficaria): A</p><p>359 threat to woodland habitats in the northern United States and southern Canada. </p><p>360 Invasive Plant Sci. Manage., 3: 190-196.</p><p>16 16 361Bais, H. P., Vepachedu, R., Gilroy, S., Callaway, R., and Vivanco, J. M. 2003. </p><p>362 Allelopathy and exotic plant invasion: From molecules and genes to species </p><p>363 interactions. Science, 301: 1377-1380.</p><p>364Barto, E. K., and Cipollini, D. 2009. Half-lives and field soil concentrations of Alliaria </p><p>365 petiolata secondary metabolites. Chemosphere, 76: 71-75. </p><p>366Barto, K., Friese, C., and Cipollini, D. 2010a. Arbuscular mycorrhizal fungi protect a </p><p>367 native plant from allelopathic effects of an invader. J. Chem. Ecol., 36: 351-360. </p><p>368 Barto, E. K, Powell, J. R., and Cipollini, D. 2010b. How novel are the chemical </p><p>369 weapons of garlic mustard in North American forest understories? Biol. </p><p>370 Invasions, 10: 3465-3471.</p><p>371Callaway, R., and Aschehoug, E. 2000. Invasive plant versus their new and old </p><p>372 neighbors: a mechanism for exotic invasion. Science, 290: 521-523.</p><p>373Callaway R.M., and Ridenour, W. M. 2004. Novel weapons: invasive success and the </p><p>374 evolution of increased competitive ability. Front. Ecol. Environ., 2: 436-443.</p><p>375Callaway, R. M., Cipollini, D., Barto, K., Thelen, G. C., Hallett, S. G., Prati, Dr., Stinson,</p><p>376 K., and Klironomos, J. 2008. Novel weapons: invasive plant suppresses fungal </p><p>377 mutualists in America but not in its native Europe. Ecology, 89: 1043-1055.</p><p>378Carlson, A. M., and Gorchov, D. L. 2004. Effects of herbicide on the invasive biennial </p><p>379 Alliaria petiolata (garlic mustard) and initial responses of native plants in a </p><p>380 southwestern Ohio forest. Rest. Ecol., 12: 559-567. </p><p>381Chevallier, A. 1996. The encyclopedia of medicinal plants. DK, New York. </p><p>17 17 382Cipollini, D., and Dorning, M. 2008. Direct and indirect effects of conditioned soils and </p><p>383 tissue extracts of the invasive shrub, Lonicera maackii, on target plant </p><p>384 performance. Castanea, 73: 166-176.</p><p>385Cipollini, D., and Gruner, W. 2007. Cyanide in the chemical arsenal of garlic mustard, </p><p>386 Alliaria petiolata. J. Chem. Ecol., 33: 85-94.</p><p>387Cipollini, D., Mbagwu, J., Barto, K., Hillstrom, C., and Enright, S. 2005. Expression of </p><p>388 constitutive and inducible chemical defenses in native and invasive populations of</p><p>389 garlic mustard (Alliaria petiolata). J. Chem. Ecol., 31: 1243-1255.</p><p>390Cipollini, D., Stevenson, R., and Cipollini, K. 2008a. Contrasting effects of </p><p>391 allelochemicals from two invasive plants on the performance of a non-</p><p>392 mycorrhizal plant. Int. J. Plant Sci., 169: 371-375.</p><p>393Cipollini, D., Stevenson, R., Enright, S., Eyles, A., and Bonello, P. 2008b. Phenolic </p><p>394 metabolites in leaves of the invasive shrub, Lonicera maackii, and their potential </p><p>395 phytotoxic and anti-herbivore effects. J. Chem. Ecol., 34: 144-152.</p><p>396Cipollini, K. A., and Schradin, K. D. 2011. Guilty in the court of public opinion: Testing </p><p>397 presumptive impacts and allelopathic potential of Ranunculus ficaria. Am. Midl. </p><p>398 Nat., 166: 63-74.</p><p>399Cipollini, K., McClain, G., and Cipollini, D. 2008. Separating above- and belowground </p><p>400 effects of Alliaria petiolata and Lonicera maackii on the performance of </p><p>401 Impatiens capensis. Am. Midl. Nat., 160: 117-128.</p><p>402Collier, M. H., Vankat, J. L., and Hughes, M. R. 2002. Diminished species richness and </p><p>403 abundance below Lonicera maackii, an invasive shrub. Am. Midl. Nat., 147: 60-</p><p>404 71.</p><p>18 18 405Dorning, M., and Cipollini, D. 2006. Leaf extracts of the invasive shrub, Lonicera </p><p>406 maackii inhibit seed germination of three herbs with no autotoxic effects. Plant </p><p>407 Ecol., 184: 287-296. </p><p>408Ehrenfeld, J. G. 2006. A potential source of information for screening and monitoring </p><p>409 the impact of exotic plants on ecosystems. Biol. Invasions, 8: 1511-1521.</p><p>410Gould, A. M. A., and Gorchov, D. L. 2000. Effects of the invasive shrub Lonicera </p><p>411 maackii on the survival and fecundity of three species of native annuals. Am. </p><p>412 Midl. Nat., 144: 36-50.</p><p>413Hartman, K. M., and McCarthy, B. C. 2004. A dendro-ecological study of forest </p><p>414 overstorey productivity following the invasion of the non-indigenous shrub </p><p>415 Lonicera maackii. J. Appl. Veg. Sci., 10: 3-14. </p><p>416Hierro, J. L., and Callaway, R. M. 2003. Allelopathy and exotic plant invasion. Plant </p><p>417 Soil, 256: 29-39.</p><p>418Inderjit, and Callaway, R. M. 2003. Experimental designs for the study of allelopathy. </p><p>419 Plant Soil, 256: 1-11.</p><p>420Keane, R.M, and Crawley, M.J. 2002. Exotic plant invasions and the enemy release </p><p>421 hypothesis. Trends Ecol. Evol., 17: 164-170.</p><p>422Kolar, C. S., and Lodge, D. M. 2001. Progress in invasion biology: Predicting invaders. </p><p>423 Trends Ecol. Evol., 16: 199-204.</p><p>424Kulmatiski, A., and Beard, K. H., 2006. Activated carbon as a restoration tool: Potential </p><p>425 for control of invasive plants in abandoned agricultural fields. Restor. Ecol., 14: </p><p>426 251-257.</p><p>19 19 427Levine, J. M., Vilà, J. M., D’Antonio, C. M., Dukes, J. S., Grigulis, K., and Lavorel, S. </p><p>428 2003. Mechanisms underlying the impacts of exotic plant invasions. Proc. R. Soc.</p><p>429 Lond. B, 270: 775-781.</p><p>430McCarthy, B. C. 1997. Response of a forest understory community to experimental </p><p>431 removal of an invasive nonindigenous plant (Alliaria petiolata, Brassicaceae). In </p><p>432 Assessment and management of plant invasions. Edited by J.O. Luken and J. W. </p><p>433 Thieret. Springer-Verlag, New York, pp. 117-130.</p><p>434McCarthy, and B. C., Hanson, S. L. 1998. An assessment of the allelopathic potential of</p><p>435 the invasive weed Alliaria petiolata (Brassicaceae). Castanea, 63: 68-73.</p><p>436Meekins, J. F., and McCarthy, B. C. 1999. Competitive ability of Alliaria petiolata </p><p>437 (garlic mustard, Brassicaceae), an invasive, nonindigenous forest herb. Int. J. </p><p>438 Plant Sci., 160: 743–752. </p><p>439McEwan, R. W., Arthur-Paratley L. G., Rieske L. K., and Arthur M. A., 2010. A multi-</p><p>440 assay comparison of seed germination inhibition by Lonicera maackii and co-</p><p>441 occuring native shrubs. Flora, 205: 475-483.</p><p>442McGeoch, M. A., Butchart, S. H. M., Spear, D., Marais, E., Kleynhans, E. J., Symes, A., </p><p>443 Chanson, J., and Hoffman, M. 2010. Global indicators of biological invasion: </p><p>444 species numbers, biodiversity impact and policy responses. Diversity Distrib., 16: </p><p>445 95-108.</p><p>446Orr, S. P., Rudgers, J. A., and Clay, K. 2005. Invasive plants can inhibit native tree </p><p>447 seedlings: testing potential allelopathic mechanisms. Plant Ecol., 181: 153-165.</p><p>448Pennacchio M., Jefferson L., and Havens, K. 2005. Arabidopsis thaliana: a new test </p><p>449 species for phytotoxic bioassays. J. Chem. Ecol., 31: 1877-1885.</p><p>20 20 450Pimentel, D., Lach, L., Zuniga, R., and Morrison, D. 2005. Update on the environmental</p><p>451 and economic costs associated with alien-invasive species in the United States. </p><p>452 Ecol. Econ., 52: 273-288.</p><p>453Pisula, N. L., and Meiners, S. J. 2010. Relative allelopathic potential of invasive plant </p><p>454 species in a young disturbed woodland. J. Torr. Bot. Soc., 137: 81-87.</p><p>455Prati, D., and Bossdorf O. 2004. Allelopathic inhibition of germination by Alliaria </p><p>456 petiolata (Brassicaceae). Am. J. Bot., 91: 285-288.</p><p>457Rice, E. L. 1974. Allelopathy. Academic Press, New York. </p><p>458Ridenour, W. M., and Callaway, R. M. 2001. The relative importance of allelopathy in </p><p>459 interference: The effects of an invasive weed on a native bunchgrass. Oecologia, </p><p>460 126: 444-450. </p><p>461Ryan, B. F., Joiner, B. L., and Cryer, J. D. 2005. Minitab handbook, 5th Ed. Thomson </p><p>462 Brooks/Cole, Belmont.</p><p>463Sakai, A. K., Allendorf, F. W., Holt, J. S., Lodge, D. M., Molofsky, J., With, K. A., </p><p>464 Baughman, S., Cabin, R. J., Cohen, J. E., Ellstrand, N. C., McCauley, D. E., </p><p>465 O'Neil, P., Parker, I. M., Thompson, J. N., Weller, S. G., Fautin, D. G., Futuyma, </p><p>466 D. J., and Shaffer, H. B. 2001. The population biology of invasive species. </p><p>467 Annu. Rev. Ecol. Syst., 32: 305-322.</p><p>468Stinson, K. A., Campbell, S. A., Powell, J. R., Wolfe, B. E., Callaway, R. M., Thelen, G. </p><p>469 C., Hallett, S. G., Prati, D., and Klironomos, J. 2006. Invasive plant suppresses </p><p>470 the growth of native tree seedlings by disrupting below-ground mutualisms. PLoS</p><p>471 Biol., 4:e140. doi:10.1371/journal.pbio.0040140.</p><p>472U.S. Department of Agriculture (USDA). 2011. The PLANTS Database [online].</p><p>21 21 473Vaughn, S. F., and Berhow, M. A. 1999. Allelochemicals isolated from tissues of the </p><p>474 invasive weed garlic mustard (Alliaria petiolata). J. Chem. Ecol., 25: 2495-2504.</p><p>475von Ende, C. N., 1993. Repeated-measures analysis: growth and other time-dependent </p><p>476 measures. In Design and Analysis of Ecological Experiments. Edited by S. M. </p><p>477 Scheiner and J. Gurevitch, J. Chapman and Hall, New York, pp. 113-135.</p><p>478Wilcove, D. S., Rothstein, D., Dubow, J., Phillips, A., and Losos, E. 1998. Quantifying </p><p>479 threats to imperiled species in the United States. Bioscience, 48: 607-615.</p><p>480Zhang, S., Jin, Y., Tang, J., and Chen, X. 2009. The invasive plant Solidago canadensis </p><p>481 L. suppresses local soil pathogens through allelopathy. Appl. Soil Ecol., 41: 215-</p><p>482 222.</p><p>22 22 483Table 1. Three-way Analysis of Variance (ANOVA) results for germination of three test</p><p>484species - Brassica oleracea, Lactuca sativa and Ocimum basilicum - treated with extracts </p><p>485of three invasive species - Alliaria petiolata, Lonicera maackii and Ranunculus ficaria at </p><p>486three extract concentrations.</p><p>487Source of variation Df F p</p><p>488Test Species 2 29.93 <0.001</p><p>489Extract Species 2 11.63 <0.001</p><p>490Extract Concentration 2 62.86 <0.001</p><p>491Extract Species*Test Species 4 11.42 <0.001</p><p>492Extract Species*Extract Concentration 4 3.50 0.011</p><p>493Test Species*Extract Concentration 4 0.85 0.495</p><p>494Extract Species* Test Species*Concentration 8 6.56 <0.001</p><p>495Error 81</p><p>496</p><p>23 23 497Figure legends</p><p>498Fig. 1. Mean number of seeds germinated (± SE) of Arabidopsis thaliana for control (0 </p><p>499g/mL) and three concentrations (0.1, 0.2 and 0.3 g fresh leaf/mL distilled H2O) of </p><p>500Lonicera maackii leaf extracts. Asterisks indicate dates for which there were significant </p><p>501differences between treatments. Letters indicate significant differences within each date </p><p>502using Tukey’s test at α = 0.05.</p><p>503</p><p>504Fig. 2. Mean number of siliques (± SE) of Arabidopsis thaliana in potting soil for </p><p>505treatments containing no invasive species (control) and separate leaf extracts of three </p><p>506invasive species - Alliaria petiolata, Lonicera maackii and Ranunculus ficaria. </p><p>507Treatments with different letters are significantly different from each other using Tukey’s</p><p>508test at α = 0.05. </p><p>509</p><p>510Fig. 3. Mean days to flowering (± SE) of Arabidopsis thaliana for treatments containing </p><p>511no invasive species (control) and separate leaf extracts of three invasive species - Alliaria</p><p>512petiolata, Lonicera maackii and Ranunculus ficaria. Treatments with different letters are</p><p>513significantly different from each other using Tukey’s test at α = 0.10. </p><p>514</p><p>515Fig. 4. Mean number of siliques (± SE) of Arabidopsis thaliana in field soil for </p><p>516treatments containing separate leaf extracts of three invasive species - Alliaria petiolata, </p><p>517Lonicera maackii and Ranunculus ficaria. Treatments with different letters are </p><p>518significantly different from each other using Tukey’s test at α = 0.05. </p><p>519</p><p>24 24 520Fig. 5. Mean number of seeds germinated (± SE) of three test species - Brassica </p><p>521oleracea, Lactuca sativa and Ocimum basilicum - treated with leaf extracts of three </p><p>522invasive species - Alliaria petiolata, Lonicera maackii and Ranunculus ficaria across </p><p>523three extract concentration treatments.</p><p>524</p><p>525Fig. 6. Mean number of seeds germinated (± SE) across three test species treated with </p><p>526leaf extracts of three invasive species - Alliaria petiolata, Lonicera maackii and </p><p>527Ranunculus ficaria – at three leaf extract concentrations (0.1, 0.2 and 0.3 g fresh leaf/mL </p><p>528distilled H2O).</p><p>529</p><p>530Fig. 7. Mean number of seeds germinated (± SE) of three test species - Brassica </p><p>531oleracea, Lactuca sativa and Ocimum basilicum - treated with leaf extracts of three </p><p>532invasive species - Alliaria petiolata, Lonicera maackii and Ranunculus ficaria at three </p><p>533extract concentrations. Asterisks indicate significant difference within each extract </p><p>534species from the control in one-way ANOVAs using Tukey’s test at α = 0.05.</p><p>535</p><p>536</p><p>537</p><p>538</p><p>25 25 10</p><p> d * e t</p><p> a 8 a n i</p><p> m a r</p><p> e 6 * G</p><p> s</p><p> d a</p><p> e 4 e</p><p>S b</p><p> f o</p><p>2 0 g/mL r</p><p> e b 0.1 g/mL b b 0.2 g/mL </p><p> m 0 b</p><p> u 0.3 g/mL N</p><p>1 2 3 4 5 6 7</p><p>539 Days</p><p>26 26 900 p = 0.049 a a 800 s e u q i</p><p> l ab i 700 S</p><p> b f o</p><p> r</p><p> e 600 b m u</p><p>N 500</p><p>400 Control A. petiolata L. maackii R. ficaria</p><p>540 Extract Type</p><p>27 27 p = 0.071 28 ab ab b g n i</p><p> r 26 e a w o l F</p><p> o 24 t</p><p> s y a</p><p>D 22</p><p>20 Control A. petiolata L. maackii R. ficaria</p><p>541 Extract Type</p><p>28 28 350 a</p><p> s 300 e</p><p> u b q b i l i S</p><p> f 250 o</p><p> r e b m</p><p> u 200 N</p><p>150 A. petiolata L. maackii R. ficaria</p><p>542 Extract Type</p><p>29 29 d A. petiolata e</p><p> t 12</p><p> a L. maackii n</p><p> i R. ficaria </p><p> m 10 r e G 8 s d e</p><p> e 6 S</p><p> f o</p><p> r 4 e b</p><p> m 2 u N 0 B. oleracea L. sativa O. basilicum</p><p>543 Test Species</p><p>30 30 d 0.1 g/mL e</p><p> t 12</p><p> a 0.2 g/mL n i 0.3 g/mL </p><p> m 10 r e G 8 s d e</p><p> e 6 S</p><p> f o</p><p> r 4 e b</p><p> m 2 u N 0 A. petiolata L. maackii R. ficaria</p><p>544 Extract Type</p><p>31 31 10 *</p><p>8 * * 6</p><p>4 A. petiolata * L. maackii 2 R. ficaria</p><p>0 Brassica oleracea d e t a</p><p> n 10 i * m *</p><p> r 8 e G</p><p>6 * s d *</p><p> e 4 * e S</p><p>2 f o</p><p> r 0 Lactuca sativa e b m</p><p> u 10 N</p><p>8 *</p><p>6 *</p><p>4 * *</p><p>2 *</p><p>0 Ocimum basilicum</p><p>0.1 0.2 0.3 Extract Concentration (in 545 g fresh leaf/mL distilled water)</p><p>32 32</p>
Details
-
File Typepdf
-
Upload Time-
-
Content LanguagesEnglish
-
Upload UserAnonymous/Not logged-in
-
File Pages32 Page
-
File Size-