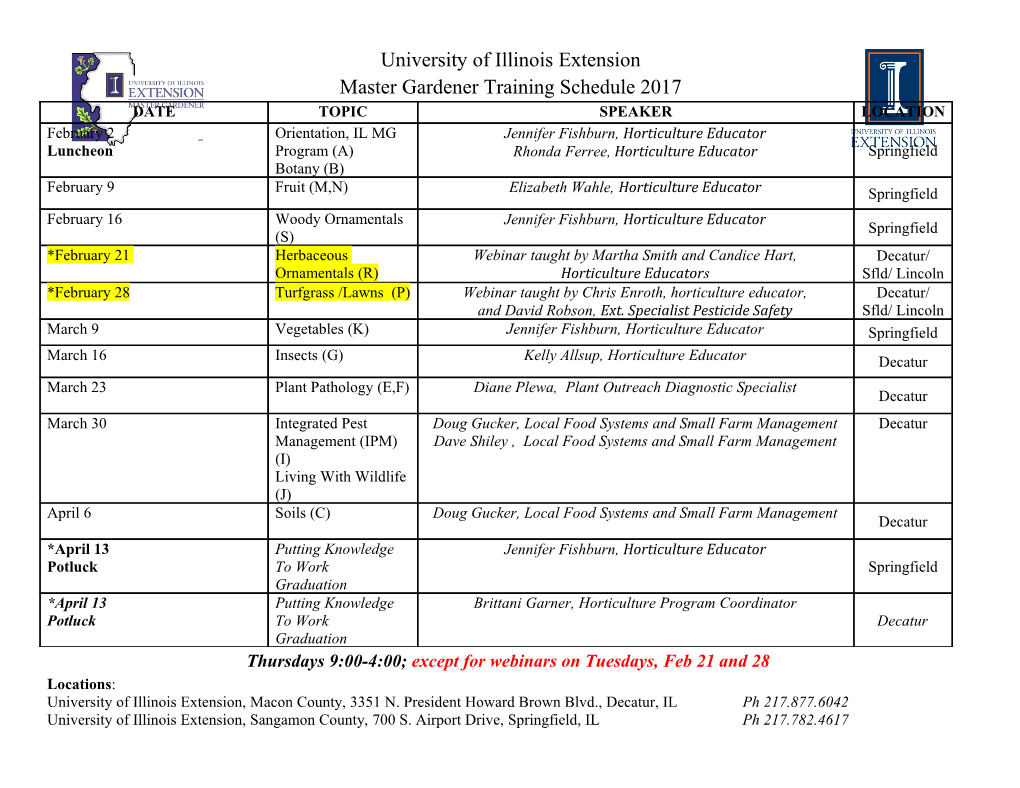
Math book From Wikipedia PDF generated using the open source mwlib toolkit. See http://code.pediapress.com/ for more information. PDF generated at: Mon, 25 Jul 2011 10:39:12 UTC Contents Articles 0.999... 1 1 (number) 20 Portal:Mathematics 24 Signed zero 29 Integer 32 Real number 36 References Article Sources and Contributors 44 Image Sources, Licenses and Contributors 46 Article Licenses License 48 0.999... 1 0.999... In mathematics, the repeating decimal 0.999... (which may also be written as 0.9, , 0.(9), or as 0. followed by any number of 9s in the repeating decimal) denotes a real number that can be shown to be the number one. In other words, the symbols 0.999... and 1 represent the same number. Proofs of this equality have been formulated with varying degrees of mathematical rigour, taking into account preferred development of the real numbers, background assumptions, historical context, and target audience. That certain real numbers can be represented by more than one digit string is not limited to the decimal system. The same phenomenon occurs in all integer bases, and mathematicians have also quantified the ways of writing 1 in non-integer bases. Nor is this phenomenon unique to 1: every nonzero, terminating decimal has a twin with trailing 9s, such as 8.32 and 8.31999... The terminating decimal is simpler and is almost always the preferred representation, contributing to a misconception that it is the only representation. The non-terminating form is more convenient for understanding the decimal expansions of certain fractions and, in base three, for the structure of the ternary Cantor set, a simple fractal. The non-unique form must be taken into account in a classic proof of the uncountability of the entire set of real numbers. Even more generally, any positional numeral system for the real numbers contains infinitely many numbers with multiple representations. The equality 0.999... = 1 has long been accepted by mathematicians and taught in textbooks to students. In the last few decades, researchers of mathematics education have studied the reception of this equality among students, many of whom initially question or reject it. Many are persuaded by an appeal to authority from textbooks and teachers, or by arithmetic reasoning as below to accept that the two are equal. However, some are often uneasy enough that they seek further justification. The equality of 0.999... and 1 is closely related to the absence of nonzero infinitesimal real numbers. Some alternative number systems, such as the hyperreals, do contain nonzero infinitesimals. In these systems, unlike in the reals, there can be numbers whose difference from 1 is less than any rational number. Other systems, known as the p-adic numbers, have a different form of "decimal expansions" which behave quite differently than expansions of real numbers. Although the real numbers are the most common object of study in the field of mathematical analysis, the hyperreals and p-adics both have applications in that area. Algebraic proofs Algebraic proofs showing that 0.999... represents the number 1 use concepts such as fractions, long division, and digit manipulation to build transformations preserving equality from 0.999... to 1. Fractions and long division One reason that infinite decimals are a necessary extension of finite decimals is to represent fractions. Using long division, a simple division of integers like 1⁄ becomes a recurring decimal, 0.111..., in which the digits repeat 9 without end. This decimal yields a quick proof for 0.999... = 1. Multiplication of 9 times 1 produces 9 in each digit, so 9 × 0.111... equals 0.999... and 9 × 1⁄ equals 1, so 0.999... = 1: 9 0.999... 2 Another form of this proof multiplies 1⁄ = 0.333... by 3. 3 Digit manipulation When a number in decimal notation is multiplied by 10, the digits do not change but each digit moves one place to the left. Thus 10 × 0.999... equals 9.999..., which is 9 greater than the original number. To see this, consider that in subtracting 0.999... from 9.999..., each of the digits after the decimal separator cancels, i.e. the result is 9 − 9 = 0 for each such digit. The final step uses algebra: Discussion Although these proofs demonstrate that 0.999... = 1, the extent to which they explain the equation depends on the audience. In introductory arithmetic, such proofs help explain why we have 0.999... = 1 but 0.333... < 0.4. And in introductory algebra, the proofs help explain why the general method of converting between fractions and repeating decimals works. But the proofs shed little light on the fundamental relationship between decimals and the numbers they represent, which underlies the question of how two different decimals can be said to be equal at all.[1] William Byers argues that a student who agrees that 0.999... = 1 because of the above proofs, but hasn't resolved the ambiguity, doesn't really understand the equation.[2] Fred Richman argues that the first argument "gets its force from the fact that most people have been indoctrinated to accept the first equation without thinking".[3] Once a representation scheme is defined, it can be used to justify the rules of decimal arithmetic used in the above proofs. Moreover, one can directly demonstrate that the decimals 0.999... and 1.000... both represent the same real number; it is built into the definition. This is done below. Analytic proofs Since the question of 0.999... does not affect the formal development of mathematics, it can be postponed until one proves the standard theorems of real analysis. One requirement is to characterize real numbers that can be written in decimal notation, consisting of an optional sign, a finite sequence of any number of digits forming an integer part, a decimal separator, and a sequence of digits forming a fractional part. For the purpose of discussing 0.999..., the integer part can be summarized as b and one can neglect negatives, so a decimal expansion has the form 0 It is vital that the fraction part, unlike the integer part, is not limited to a finite number of digits. This is a positional notation, so for example the 5 in 500 contributes ten times as much as the 5 in 50, and the 5 in 0.05 contributes one tenth as much as the 5 in 0.5. 0.999... 3 Infinite series and sequences Further information: Decimal representation Perhaps the most common development of decimal expansions is to define them as sums of infinite series. In general: For 0.999... one can apply the convergence theorem concerning geometric series:[4] If then Since 0.999... is such a sum with a common ratio r = 1⁄ , the theorem makes short work of the question: 10 This proof (actually, that 10 equals 9.999...) appears as early as 1770 in Leonhard Euler's Elements of Algebra.[5] The sum of a geometric series is itself a result even older than Euler. A typical 18th-century derivation used a term-by-term manipulation similar to the algebraic proof given above, and as late as 1811, Bonnycastle's textbook An Introduction to Algebra uses such an argument for geometric series to justify the same [6] maneuver on 0.999... A 19th-century reaction against such Limits: The unit interval, including the base-4 fraction liberal summation methods resulted in the definition that still sequence (.3, .33, .333, ...) converging to 1. dominates today: the sum of a series is defined to be the limit of the sequence of its partial sums. A corresponding proof of the theorem explicitly computes that sequence; it can be found in any proof-based introduction to calculus or analysis.[7] A sequence (x , x , x , ...) has a limit x if the distance |x − x | becomes arbitrarily small as n increases. The statement 0 1 2 n that 0.999... = 1 can itself be interpreted and proven as a limit:[8] The last step, that 1⁄ n → 0 as n → ∞, is often justified by the axiom that the real numbers have the Archimedean 10 property. This limit-based attitude towards 0.999... is often put in more evocative but less precise terms. For example, the 1846 textbook The University Arithmetic explains, ".999 +, continued to infinity = 1, because every annexation of a 9 brings the value closer to 1"; the 1895 Arithmetic for Schools says, "...when a large number of 9s is taken, the difference between 1 and .99999... becomes inconceivably small".[9] Such heuristics are often interpreted by students as implying that 0.999... itself is less than 1. 0.999... 4 Nested intervals and least upper bounds Further information: Nested intervals The series definition above is a simple way to define the real number named by a decimal expansion. A complementary approach is tailored to the opposite process: for a given real number, define the decimal expansion(s) to name it. If a real number x is known to lie in the closed interval [0, 10] (i.e., it is greater than or equal to 0 and less than or equal to 10), one can imagine dividing that interval into ten pieces that overlap only at their endpoints: [0, 1], [1, 2], [2, 3], and so on up to [9, 10]. The number x must belong to one of these; if it belongs to [2, 3] then one records the digit "2" and subdivides that interval into [2, 2.1], [2.1, 2.2], ..., [2.8, 2.9], [2.9, 3]. Continuing this process yields an infinite sequence of nested intervals, labeled by an infinite sequence of Nested intervals: in base 3, 1 = 1.000..
Details
-
File Typepdf
-
Upload Time-
-
Content LanguagesEnglish
-
Upload UserAnonymous/Not logged-in
-
File Pages50 Page
-
File Size-