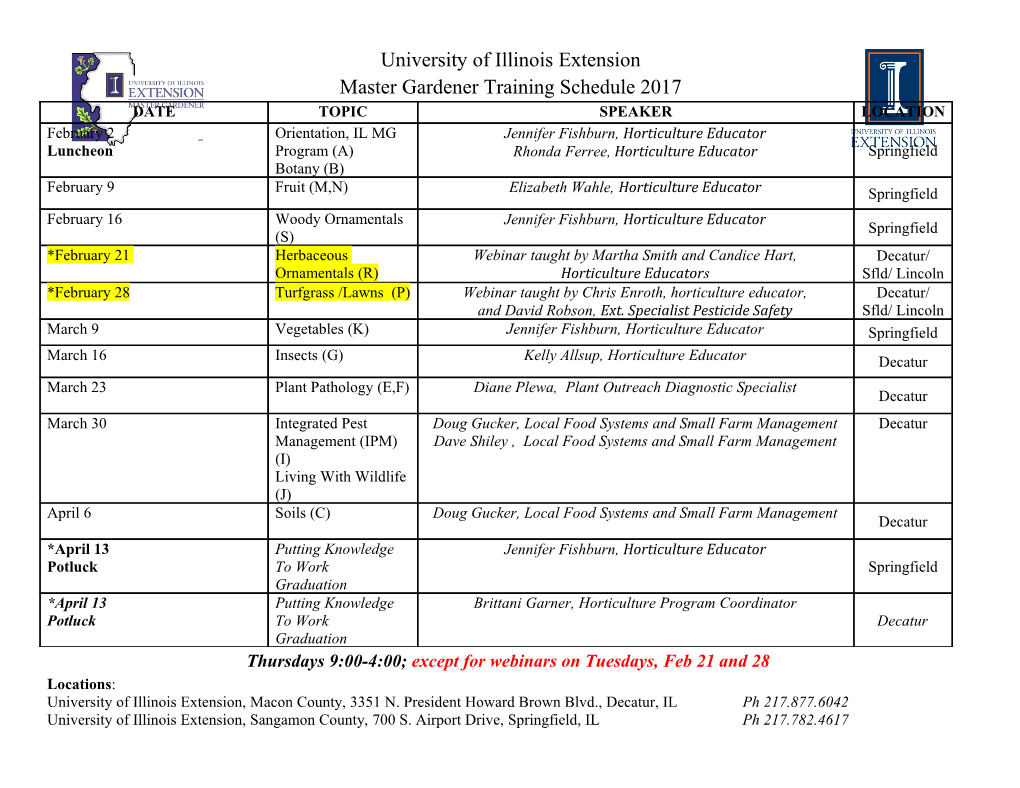
<p> 1Appendix A. Individual conceptual networks representing agricultural </p><p>2management and ecosystem services provision.</p><p>3</p><p>4 The relationships between agricultural management and eight ecosystem </p><p>5services (ES) provided by Pampean agroecosystems were represented in individual </p><p>6conceptual networks (Figs. 1 to 8). The eight conceptual networks developed in this </p><p>7work contained five types of nodes, and four types of logical links between nodes (see </p><p>8Section 2.3.). These logical links present capital letters in order to easily explain each </p><p>9conceptual network. </p><p>10</p><p>111. Supporting Service: Elements cycling - C Balance</p><p>12</p><p>13Fig. 1 Conceptual network representing functional relationships between agricultural management and </p><p>14provision of the Supporting service: Elements cycling - C balance. Capital letters represent the logical </p><p>15links between nodes. Legend: circles meaning input variables; rounded-squares meaning decision </p><p>16variables; squares meaning state variables; triangles meaning ecosystem processes and diamond meaning </p><p>17ecosystem service provision indicators. Tº: temperature, and Pp: rainfall </p><p>18</p><p>1 1 19 It is generally known that C inputs in soils consist of crop residues and roots, </p><p>20and sometimes additions of soil organic amendments; while C loss is caused by humus </p><p>21and residue mineralization, in conditions where soil erosion and C leaching are minimal </p><p>22(C leaching is not an important cause of soil organic carbon (SOC) losses in Pampean </p><p>23agroecosystems (Roberto Álvarez, personal communication)) (Oorts and others 2006). </p><p>24The interaction between temperature and rainfall regulates SOC through the influence </p><p>25of soil organic matter (SOM) mineralization (Fig. 1, Relations A and B) (Roberto </p><p>26Álvarez and Raúl Lavado, personal communication). High temperature reduces SOC </p><p>27because of intense SOM mineralization, while there is no linear answer for rainfall (Fig.</p><p>281, Relations A, B and F) (Álvarez and Lavado 1998). However, it is widely accepted </p><p>29that, in general, rainfall has the same effect as temperature (Fig. 1, Relations B and F) </p><p>30(Roberto Álvarez and Raúl Lavado, personal communication). Additionally, crop </p><p>31species, their growth rate and yield determine the amount and type (i.e., quality) of crop </p><p>32residue (including crop roots) (Fig. 1, Relations D and I) (Ernst and others 2002) which </p><p>33change SOC in surface soil layers, specially in no-tillage systems (Álvarez and Lavado </p><p>341998). Surface soil layers have greater C amounts because of the input of crop residue </p><p>35from harvested plants (Álvarez and Lavado 1998). Generally, legume species (e.g., </p><p>36soybean) have higher mineralization rates than gramineous species (e.g., wheat, maize) </p><p>37due to lower C/N relations (Fig. 1, Relation E) (Ernst and others 2002). </p><p>38 Another conditioning factor of SOC reduction is erosion vulnerability which is </p><p>39higher in continuous cropping systems, principally by 1) removing C from one site and </p><p>40depositing it elsewhere, and 2) promoting soil degradation and then reducing </p><p>41productivity (Fig. 1, Relation G) (Martínez-Mena and others 2008). However, it can be </p><p>42assumed that SOC movement is dependent on the topographic position (Haydée </p><p>43Steinbach and Roberto Álvarez, personal communication). Soils under no-tillage reduce</p><p>2 2 44both eolic erosion in semiarid sites, and hydric erosion in sites with great slopes </p><p>45(Monzon and others 2006).</p><p>46</p><p>472. Supporting Service: Elements cycling - N Balance</p><p>48</p><p>49Fig. 2 Conceptual network representing functional relationships between agricultural management and </p><p>50provision of the Supporting service: Elements cycling - N balance. Capital letters represent the logical </p><p>51links between nodes. Legend: circles meaning input variables; rounded-squares meaning decision </p><p>52variables; squares meaning state variables; triangles meaning ecosystem processes and diamond meaning </p><p>53ecosystem service provision indicator. Tº: temperature, and Pp: rainfall </p><p>54 55 Soil nitrogen (N) availability is modulated by four main factors: SOM </p><p>56mineralization, crop residue, fertilization regime and N losses (Cassman and others </p><p>572002). N mineralization through SOM is a very important supply source due to its usage</p><p>58availability (Fig. 2, Relation G), increasing or decreasing crop yield (Fig. 2, Relation J) </p><p>59(Bono and Álvarez 2007). The increase in soil moisture content increases mineralized N</p><p>60(Fig. 2, Relations B and F) (Helena Rimski-Korsakov, personal communication). This </p><p>61increase is a direct consequence of higher microbial activity, until the concentration of </p><p>3 3 62oxygen in the soil becomes a limitation for the microorganisms (Navarro and others </p><p>631991). Moreover, SOM is not only affected by mineralization but also by crop residue </p><p>64disposal on soil surface layers (Fig. 2, Relation E) (Ernst and others 2002), as it occurs </p><p>65for SOC in no- and reduced tillage systems. N fertilization can increase the amount of </p><p>66soil N pools which will be available for crops (Fig. 2, Relation K) (Abril and others </p><p>672007). However, N excedent can also be immobilized by microorganisms, resulting in a</p><p>68non linear effect (i.e., increase or reduce) of the application (Fig. 2, Relation K) </p><p>69(Cassman and others 2002; Portela and others 2006). Furthermore, N losses by </p><p>70denitrification, volatilization or leaching are the main causes for the low efficiency in </p><p>71the use of N, and therefore they affect available N in soil (Fig. 2, Relation L) (Abril and </p><p>72others 2007). Because of the low degree of these losses during the whole crop growth </p><p>73cycle (Álvarez and Grigera 2005), they can be grouped all together under the name of N</p><p>74losses (Roberto Álvarez, personal communication). </p><p>75</p><p>763. Supporting Service: Water cycling - Soil water balance</p><p>77</p><p>78Fig. 3 Conceptual network representing functional relationships between agricultural management and </p><p>79provision of the Supporting service: Water cycling - Soil water balance. Capital letters represent the </p><p>80logical links between nodes. Legend: circles meaning input variables; rounded-squares meaning decision </p><p>81variables; squares meaning state variables; triangles meaning ecosystem processes and diamonds meaning</p><p>82ecosystem service provision indicators. Tº: temperature, and Pp: rainfall </p><p>4 4 83 84 In Pampean agroecosystems, water supply for crops is determined by nine </p><p>85variables: 1) evaporation, 2) runoff, 3) soil structural stability, 4) soil texture, 5) aquifer </p><p>86depth, 6) soil depth, 7) presence of weeds/fallow/cover crops, 8) irrigation, and 9) </p><p>87rainfall (Fig. 3, Relations M, N, O, P, Q, R, S, T and C). These variables, in general, </p><p>88increase or affect water supply for crops. For instance, no-tillage systems leave crop </p><p>89residue on the soil surface and, therefore, soil evaporation is clearly decreased (Fig. 3, </p><p>90Relations E and F) (Monzon and others 2006). Relative soil evaporation rates directly </p><p>91influence the amount of soil water retained which will be used by the crop (Fig. 3, </p><p>92Relation M) (O´Leary and Connor 1997). Stubble mulch protects the surface soil from </p><p>93erosion and runoff, and increases water storage by minimising surface sealing and </p><p>94enhancing infiltration, as well as by directly reducing evaporation (Fig. 3, Relations G, </p><p>95J, N and O) (O´Leary and Connor 1997). Moreover, irrigation not only increases water </p><p>96supply for crops (Fig. 3, Relation T) but also affects runoff, depending on the amount of</p><p>97water irrigated and crop residue on soil surface (Fig. 3, Relation L) (Olga Heredia, </p><p>98personal communication). Systems under no-tillage can increase soil water </p><p>99accumulation during fallows (Fig. 3, Relation S), and thereby offer the potential for </p><p>100affecting crop yield in Pampean agroecosystems (Olga Heredia and Francisco Bedmar, </p><p>101personal communication) (Fig. 3, Relation U). </p><p>102 Soil depth is related with the ability of roots to explore soil profile and to absorb </p><p>103water stored there (Fig. 3, Relation R); on the other hand, aquifer depth can be defined </p><p>104by characterizing the average depth fluctuation of water table in different regions (Fig. </p><p>1053, Relation Q) (Esteban Jobbágy, personal communication). This is specially important </p><p>106in sandy soils (Claudia Sainato, personal communication). Finally, weeds can be burned</p><p>107to avoid evaporation as well as the establishment of cover crops (Fig. 3, Relation S) </p><p>108(Olga Heredia and Silvina Portela, personal communication). </p><p>5 5 109</p><p>1104. Supporting service: Soil conservation – Soil structural maintenance</p><p>111</p><p>112Fig. 4 Conceptual network representing functional relationships between agricultural management and </p><p>113provision of the Supporting service: Soil conservation – Soil structural maintenance. Capital letters </p><p>114represent the logical links between nodes. Legend: circles meaning input variables; rounded-squares </p><p>115meaning decision variables; squares meaning state variables; triangles meaning ecosystem processes and </p><p>116diamonds meaning ecosystem service provision indicators. Tº: temperature, and Pp: rainfall </p><p>117 118 Structural stability is defined as soil capacity to preserve the system of solids and</p><p>119pore space, when subjected to different external disturbances (e.g., tillage) (Taboada </p><p>120and Micucci 2002). Its loss is the critical factor which determines structural </p><p>121deterioration. This deterioration is evidenced by the formation of surface crusts, higher </p><p>122rates of runoff and soil loss due to erosion, as well as reduced water storage (Taboada </p><p>123and Micucci 2002). Soil structural stability is clearly affected by land use, which is in </p><p>124turn positively associated with crop residue, total organic C concentration and the forms</p><p>125of organic C (Fig. 4, Relations I and J) (Caravaca and others 2004). The close </p><p>126association found between structural stability, labile carbon and microbial biomass </p><p>6 6 127confirms both their importance in the mineralization process and their ability as </p><p>128aggregate cementitious (Fig. 4, Relations G and H) (Urricarriet and Lavado 1999). </p><p>129According to the first statement, SOM decomposition may be limited by pore size </p><p>130distribution due to the localization of SOM in pores inaccesible to microorganisms, a </p><p>131limited nutrient supply to microorganisms and restricted predation of those </p><p>132microorganisms (Miguel Taboada and Roberto Casas, personal communication). </p><p>133Furthermore, soil structural stability is one of the most important characteristics </p><p>134affecting crop yield (Fig. 4, Relation K) because it affects root penetration, water </p><p>135storage capacity, and air and water movement in soil (Fig. 4, Relation O) (Aparicio and </p><p>136Costa 2007). </p><p>137</p><p>1385. Regulating Service: Climate regulation – N2O emission control </p><p>139</p><p>140Fig. 5 Conceptual network representing functional relationships between agricultural management and </p><p>141provision of the Regulating service: Climate regulation – N2O emission control. Capital letters represent </p><p>142the logical links between nodes. Legend: circles meaning input variables; rounded-squares meaning </p><p>143decision variables; squares meaning state variables; triangles meaning ecosystem processes and diamonds</p><p>144meaning ecosystem service provision indicators. Tº: temperature, and Pp: rainfall </p><p>145</p><p>7 7 146 Although denitrification is only part of direct N2O emissions from soils, it is the </p><p>147most studied process in contrast with nitrification occurring in unsaturated soils, among </p><p>148other conditions (Fig. 5, Relation P) (Laura Yahdjian, personal communication). Thus, </p><p>149the main factors controlling denitrification are: soil pH, soil texture, nitrate </p><p>150concentration, C availability, aeration and moisture content (Guo and Zhou 2007). </p><p>151However, the major factors to consider, in terms of N2O production in Pampean </p><p>152agroecosystems, are available N in soil and moisture content (in this case, rainfall) (Fig. </p><p>1535, Relations N and O) (Palma and others 1997; Ciampitti and others 2005). For instance,</p><p>154it is known that the presence of actively growing plants limits the denitrification process</p><p>155in comparison with those treatments without plants, due to reduced water availability </p><p>156and to lower levels of nitrates in soil, to a lesser extent (Sainz Rozas and others 2004). </p><p>157Once the crop is harvested and crop residue remains on the surface, soluble C </p><p>158concentration is associated with denitrification (Fig. 5, Relation E); this is because </p><p>159bacteria biomass capable of denitrification is probably controlled primarily by C </p><p>160availability under aerobic conditions (Fig. 5, Relation M) (Miguel Taboada, personal </p><p>161communication), while emissions occur mainly during anaerobic conditions (Fig. 5, </p><p>162Relation O).</p><p>163</p><p>1646. Regulating Service: Water purification - Groundwater contamination control </p><p>165</p><p>8 8 166Fig. 6 Conceptual network representing functional relationships between agricultural management and </p><p>167provision of the Regulating service: Water purification - Groundwater contamination control. Capital </p><p>168letters represent the logical links between nodes. Legend: circles meaning input variables; rounded-</p><p>169squares meaning decision variables; squares meaning state variables; triangles meaning ecosystem </p><p>170processes and diamonds meaning ecosystem service provision indicators. Tº: temperature, and Pp: rainfall</p><p>171</p><p>172 Nitrate (NO3) leaching is one of the main causes for groundwater contamination </p><p>173(Fig. 6, Relations N and O) (Abril and others 2007; Claudia Sainato and Olga Heredia, </p><p>174personal communication). However, Mugni and others (2005) measured NO3 </p><p>175concentration in four Pampasic streams and concluded that it was relatively modest </p><p>176compared to intensively cultivated basins in Europe and North America. Consequently, </p><p>177there is a slow N enrichment of water resources in Pampean agroecosystems (Portela </p><p>178and others 2006). Water quality is reduced not only by N fertilization (Fig. 6, Relation </p><p>179J) (Rimski-Korsakov and others 2004; Abril and others 2007), but also SOM </p><p>180mineralization through several years removes great amounts of NO3 towards aquifers </p><p>181(Portela and others 2006; Helena Rimski-Korsakov and Raúl Lavado, personal </p><p>182communication) (Fig. 6, Relation G). N fertilization could also be indirectly inducing </p><p>183soil NO3 leaching, by altering the ability of plants root system to acquire N from soil </p><p>184and net mineralization rate from organic N pools (Cassman and others 2002). </p><p>185Furthermore, fertilization in excess of crop requirements or water excedent, such as </p><p>186rainfall events (Fig. 6, Relation M) or irrigation (Fig. 6, Relation K), increase the </p><p>187probability of soil NO3 leaching (Costa and others 2002; Rimski-Korsakov and others </p><p>1882004; Vergé and others 2007). It is important to clarify that the Pampa region has low N</p><p>189inputs through rainfall (Portela and others 2006). Other factors affecting soil NO3 </p><p>190leaching are particle size distribution, soil porosity and the ocurrence of preferential </p><p>191flow paths. These causes can be grouped under soil texture, which is another important </p><p>9 9 192factor because of its ability for retaining water (Fig. 6, Relation L) (Taboada and </p><p>193Micucci 2002). </p><p>194</p><p>1957. Regulating Service: Regulation of biotic adversities</p><p>196</p><p>197Fig. 7 Conceptual network representing functional relationships between agricultural management and </p><p>198provision of the Regulating service: Regulation of biotic adversities. Capital letters represent the logical </p><p>199links between nodes. Legend: circles meaning input variables; rounded-squares meaning decision </p><p>200variables; squares meaning state variables; triangles meaning ecosystem processes and diamonds meaning</p><p>201ecosystem service provision indicators </p><p>202</p><p>203 In Pampean agroecosystems, crop environment is determined by: 1) tillage </p><p>204system, 2) crop protection, 3) sowing density, 4) sowing date, 5) fertilization, 6) </p><p>205genotype selection, and 7) irrigation (Fig. 7, Relations B, A, C, D, E, F and G). These </p><p>206variables affect not only crop yield but also species composition and abundance of plant</p><p>207and animal community, and beneficial species (Fig. 7, Relations H, J and L) (Emilio </p><p>208Satorre and Elba De la Fuente, personal communication). Beneficial species as well as </p><p>209crop environment and crop changes affect species composition and </p><p>210abundance/incidence of pests, diseases and weeds (Fig. 7, Relations K, L and N). The </p><p>211latter reduces crop yield and affects natural pest mitigation of ecosystems (Fig. 7, </p><p>10 10 212Relations I and O). The presence of weeds influences the presence of diseases and </p><p>213diversity and abundance of two insect types: pests, with negative consequences for </p><p>214cropping systems, and their natural enemies (Altieri 1999). Generally, a high density of </p><p>215weeds is counter-productive because they reduce crop yield and its quality (Albrecht </p><p>2162003). </p><p>217</p><p>2188. Biodiversity maintenance</p><p>219</p><p>220Fig. 8 Conceptual network representing functional relationships between agricultural management and </p><p>221Biodiversity maintenance. Capital letters represent the logical links between nodes. Legend: circles </p><p>222meaning input variables; rounded-squares meaning decision variables; squares meaning state variables; </p><p>223triangles meaning ecosystem processes and diamond meaning ecosystem service provision indicator </p><p>224</p><p>225 Biological diversity or biodiversity is defined as the wide variety of plants, </p><p>226animals, microorganisms and their genetic variations (Altieri 1999). In agroecosystems, </p><p>227the variety of crops are also considered as biodiversity components (María Elena </p><p>228Zaccagnini, personal communication). However, the type and abundance of biodiversity</p><p>229may differ across agroecosystems in relation to their crop protection (i.e., </p><p>11 11 230phytotherapics application) and tillage system (Fig. 8, Relations A, B and C) (María </p><p>231Elena Zaccagnini, personal communication). </p><p>232 Other management strategies for increasing biodiversity involve the 1) </p><p>233manipulation of undisturbed areas within agroecosystems, 2) preservation of weeds, or </p><p>2343) introduction of mixtures containing grasses, legumes, flowering and/or aromatic </p><p>235plants. These strategies offer alternative food sources (i.e., pollen, nectar) to different </p><p>236organisms, and places for hibernation and reproduction (Fig. 8, Relation D) (Carmona </p><p>237and Landis 1999; Carmona and others 1999; Landis and others 2000). Furthermore, </p><p>238areas functioning as shelters act as biological corridors in the mobility of natural </p><p>239enemies (decreasing, in some cases, phytotherapic application) and their non-</p><p>240fragmentation is fundamental to the establishment of these organisms and the rapid </p><p>241recolonization of agroecosystems after a disturbance (Fig. 8, Relation D) (Carmona and </p><p>242Landis 1999; Landis and others 2000; Jonsson and others 2008; Gardiner and others </p><p>2432009; Rufus and others 2009). </p><p>244 Sequences, rotations and landscape structure provide two types of </p><p>245agroecosystems heterogeneity (Fig. 8, Relations E and F). On the one hand, </p><p>246sequences/rotations are recognized as temporal heterogeneity even though they are </p><p>247planned biodiversity (e.g., crops, pastures); on the other hand, landscape structure can </p><p>248be identified as spatial heterogeneity (Elba De la Fuente, personal communication). </p><p>249Landscape structure plays a crucial role in the survival of species by offering different </p><p>250kinds of habitat (Claudio Ghersa, personal communication). </p><p>251</p><p>252References</p><p>12 12 253Abril A, Baleani D, Casado-Murillo N, Noe L (2007) Effect of wheat crop fertilization</p><p>254 on nitrogen dynamics and balance in the Humid Pampas, Argentina. Agriculture,</p><p>255 Ecosystems & Environment 119:171-176. </p><p>256Albrecht H (2003) Suitability of arable weeds as indicator organisms to evaluate species</p><p>257 conservation effects of management in agricultural ecosystems. Agriculture,</p><p>258 Ecosystems & Environment 98:201-211.</p><p>259Altieri MA (1999) The ecological role of biodiversity in agroecosystems. Agriculture,</p><p>260 Ecosystems & Environment 74:19-31.</p><p>261Álvarez R, Grigera S (2005) Analysis of soil fertility and management effects on yields</p><p>262 of wheat and corn in the Rolling Pampa of Argentina. Journal of Agronomy & Crop</p><p>263 Science 191:321-329. </p><p>264Álvarez R, Lavado RS (1998) Climate, organic matter and clay content relationships in</p><p>265 the Pampa and Chaco soils, Argentina. Geoderma 83:127-141.</p><p>266Aparicio V, Costa JL (2007) Soil quality indicators under continuous cropping systems</p><p>267 in the Argentinean Pampas. Soil & Tillage Research 96:155-165.</p><p>268Bono A, Álvarez R (2007) Mineralización de nitrógeno del suelo en la región semiárida</p><p>269 pampeana. Instituto Nacional de Tecnología Agropecuaria, Publicación Técnica Nº</p><p>270 69:65-76. </p><p>271Caravaca F, Lax A, Albaladejo J (2004) Aggregate stability and carbon characteristics</p><p>272 of particle-size fractions in cultivated and forested soils of semiarid Spain. Soil &</p><p>273 Tillage Research 78:83-90.</p><p>274Carmona D, Landis D (1999) Influence of refuge habitats and cover crops on seasonal</p><p>275 activity-density of ground beetles (Coleoptera: Carabidae) in field crops.</p><p>276 Environmental Entomology 28:1145-1153.</p><p>13 13 277Carmona D, Menalled F, Landis D (1999) Northern Field Cricket Gryllus pensylvanicus</p><p>278 Burmeister (Orthoptera: Gryllidae): Weed seed predation and within field activity-</p><p>279 density. Journal Economic of Entomology 92:825-829.</p><p>280Cassman KG, Dobermann A, Walters DT (2002) Agroecosystems, nitrogen-use</p><p>281 efficiency, and nitrogen management. Ambio 31:132-140. </p><p>282Ciampitti IA, Ciarlo EA, Conti ME (2005) Emisiones de óxido nitroso en un cultivo de</p><p>283 soja (Glycine max (L.) Merrill): efecto de la inoculación y de la fertilización</p><p>284 nitrogenada. Ciencia del Suelo 23:123-131. </p><p>285Costa JL, Massone H, Martínez D, Suero EE, Vidal CM, Bedmar F (2002) Nitrate</p><p>286 contamination of a rural aquifer and accumulation in the unsaturated zone.</p><p>287 Agricultural Water Management 57:33-47. </p><p>288Ernst O, Betancur O, Borges R (2002) Descomposición de rastrojos de cultivos en</p><p>289 siembra sin laboreo: trigo, maíz, soja y trigo después de maíz o de soja. Agrociencia</p><p>290 6:20-26.</p><p>291Gardiner MM, Landis DA, Gratton C, Difonzo C, O´Neal M, Chacon JM, Wayo MT,</p><p>292 Schmidt NP, Mueller EE, Heimpel,GE (2009) Landscape diversity enhances</p><p>293 biological control of an introduced crop pest in the north-central USA. Ecological</p><p>294 Applications 19:143-154. </p><p>295Guo J, Zhou C (2007) Greenhouse gas emissions and mitigation measures in Chinese</p><p>296 agroecosystems. Agricultural and Forest Meteorology 142:270-277. </p><p>297Jonsson M, Wratten SD, Landis DA, Gurr GM (2008) Recent advances in conservation</p><p>298 biological controls of arthropods by arthropods. Biological Control 45:172-175.</p><p>299Landis D, Menalled FD, Lee J, Carmona DM, Perez Valdez A (2000) Habitat</p><p>300 management to enhance biological control in IPM. In: Kenedy GG, Sutton TB (eds)</p><p>14 14 301 Emerging technologies for Integrated Pest Management: Concepts, Research and</p><p>302 Implementation. APS PRESS. St. Paul, Minesota, pp. 226-239. </p><p>303Martínez-Mena M, Lopez J, Almagro M, Boix-Fayos C, Albaladejo J (2008) Effect of</p><p>304 water erosion and cultivation on the soil carbon stock in a semiarid area of South-</p><p>305 East Spain. Soil & Tillage Research 99:119-129. </p><p>306Monzon JP, Sadras VO, Andrade FH (2006) Fallow soil evaporation and water storage</p><p>307 as affected by stubble in sub-humid (Argentina) and semi-arid (Australia)</p><p>308 environments. Field Crops Research 98:83-90.</p><p>309Mugni H, Jergentz S, Schultz R, Maine A, Bonetto C (2005) Phosphate and nitrogen</p><p>310 compounds in streams of Pampean Plain areas under intensive cultivation (Buenos</p><p>311 Aires, Argentina). In: Serrano L, Golterman HL (eds) Proceedings of the 4th</p><p>312 International Symposium Phosphates in Sediments. Backhuys Publishers, The</p><p>313 Netherlands, pp. 163-170. </p><p>314Navarro C, Echeverría H, Fonalleras M, Manavella F (1991) Efecto de los contenidos</p><p>315 de humedad sobre la mineralización del nitrógeno en suelos del sudeste bonaerense.</p><p>316 Ciencia del Suelo 9:13-19. </p><p>317O´Leary GJ, Connor DJ (1997) Stubble retention and tillage in a semi-arid environment:</p><p>318 1. Soil water accumulation during fallow. Field Crops Research 52:209-219.</p><p>319Oorts K, Nicolardot B, Merckx R, Richard G, Boizard H (2006) C and N mineralization</p><p>320 of undisrupted and disrupted soil from different structural zones of conventional</p><p>321 tillage and no-tillage systems in northern France. Soil Biology & Biochemistry</p><p>322 38:2576-2586. </p><p>323Palma RM, Rímolo M, Saubidet MI, Conti ME (1997) Influence of tillage system on</p><p>324 denitrification in maize-cropped soils. Biology and Fertility of Soils 25:142-146. </p><p>15 15 325Portela SI, Andriulo AE, Sasal MC, Mary B, Jobbágy EG (2006) Fertilizer vs. organic</p><p>326 matter contributions to nitrogen leaching in cropping systems of the Pampas: 15N</p><p>327 application in field lysimeters. Plant Soil 289:265-277.</p><p>328Rimski-Korsakov H, Rubio G, Lavado RS (2004) Potential nitrate losses under different</p><p>329 agricultural practices in the pampas region, Argentina. Agricultural Water</p><p>330 Management 65:83-94. </p><p>331Rufus I, Tuell J, Fiedler A, Gardiner M, Landis D (2009) Maximizing arthropod-</p><p>332 mediated ecosystem services in agricultural landscapes: the role of native plants.</p><p>333 Frontiers in Ecology and the Environment 7:196-203.</p><p>334Sainz Rozas H, Echeverría HE, Barbieri P (2004) Denitrification in a soil under no-</p><p>335 tillage as a function of presence of maize plant and nitrogen rate. Ciencia del Suelo</p><p>336 22:27-35.</p><p>337Taboada MA, Micucci FG (2002) Fertilidad física de los suelos. Editorial Facultad</p><p>338 Agronomía, Universidad de Buenos Aires. Buenos Aires, Argentina.</p><p>339Urricarriet S, Lavado RS (1999) Indicadores de deterioro en suelos de la Pampa</p><p>340 Ondulada. Ciencia del Suelo 17:37-44.</p><p>341Vergé XPC, De Kimpe C, Desjardins RL (2007) Agricultural production, greenhouse</p><p>342 gas emissions and mitigation potential. Agricultural and Forest Meteorology</p><p>343 142:255-269. </p><p>16 16</p>
Details
-
File Typepdf
-
Upload Time-
-
Content LanguagesEnglish
-
Upload UserAnonymous/Not logged-in
-
File Pages16 Page
-
File Size-