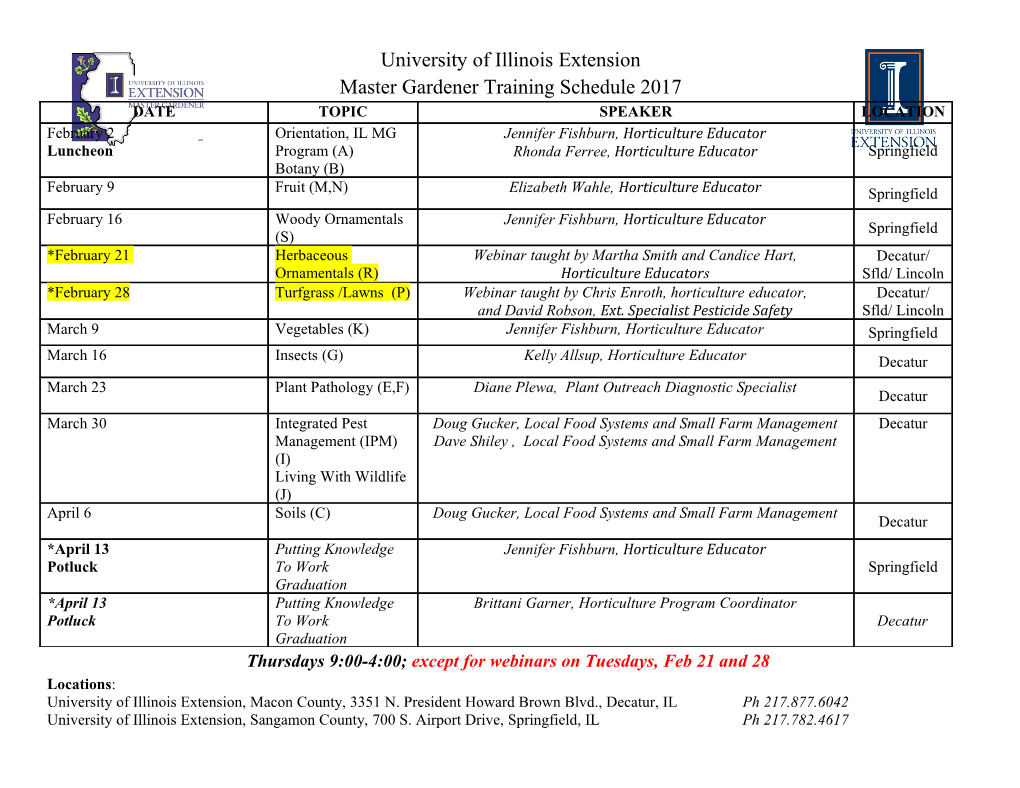
<p> HIGH VOLTAGE PULSE TESTING OF ATTENUATORS AND OTHER COMPONENTS OF THE ANITA GROUND STATION PULSER </p><p>Jacob Ribnik, Mark Harrison and David Saltzberg (UCLA)</p><p>This document describes the process by which we stress test Mini-Circuits and other attenuators to determine their suitability for the ANITA ground validation pulser. The motivation behind these tests is financial: if the Mini-Circuits attenuators can withstand thousands of near instantaneous (<5 nanosecond) high-voltage (up to 4 kV) pulses while preserving the quality of the signal, then they can be purchased and used in the ground validation for nearly twenty dollars each rather than require custom HV attenuators for nearly two thousand dollars each. We have purchased one such HV attenuator from Barth. Ideally these tests are performed on new out-of-the-box attenuators, but as the only 20dB N-type Mini-Circuits attenuators available to me have already been exposed to an unknown albeit relatively small number of HV pulses, We will describe the process by which we test these and present evidence for their suitability in the ground validation system</p><p>The process is simple and recommended by Barth engineers. We measure the input resistances of both attenuator ports with a laboratory ohmmeter, then send thousands of HV pulses through either port of the attenuator and re-measure the resistances of both ports. If the new values are markedly different from the original values (how much?) the attenuator has likely been damaged by the HV pulses. In addition to the resistance checks, scope traces of the HV pulses are taken before and after pulsing to check for signal quality. This test would ideally be performed with a spectrum analyzer by scanning the entire range of desired experimental frequencies and searching for impurities in the Fourier transforms of the signals through the attenuator. However, we are currently only concerned with preserving the quality of the HV impulses created by the 4kV Pockel's cell driver, and will visually inspect the before and after scope traces of these signals to determine quality equivalence or loss.</p><p>The 20dB N-type Mini-Circuits attenuator we are testing has been exposed to no more than 250 HV pulses. It is difficult to determine after this many pulses whether the success of the attenuator is due to conditioning by the aforementioned pulsing or just the high quality of its initial state; or whether it can be further conditioned by pulsing from its current state. This can likely be determined for a new attenuator by first pulsing each port ten times or so and comparing the signal quality and resistances to those in its initial state, and then increasing the number of pulses to one hundred and again comparing these values to the initial values. Fortunately, we do have the initial resistance values and scope trace for this particular 20dB attenuator. We will begin by checking its current resistances and signal quality, and proceed directly to bombardment by one thousand 4kV pulses at 1Hz.</p><p>We first determine the internal resistance of the ohmmeter by connecting its leads and measuring resistance. The internal resistance of the ohmmeter is 0.2 ohms. Next we establish a reference load (RL) that will be used for all subsequent resistance measurements of the attenuator. The RL is not used to terminate the attenuator during pulsing because its properties can likewise be affected by pulsing, and could result in inaccurate resistance measurements of the attenuator. The RL is an N-type 50 ohm terminator with a measured resistance of 50.1 ohms. We now measure the current resistances of both ports on the attenuator with the RL on the opposite port: the male side has a resistance of 49.6 ohms, and the female side has a resistance of 49.4 ohms. The male side was measured through an N-type fem-fem barrel as this barrel will be required in every connection. The resistance of the barrel is 0.1 ohms. As mentioned, We are lucky to have the initial resistance values of the attenuator before any HV pulsing: the male side was 50.3 ohms and the female side was 50.3 ohms. These values were ascertained using the same ohmmeter and RL. We are not yet concerned with the obvious fluctuations in resistance after pulsing because the attenuator may be in a state of conditioning, and as seen from the 4kV impulse scope trace images of the initial and current states of the attenuator, the signal quality has been well preserved.</p><p>Figure 1 Initial state before HV-pulsing Figure 2 Current state after < 250 HV pulses</p><p>Time to pulse; we attach an independent N-type 50 ohm terminator to the female port of the attenuator, and connect the male side through the N barrel directly to the 4kV impulse generator. I trigger the impulse generator at a frequency of 1Hz, and allow 1000 pulses through the attenuator. After pulsing, we measure the resistances of both attenuator ports while being sure to attach the RL to the opposite side. The male side now reads 51.5 ohms and the female side reads 49.6 ohms. Despite the fluctuations in resistance, the scope trace still shows preservation of quality for the 4kV impulse. Figure 3 After 1000 pulses through the male port</p><p>We now attach the female side of the attenuator directly to the impulse generator with the independent 50 ohm terminator on the male end, and allow 1000 pulses through it. Using the RL, the male side has a resistance of 51.5 ohms and the female side has a resistance of 51.0 ohms. The scope trace shows a high quality impulse. Figure 4 After another 1000 pulses through female port</p><p>After another 1000 pulses through the male end of the attenuator, the male side has a resistance of 51.7 ohms and the female side has a resistance of 51.0 ohms. Again the scope trace shows no loss in quality from the initial state of the attenuator.</p><p>Figure 5 After yet another 1000 pulses through male port Lastly, we allow another 1000 4kV pulses through the female end of the attenuator, and measure the resistances using the RL. The male side measures 51.8 ohms and the female side measures 51.2 ohms. Again though, the scope trace is marvelous.</p><p>Figure 6 Lastly, after another 1000 pulses through female port</p><p>While it is hard to determine the effectiveness of the DC resistance tests in determining the integrity of the 20dB Mini-Circuits attenuator, the scope traces reveal no loss in quality of the incident 4kV impulse from the initial attenuator state. There are many things to note: First, this line of testing was proposed by the Barth attenuator company to be performed on their two thousand dollar attenuators and not the twenty dollar Mini-Circuits attenuators. The procedure claims that an increase in resistance over 0.1 ohms means the attenuator is damaged. Of course, the Mini-Circuits attenuator resistances fluctuated by many more times this number of ohms. If the procedure is demonstrated effective for attenuators in general, then it should be reapplied with a 4- wire ohmmeter for better resistance resolution, and the attenuator should be exposed to many thousands more impulses than I have just now allowed. Perhaps an AC-voltage test would be better suited, or an impedance test rather than a resistance test. * * *</p><p>Since the attenuator stress testing revealed no problems with sending kilovolt pulses through cheaper equipment, we tested several other components of the ANITA ground pulser in a similar manner. Six- and twelve-inch cable assemblies, screw connector adapters (N-female to SMA-female), switches, N-type elbow joints, and N- type right-angle jacks were all subjected to a series of 10, 100, and 1000 4-kilovolt pulses at a rate of one per second, before and after which their impedance was measured using a multimeter while each component was connected to a 50-ohm terminator. During the pulsing, an oscilloscope was used to monitor the form of the pulse after passing through each component.</p><p>The following tables chart the results of all this pulsing:</p><p>6” SMA Cable assembly No. of Pulses Impedance (Ω) 0 50.2 10 50.2 100 50.2 1000 50.3</p><p>12” SMA Cable assembly #1 No. of Pulses Impedance (Ω) 0 50.4 10 50.4 100 50.5 1000 50.4</p><p>#2 No. of Pulses Impedence (Ω) 0 50.3 10 50.3 100 50.4 1000 50.4</p><p>Screw connector adapter (SMA-female to N-female) #1 No. of Pulses Impedence (Ω) 0 50.3 10 50.4 100 50.4 1000 50.2 #2 No. of Pulses Impedence (Ω) 0 50.2 10 50.3 100 50.4 1000 50.3</p><p>Switches #1 Side 1 Side 2 No. of Pulses Impedance (Ω) No. of Pulses Impedance (Ω) 0 50.4 0 50.5 10 50.5 10 50.6 100 50.6 100 50.5 1000 50.7 1000 50.6</p><p>#2 Side 1 Side 2 No. of Pulses Impedance (Ω) No. of Pulses Impedance (Ω) 0 50.1 0 50.6 10 50.4 10 50.6 100 50.5 100 50.7 1000 50.7 1000 50.7</p><p>N-Type Elbow Joints #1 No. of Pulses Impedance (Ω) 0 50.2 10 50.3 100 50.4 1000 50.3</p><p>#2 No. of Pulses Impedance (Ω) 0 50.3 10 50.4 100 50.4 1000 50.5 N-Type Right Angle Jacks #1 No. of Pulses Impedance (Ω) 0 50.5 10 50.5 100 50.5 1000 50.5</p><p>#2 No. of Pulses Impedance (Ω) 0 50.6 10 ----- 100 50.6 1000 50.4</p><p>During all of these tests, we did not observe a change in the shape of the pulses on the oscilloscope. Furthermore, despite seeing an overall increase in the impedance of each component, this can be attributed to the error in the digital multimeter. These readings would often change by as much as 0.5Ω depending on how hard, at what angle I held the probes to the various connectors.</p>
Details
-
File Typepdf
-
Upload Time-
-
Content LanguagesEnglish
-
Upload UserAnonymous/Not logged-in
-
File Pages9 Page
-
File Size-