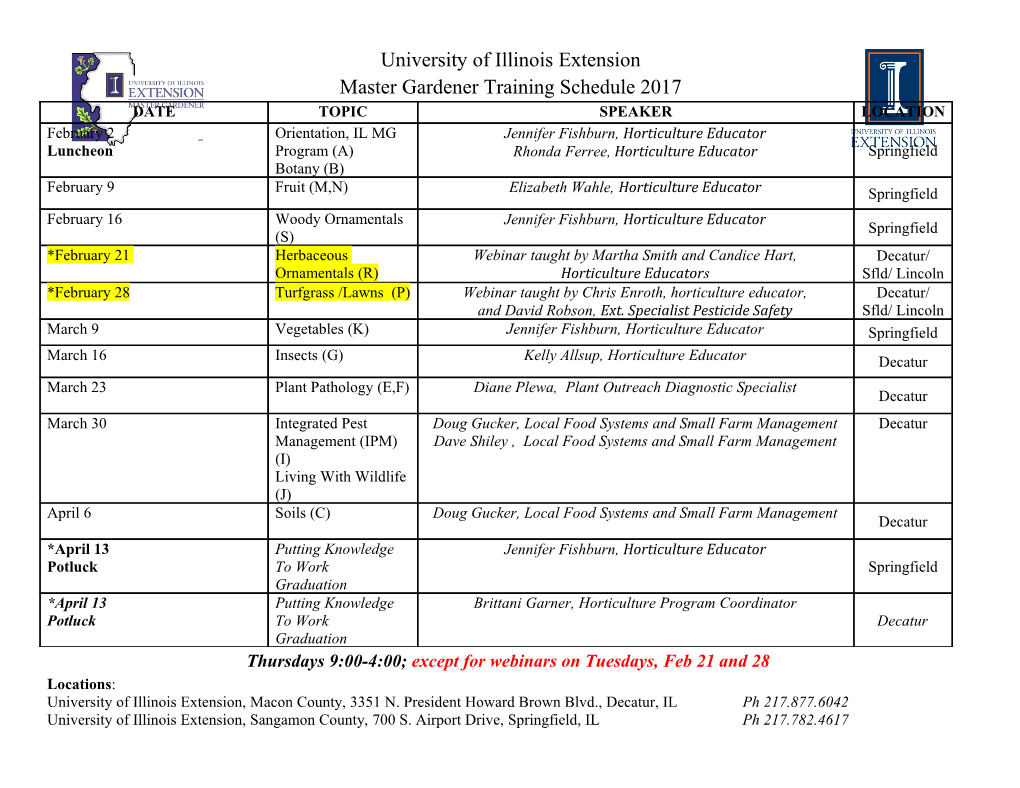
<p> EAR 203, 2013-2014 Name: ______Date: ______Block: _____</p><p>EAR 203 EARTH SYSTEM SCIENCE SYRACUSE UNIVERSITY LAB 5B - PROPERITES OF SEAWATER </p><p>PURPOSE: To empirically measure and compare the properties of distilled, tap and sea water.</p><p>Importance of the Ocean: Since ancient times, the ocean has served as a source of food such as fish and shellfish. Rock strata beneath the ocean floor are an important source of petroleum and natural gas. Seawater is a source of sodium chloride and other salts. Seawater from which salt has been removed is used for drinking and for agri - cultural and industrial purposes.</p><p>Seawater is ordinary water (H2O) that contains dissolved mineral ions in an average concentration of 3.5% by weight. Seawater also contains numerous organic and inorganic particles in suspension (undissolved). The mineral ions come from eroded crustal rocks and volcanic activity (hydrothermal vents and recycling in the upper mantle). Much of the crustal debris resulting from erosion is ultimately carried to the sea by rivers, glaciers, and winds, and thus several billion tons of salts are added to the sea each year.</p><p>THE BIG PICTURE: The Ocean plays an important role in the water cycle – the constant movement of water from the atmosphere to the earth's surface and back. The ocean and its currents influence the climate of many areas.</p><p>Salinity and the Main Mineral Ions: The amount of dissolved inorganic solids in seawater, expressed in parts per thousand by weight, is referred to as its salinity. The average salini- ty of seawater is 3.5% or 35‰ or 35ppt. The solvent is the substance doing the dissolving, and in sea water it is the water. The solute is the material being dissolved. Water dissolves salts by surrounding the atoms in the salt molecule and neutralizing the ionic bond holding the molecule together. Dissolved salts form cations (positively charged ions) and anions (negatively charged ions). Ions are atoms with a different number of elec- trons than of protons, and are therefore electrically charged. Interestingly, the proportions of dissolved ions in seawater are constant regardless of salinity. This implies that the oceans are chemically well-mixed and that ocean salinity varies almost entirely as a result of the addition or removal of pure wa- ter (evaporation of pure water, freezing and thawing), not the addition or removal of salts. Salinity affects marine organisms because the process of osmosis transports water towards a higher concentration through cell walls. A fish with a cellular salinity of 1.8% will swell in fresh water and dehydrate in salt water. So, saltwater fish drink water copi - ously while excreting excess salts through their gills. Freshwater fish do the opposite by not drinking but excreting copious amounts of urine while losing little of their body salts. Many marine plants (seaweeds) and lower organisms have no mechanism to control osmosis, which makes them very sensitive to the salinity of the water in which they live. </p><p>- - + The main nutrients for plant growth are nitrogen (N as in nitrate NO 3 , nitrite NO2 , ammonia NH4 ), phosphorus (P as phosphate 3- PO4 ) and potassium (K) followed by sulfur (S), magnesium (Mg), and calcium (Ca). Iron (Fe) is an essential component of enzymes and is readily available in soil, but not in sea water (0.0034ppm). This makes iron an essential nutrient for plankton growth. Phyto - plankton organisms (like diatoms) that make shells of silicon compounds need dissolved silica (SiO2) which at 3ppm can be rather limiting.</p><p>Conductivity: Salts break down into their ions when dissolved in water. For example, sodium chloride (NaCl) breaks down into Na + and Cl-. Conductivity varies with the concentration and mobility of ions present and with water temperature, and is used to deter - mine salinity.</p><p>Lab 5B – Properties of Seawater Page 1 EAR 203, 2013-2014 Dissolved Gases in Seawater: Seawater has many different gases dissolved in it. In order of decreasing abundance, the major gases in the sea are nitrogen, oxygen, carbon dioxide, and the noble gases, argon (Ar), neon (Ne) and heli - um (He). Seawater exchanges these gases with the atmosphere to keep a balance between the ocean and the atmos- phere. This exchange is helped by the mixing of the surface by wind and waves. Some of the properties of seawater affect how much gas can be dissolved in it. As salinity increases, the amount of gas dissolved decreases because more water molecules are immobilized by the salt ion. Cold water holds more gas than warm water; as water temperature increases, the increased mobility of gas molecules makes them escape from the water, thereby reducing the amount of gas dissolved. Deep water, which has a high pressure, holds more gas than shallow water. Carbon dioxide is much more soluble in water than in air. The use and creation of dissolved gases by living things can over-ride the effect of these properties. For example, warm water with lots of plankton in it can hold more carbon dioxide than cold water with few living things in it. Inert gases like nitrogen and argon do not take part in the processes of life and are thus not affected by plant and animal life. But non-conservative gases like oxygen and carbon dioxide are influenced by sea life. Dissolved oxygen and carbon dioxide are vital for marine life. Marine plants use dissolved carbon dioxide, sunlight and water to make carbohydrates through the process of photosynthesis. This process releases oxygen into the water. Marine organisms use oxygen for respiration. This process produces energy from carbohydrates, and releases carbon dioxide and water as byproducts. Marine animals with gills, such as fish, use these organs to extract oxygen from the seawater. Bacteria are even capable of using up all the oxygen in seawater. When water is warmed, it becomes more saturated with gases, eventually resulting in bubbles leaving the liquid. Fish like sun- bathing or resting near the warm surface or in warm water outfalls because oxygen levels there are higher. The elevated tempera- ture also enhances their metabolism, resulting in faster growth, and perhaps a sense of wellbeing. Due to lack of oxygen, deep sea fish cannot be very active. If the whole ocean were to warm up, the equilibrium with the atmosphere would change towards more carbon dioxide (and oxygen) being released to the atmosphere, thereby exacerbating global warming.</p><p>Nutrients are Chemicals Essential for Life: Major nutrients in the sea are compounds of nitrogen, phosphorus, carbon and silicon. Because of usage, nutrients are scarce at the surface, and their concentrations are measured in parts per million (ppm). Nutrient concentrations vary greatly over time, and because of this they are considered a non-conservative property of the ocean. Trace elements occur in minute quantities and are usually measured in parts per million (ppm) or parts per billion (ppb). Even in small quantities they are important in either promoting life, or killing it. Marine organic compounds occur in low concentrations and consist of large complex molecules, such as fat, proteins, carbohydrates, hormones and vitamins. These organic compounds are produced by organisms or through decay.</p><p>Chlorinity: Chlorinity is the amount of halogens (chlorine, bromine, iodine and fluorine) in seawater and is expressed as grams/kilo - gram or ppm (parts per million). As the chloride ion, which is part of common salt and other compounds, chlorine is abundant in na- ture and necessary to most forms of life, including humans. In its elemental form (Cl 2 or "dichlorine"), under standard conditions, chlorine is a powerful oxidant and is used in bleaching and disinfectants, as well as an essential reagent in the chemical industry. As a common disinfectant, chlorine compounds are used in swimming pools to keep them clean and sanitary. In the upper atmosphere, chlorine-containing molecules such as chlorofluorocarbons have been implicated in the destruction of the ozone layer. pH: pH is a measure of how acidic/basic water is and ranges from 0 - 14, with 7 being neutral. pH values less than 7 indicate acidity, and pH values greater than 7 indicate a base. pH is really a measure of the relative amount of free hydrogen (H +) and hydroxyl (OH-) ions in the water. Water that has more free hydrogen ions is acidic, whereas water that has more free hydroxyl ions is basic. Since pH can be affected by chemicals in the water, pH is an important indicator of water that is chemically changing. pH is reported in log - arithmic units; each number represents a 10-fold change in the acidity/baseness of the water. Water with a pH of five is ten times more acidic than water having a pH of six. The pH of water determines solubility and biological availability (amount that can be utilized by aquatic life) of chemi- cal constituents such as nutrients (phosphorus, nitrogen, and carbon) and heavy metals (lead, copper, cadmium, etc.). For example, in addition to affecting how much and what form of phosphorus is most abundant in the water, pH also determines whether aquatic life can use it. In the case of heavy metals, the degree to which they are soluble deter- mines their toxicity. Metals tend to be more toxic at lower pH because they are more soluble.</p><p>Lab 5B – Properties of Seawater Page 2 EAR 203, 2013-2014 Alkalinity: Alkalinity is the water's capacity to resist changes in pH that would make the water more acidic. This ability is commonly known as "buffering capacity." For example, if you add the same weak acid solution to two vials of water - both with a pH of 7, but one with no buffering capacity (e.g., zero alkalinity) and the other with buffering capacity (e.g., alkalinity of 50 mg/l), - the pH of the zero alkalinity water will immediately drop while the pH of the buffered water will change very little, or not at all. Alkalinity is important for fish and aquatic life because it protects or buffers against rapid pH changes. Living organisms, especially aquatic life, function best in a pH range of 6.0 to 9.0. Alkalinity is a measure of how much acid can be added to a liquid without caus - ing a large change in pH. Higher alkalinity levels in surface waters will buffer acid rain and other acid wastes and prevent pH changes that are harmful to aquatic life. Acid shock may occur in spring when acid snows melt, thunderstorms, natural discharges of tannic waters, "acid rain", acidic dryfall, and other discharges enter the stream. If increasing amounts of acids are added to a body of water, the water's buffering capacity is consumed. If additional buffering material can be obtained from surrounding soils and rocks, the alkalinity level may eventually be restored. However, a temporary loss of buffering capacity can permit pH levels to drop to those harmful to life in the water. </p><p>Density: Density is a physical property of matter, as each element and compound has a unique density associated with it. Density de- fined in a qualitative manner as the measure of the relative "heaviness" of objects with a constant volume. The density of a material is defined as its mass per unit volume. Each substance in nature has a definite density that is characteristic of the material. However, this char - acteristic density applies only to pure substances at standard temperature and pressure. This is especially true of gases, which tend to expand and become less dense upon heating. Solids and liquids also expand and contract in response to heating and cooling, but to a lesser degree. Water is the only substance on Earth that is found naturally in all three physical states: as a gas, liquid, and solid. At temperatures below freezing, each state may even be present at the same time. Most solids normally sink in their own liquid, and yet we observe that ice cubes float in a glass of water. In contrast, water behaves much like other liquids and becomes less dense as it enters a gaseous state. However, as water warms from its melting point at 0°C, something strange occurs. The density of water actually in- creases as its warms, until it reaches a maximum density at 4°C. Below 4°C, water actually expands slightly and becomes less dense. This unique property of water has some important consequences. Since water near 0°C is less dense than water at 4°C, and because ice floats on water when it forms, ponds and lakes at the Earth’s surface freeze from the top down. This layer of floating ice acts as an insulator for the water below it; it keeps most lakes from freezing over completely. This process allows aquatic organisms to sur- vive through the winter in protected bottom waters of the lake. The density of seawater varies with salinity, temperature, and depth. At normal atmospheric pressure, seawater has a density that is typically about 3% greater than that of freshwater. This greater density makes it easier to float and swim in seawater than in fresh - water. The density of ocean water can also vary widely from place to place with changes in temperature, the amount of dissolved solids, and the amount of material held in suspension. The large amounts of dissolved solids present in ocean water make it more dense than pure freshwater, since the dissolved solids add mass to the water. Since salts are the most abundant dissolved solids in the ocean, a change in salinity can affect the water’s density. Salinity can be locally affected by the amount of freshwater and salts added to the sea by rivers and runoff, and by the rate of evaporation and freezing. Temperature also affects the density of ocean water, with density increasing as temperature decreases. The temperature of the ocean is basically determined by the amount of infrared radiation it receives from the sun. The densest ocean water is found in the cold temperatures of the polar regions. In the dynamic movement of the world’s ocean waters, cold heavy water sinks below warmer and light water. Water of high salinity is also heavier than the water of low salinity and sinks beneath it. Ocean water mud - died by heavy concentrations of suspended earth materials will also sink beneath light, clear water. At any particular spot on the ocean’s surface, density variation with depth may be influenced more strongly by one variable than another. In warm, tropical regions, salinity variation with depth is relatively small; however, the den- sity may increase with depth as the temperature decrease. In cold polar regions, salinity is low at the surface and increases with depth. In these areas, the increase in density with depth is largely due to the salinity increase. </p><p>Lab 5B – Properties of Seawater Page 3 EAR 203, 2013-2014 Procedure: </p><p>Part 1: Chemical Composition of Seawater Objective: to determine the chemical composition of seawater using the following ingredients. 1. Obtain a sheet of weigh paper. 2. Carefully measure the following ingredients and put them in a 250 mL beaker. (Round to the nearest hundredth) Salt Mass (grams) Sodium chloride (NaCl) 4.696</p><p>Magnesium chloride (MgCl2) 0.996</p><p>Sodium sulfate (Na2SO4) 0.784</p><p>Calcium chloride (CaCl2) 0.220 Potassium chloride (KCl) 0.132</p><p>Sodium bicarbonate (NaHCO3) 0.038 Potassium bromide (KBr) 0.020 3. Using a graduated cylinder, add 193.114 ml of distilled water to the beaker of salts. 4. Carefully mix with a stirring rod. 5. Complete Data Table 1. </p><p>Part 2: Conductivity of Seawater Objective: to determine the conductivity of seawater, tap water, and distilled water 1. Obtain the conductivity tester. 2. Test the conductivity of the SEAWATER you just made. 3. Record in Data Table 2 whether or not the seawater conducted electricity, and the strength. 4. Thoroughly wipe of the prongs of the conductivity tester. ***This is VERY important to prevent compound contamination. Most error in results is due to wet, contaminated equipment. 5. Obtain a 250 mL beaker. 6. Fill the cup with 200 mL of DISTILLED water. 7. Test the conductivity the distilled water. 8. Record in Data Table 2 whether or not the distilled water conducted electricity, and the strength. 9. Thoroughly wipe of the prongs of the conductivity tester. ***This is VERY important to prevent compound contamination. Most error in results is due to wet, contaminated equipment. 10. Pour the water down the drain. 11. Repeat steps 6-10 using TAP water. </p><p>Part 3: Dissolved Oxygen Objective: to compare the dissolved oxygen content of distilled water, tap water and sea water using the following procedure. 1. Obtain the Dissolved Oxygen test kit. 2. Fill the sample cup provided to the 25 mL mark with seawater. 3. Place the tapered tip of the ampoule into one of the 4 depressions in the bottom of the sample cup. Snap the tip by squeezing the test ampoule toward the side of the cup. The sample will fill the ampoule and begin to mix with the reagent inside. ****NOTE: A small gas bubble will remain in the ampoule. 4. Remove the fluid filled test ampoule from the cup. Keeping the open end downward, cover the tip of the ampoule with a finger. ****NOTE: Cover the end of the Snap Test ampoule with a piece of tissue before shaking contents. 5. Mix the contents of the ampoule be inverting it several times, allowing the air bubble inside to travel from end to end. 6. Wipe all liquid from the exterior of the ampoule and wait 2 minutes. 7. After waiting 2 minutes, use the comparator to determine the level of dissolved oxygen in the sample. 8. Record your result in Data Table 3. 9. Dump the contents of the sample cup down the drain. 10. Discard the used ampoule in the broken glass receptacle. 11. Rinse and dry the sample cup.</p><p>Lab 5B – Properties of Seawater Page 4 EAR 203, 2013-2014 12. Repeat steps 2-9 to first test the dissolved oxygen content of tap water and then the dissolved oxygen content of your distilled water. </p><p>Part 4: pH Objective: to compare the pH of distilled water, tap water and seawater using the following procedure. 1. Dip one piece of pHydrion paper in your seawater. 2. Match the reaction color to the color chart from the pHydrion vile. Record your result in Data Table 3. 3. Obtain a cup. 4. Fill the cup with a splash of distilled water. 5. Test the pH of the distilled water (repeating steps 1-2). 6. Pour the distilled water down the drain. 7. Fill the cup with a splash of tap water. 8. Test the pH of the tap water (repeating steps 1-2). 9. Pour the tap water down the drain.</p><p>Part 5: Chlorine, hardness, alkalinity and pH Objective: to compare the amount of total chlorine, free chlorine, total hardness, total alkalinity and pH of distilled water, tap water and seawater using the following procedure. 1. Obtain 3, 5-in-1 Water Quality Test strips. 2. Dip the entire strip into the seawater for 1 second and remove 3. Hold the strip level for 30 seconds. (Do not hake excess water from the test strip) 4. Compare TOTAL HARDNESS, TOTAL ALKALINITY and pH pads to the color chart on the side of the test strip container. 5. Dip the strip into the seawater again and move back and forth for 30 seconds. 6. Compare the CHLORINE pads to the color chart on the side of the test strip container. 7. Record your results in Data Table 4. 8. Repeat steps 2-7 using approximately 150 ml of DISTILLED water and 150 ml of TAP water respectively. </p><p>Part 6: Density of Ice (Day 1) Objective: To determine the density of frozen saltwater, tap water and distilled water. 1. Obtain one 25 mL graduated cylinder per lab table. 2. Label your graduated cylinder with a piece of masking tape. 3. Record the mass of the cylinder in Data Table 5. 4. Place EXACTLY 20 mL of either distilled, tap or seawater in the graduated cylinder. Record this volume in Data Table 5. 5. Determine the mass of the graduated cylinder and the water. (Round to the 100 th decimal place). Record the mass of the cylinder in Data Table 5. 6. Calculate the mass of the water alone. (Round to the 100th decimal place). Record the mass of the cylinder in Data Table 5. 7. Calculate the density of the water. (Round to the 100th decimal place). Record the density in Data Table 5. 8. Carefully place the graduated cylinder in the freezer in the Earth Science prep room (between 204 & 205).</p><p>PART 7: Freshwater Density Change</p><p>Lab 5B – Properties of Seawater Page 5 EAR 203, 2013-2014 Objective: To observe how the density of water changes as temperature decreases. 1. Obtain the following materials a. Density bottle apparatus (see Fig. 1 to the right) CAREFUL! b. Nail c. Large coffee can or container d. Ice and table salt 2. Carefully uncork the bottle and place a nail in the bottle. 3. Fill the bottle right up to the top (overflowing) with fresh (distilled) water, making sure that as little air is in the bottle as possible. 4. Install the rubber stopper assembly in the mouth of the bottle to seal it tightly. 5. Record the temperature and height of the water in the glass tube in Data Table 6. a. Each line = 0.5 cm. Measure to tenths place. 6. Place crushed ice in the bottom of the coffee can (or comparable container) 7. Lower the density bottle into the can. 8. Pour crushed ice all around the bottle right up to the neck of the bottle. 9. Carefully and steadily spin the bottle in the can of ice while adding small amounts of salt to the ice. 10. Record the temperature and height of the water every 30 seconds for 12 minutes. 11. Graph your data in the space provided.</p><p>PART 8: Determining the Actual Density of Sea Water Objective: To calculate the actual density of seawater. 1. Obtain a GLASS 25 mL graduated cylinder. 2. Determine the mass of the cylinder. Record this mass in Data Table 7. 3. Place EXACTLY 5 mL of seawater from your beaker into the graduated cylinder. (Use a pipet for accuracy) 4. Determine the mass of the graduated cylinder and the seawater. Record this mass in Data Table 7. 5. Calculate the mass of the seawater alone. Record this mass in Data Table 7. 6. Calculate the density of the seawater. Record the density in Data Table 7. 7. Add EXACTLY 5 more mL of seawater to the graduated cylinder. 8. Determine the mass of the graduated cylinder and the seawater. Record this mass in Data Table 7. 9. Calculate the mass of the seawater alone. Record the mass of the cylinder in Data Table 7. 10. Calculate the density of the seawater. Record the density in Data Table 7. 11. Continue this procedure of adding seawater to the graduated cylinder in EXACTLY 5 mL (that is, repeating steps 7-10) increments until you reach 25 mL. Calculate the density of the seawater for each volume. 12. Calculate your average density for seawater. Record the density in Data Table 7.</p><p>DAY 2: Density of Ice 1. Retrieve your graduated cylinder from the freezer. 2. Determine the mass of the ice and graduated cylinder. (Round to the 100th decimal place). Record this mass in Data Table 5. 3. Calculate the mass of just the ice. (Round to the 100th decimal place). Record this mass in Data Table 5. 4. Calculate the density of the ice. (Round to the 100th decimal place). Record the density in Data Table 5. 5. Let the ice melt, then pour out the water from the cylinder. 6. Take off the label and dry out the cylinder.</p><p>Lab 5B – Properties of Seawater Page 6 EAR 203, 2013-2014 Read the following. In the Analysis portion of this lab, circle the answers to questions 22- 27. </p><p>22. This demonstrates clearly that human activity far from the ocean [(can)(cannot)] have dramatic effect on the ocean. A prime example of the interconnectedness of ocean, land, and impacts of human activity in the Earth system is the increase in number and intensity of such dead zones. Dead zones are ocean areas where dissolved oxygen in bottom and near-bot - tom waters declines to deadly proportions. Such areas of the seafloor with too little oxygen for most marine life are pro- duced when excess nutrients, especially nitrogen and phosphorus compounds, enter coastal surface waters and spur algal blooms. When the algae die, they sink to the seafloor. Their decomposition consumes the oxygen dissolved in the bottom waters, leaving a “hypoxic” (low oxygen) or “anoxic” (no oxygen) environment that is lethal to many marine species. </p><p>23. Figure 2 graphically depicts how the Gulf of Mexico dead zone forms. During the spring, relatively warm freshwater dis- charge from the Mississippi River system flows into the Gulf and floats over the [(more)(less)] dense seawater. This surface water layer acts as a barrier that prevents the replenishment of dissolved oxygen in the seawater from the overlying atmos - phere. </p><p>Figure 2. How the Dead Zone Forms. [The Times-Picayune, http://blog.nola.com/graphics/deadzone_how061007.gif ] </p><p>[Seeing it in motion: A Flash animation describing the nutrient process that leads to hypoxia in the northern waters of the Gulf of Mexico can be found at: http://www.gulfhypoxia.net/Overview/hypoxia_flash.asp.] </p><p>24. The nutrients, including those from fertilizer runoff and from sewage discharged into the Mississippi River system, ignite al - gal blooms in the warmed surface water subjected to the increasingly intense sunlight during spring that remains strong into summer. The dead algae then sink and begin to decompose as they settle through the underlying seawater to the ocean bottom. The decomposition process results in the [(loss)(gain)] of dissolved oxygen in the deeper seawater. </p><p>Dissolved oxygen is an essential ingredient for sustaining life in the marine food chain. With little or no oxygen, commercially important fish and shellfish (e.g., crabs and oysters) die or are driven from their habitat. The result is the</p><p>Lab 5B – Properties of Seawater Page 7 EAR 203, 2013-2014 creation of a dead zone in bottom and near-bottom waters. The Gulf of Mexico dead zone typically persists until late summer and into early autumn when passing storms, including hurricanes, and cooler temperatures act to stir and break up the layered water structure. </p><p>25. Figure 3 depicts the bottom-water dissolved oxygen (DO) concentration in milligrams per liter (mg/L) along the Gulf of Mex- ico coast extending from Louisiana to eastern Texas as observed 18-23 July 2009. The area shaded red essentially shows where bottom-water dissolved oxygen values were measured. The lighter the red shading, the lower the dissolved oxygen concentration. The dark isoline (line of constant value) in the figure surrounding the lighter red shadings has a value of 2 mg/L. Dead zones are generally defined as places where the dissolved oxygen concentration falls below 2 mg/L. Based on this criterion, the figure reveals the centers of [(1)(2)(3)] dead zones in the sample area. </p><p>The size of the 2009 low-oxygen Gulf of Mexico dead zone, as measured from the Louisiana Universities Marine Consor - tium’s research vessel Pelican by a group of scientists led by Dr. Nancy Rabalais, extended over 8,000 square kilometers (just over 3,000 square miles). The 2009 dead zone was surprisingly small, since the average over the past five years is 15,670 square kilometers (6,000 square miles). A number of factors determine the size of the dead zone (e.g., in July 2009 the flow of the Mississippi River fell below average). </p><p>Figure 4 shows the history of the size of the Gulf of Mexico dead zone since 1985. Included on the graph are horizontal dashed lines indicating the average size over the period of record, the 5-year running average (average of the most recent five years), and the goal size sought by some attempting to reduce the human impact on the aquatic system. </p><p>Lab 5B – Properties of Seawater Page 8 EAR 203, 2013-2014</p><p>26. Figure 4 shows that in 2009 the Gulf of Mexico experienced a dead zone that was about [(half)(the same as)(double)] the size of the average dead zone over the most recent five-year period. </p><p>27. Figure 4 shows that over the period of record, there has been considerable variability in the size of the mid-summer Gulf of Mexico dead zone. At the same time, it shows a general long-term trend toward [(lower)(steady)(higher)] annual maximum areas of mid-summer Gulf of Mexico dead zones. </p><p>The phenomenon of dead zones, an example of cultural eutrophication (accelerated process of nutrient and sediment con - centration in an aquatic system due to human activity), clearly evidence growing human impacts on the ocean environ- ment. It, along with other observational evidence, demonstrates that we live in and are part of an Earth system. It also shows that no matter where we live, our actions can impact all the sub-systems of the Earth, including the ocean. </p><p>To learn more about the National Research Council report, Nutrient Control Actions for Improving Water Quality in the Mississippi River Basin and Northern Gulf of Mexico, go to: http://www8.nationalacademies.org/onpinews/newsitem.aspx?RecordID=12544. To learn more about the 2009 Gulf of Mexico dead zone, go to the LUMCON Hypoxia Site: http://www.gulfhypoxia.net/ . </p><p>Lab 5B – Properties of Seawater Page 9 EAR 203, 2013-2014 Name: ______Date: ______Block: _____</p><p>EAR 203 EARTH SYSTEM SCIENCE SYRACUSE UNIVERSITY LAB 5B – PROPERTIES OF SEAWATER</p><p>Data Table 1: Composition of Seawater percent (%) of ppt (‰) ppm Salt Mass (grams) 200 g of 200 g of 200 g Sodium chloride (NaCl) 4.696 2.348 23.48 23, 480</p><p>Magnesium chloride (MgCl2) 0.996</p><p>Sodium sulfate (Na2SO4) 0.784</p><p>Calcium chloride (CaCl2) 0.220 Potassium chloride (KCl) 0.132</p><p>Sodium bicarbonate (NaHCO3) 0.038 Potassium bromide (KBr) 0.020</p><p>Data Table 2: Conductivity Conductivity of Sea Water (yes-strength or no) Conductivity of Distilled Water (yes-strength or no) Conductivity of Tap Water (yes-strength or no)</p><p>Data Table 3: Dissolved Oxygen and pH Dissolved Oxygen Sample Salinity (‰) pH (ppm) Distilled Water 0 Tap Water 0.1 Sea Water 35</p><p>Data Table 4: Chlorine, Hardness, Alkalinity and pH of Sea Water Test Sea water Tap Water Distilled Water Total Chlorine (ppm) Free Chlorine (ppm) Hardness (gpg) Alkalinity (ppm) pH</p><p>Lab 5B – Properties of Seawater Page 10 EAR 203, 2013-2014 Data Table 5: Density of Ice Distilled Water Tap Water Sea Water Day 1 Day 2 Day 1 Day 2 Day 1 Day 2</p><p>Mass of graduated cylinder (g)</p><p>Volume of water/ice (g/mL)</p><p>Mass of graduated cylinder + water/ice (g)</p><p>Mass of water/ice (g)</p><p>Density of water/ice (g/mL)</p><p>Data Table 6: Freshwater Density Change Time Temperature Water Height (minutes) (°C) (cm) 0 0.5 1.0 1.5 2.0 2.5 3.0 3.5 4.0 4.5 5.0 5.5 6.0 6.5 7.0 7.5 8.0 8.5 9.0 9.5 10.0 10.5 11.0 11.5 12.0</p><p>Lab 5B – Properties of Seawater Page 11 EAR 203, 2013-2014</p><p>Data Table 7: Density of Seawater Mass of Mass of Water + Volume of Water Density of Water Graduated Graduated Cylinder Mass of Water (g) (ml) (g/ml) Cylinder (g) (g)</p><p>Average Density (g/ml)</p><p>Graphic Analysis:</p><p>1. Use Excel to generate a graphic model (pie chart) of the percentage of total mass of dissolved salts. You used a total mass of 6.886 grams of salts to make your salt water. Label each salt in the key by analyzing the amounts of relative salt you added when making your salt water. 2. Using Excel, generate the following line graphs: a. Salinity (x-axis) vs. dissolved oxygen (y-axis) b. pH (x-axis) vs. dissolved oxygen (y-axis) 3. Using Excel, generate a line graph illustrating Freshwater Density Change. a. Temperature (x-axis) vs. Water Height (y-axis) 4. Using Excel, generate a line graph illustrating the Density of Seawater a. Volume (x-axis) vs. Mass (y-axis)</p><p>Lab 5B – Properties of Seawater Page 12 EAR 203, 2013-2014 Analysis Textbooks or internet resources may be used to answer the following questions. Please site sources utilizing in-text citations. </p><p>1. What are the six most abundant components (elements) dissolved in seawater? What is produced when the two most abundant elements are combined? </p><p>2. What are the primary sources for the materials that comprise the dissolved components of seawater? </p><p>3. Identify three resources obtained from the ocean.</p><p>4. Which type(s) of water conducted electricity? Explain why it did so.</p><p>5. Which type(s) of water did not conduct electricity? Explain why it did not.</p><p>6. Which of your samples tested contained the most dissolved oxygen?</p><p>7. Most aquariums have “bubblers” (aerator) in them to produce bubbles and add oxygen to the water. Explain why these are important to an aquatic ecosystem.</p><p>8. In addition to a bubbler (aerator), how else could dissolved oxygen be added to a fish tank? Explain. </p><p>Lab 5B – Properties of Seawater Page 13 EAR 203, 2013-2014</p><p>9. Define pH. Would you describe the pH of your sea water as acidic, neutral, or basic?</p><p>10. Describe how ocean pH could become more acidic due to climate change. </p><p>11. Did you observe a relationship between the pH and amount of dissolved oxygen? Explain.</p><p>12. What property of water “directly determines the solubility (amount that can be dissolved in the water) and biological availability (amount that can be utilized by aquatic life) of chemical constituents such as nutrients (phosphorus, nitrogen, and carbon) and heavy metals (lead, copper, cadmium, etc.)?” Explain.</p><p>13. Compare the pH of your sea water obtained using the pHydrion strip to the pH obtained using the 5-in-1 test strip. If there was a difference, conjecture why this may have occurred. </p><p>14. Define alkalinity. Compare the alkalinity of the sea water, tap water and distilled water. Which is best for organisms? Explain.</p><p>15. Analyze your 4 line graphs. What is the trend for each graph? Describe the relationships between your variables for each graph. </p><p>Lab 5B – Properties of Seawater Page 14 EAR 203, 2013-2014</p><p>16. What is the slope of your Density of Sea Water graph? How does this compare to your calculated average sea water density in Data Table 7?</p><p>17. The actual density of seawater is (d = 1.03 g/cm3) at 25 °C. a. Determine percent error for your data obtained by averaging densities. (show calculations)</p><p> b. Determine percent error for your data obtained by the slope of your graph. (show calculations) </p><p>18. Explain “hardness” as it refers to water quality. Discuss the effect water hardness has on an aquatic ecosystem. </p><p>19. Compare the total chlorine found in sea water, tap water and fresh water. Is chlorinated water beneficial to living organisms? Explain. </p><p>20. Why was a nail used in the density bottle experiment?</p><p>21. Analyze the density of frozen sea water, frozen tap water and frozen distilled water. </p><p>22. Human activity far from the ocean [(can)(cannot)] have dramatic effect on the ocean. 23. During the spring, relatively warm freshwater discharge from the Mississippi River system flows into the Gulf and floats over the [(more)(less)] dense seawater 24. The decomposition process results in the [(loss)(gain)] of dissolved oxygen in the deeper seawater. 25. Based on this criterion, the figure reveals the centers of [(1)(2)(3)] dead zones in the sample area. </p><p>Lab 5B – Properties of Seawater Page 15 EAR 203, 2013-2014 26. Figure 4 shows that in 2009 the Gulf of Mexico experienced a dead zone that was about [(half)(the same as)(double)] the size of the average dead zone over the most recent five-year period. 27. Figure 4 shows that over the period of record, there has been considerable variability in the size of the mid-summer Gulf of Mexico dead zone. At the same time, it shows a general long-term trend toward [(lower)(steady)(higher)] annual maximum areas of mid-summer Gulf of Mexico dead zones. </p><p>Lab 5B – Properties of Seawater Page 16</p>
Details
-
File Typepdf
-
Upload Time-
-
Content LanguagesEnglish
-
Upload UserAnonymous/Not logged-in
-
File Pages16 Page
-
File Size-