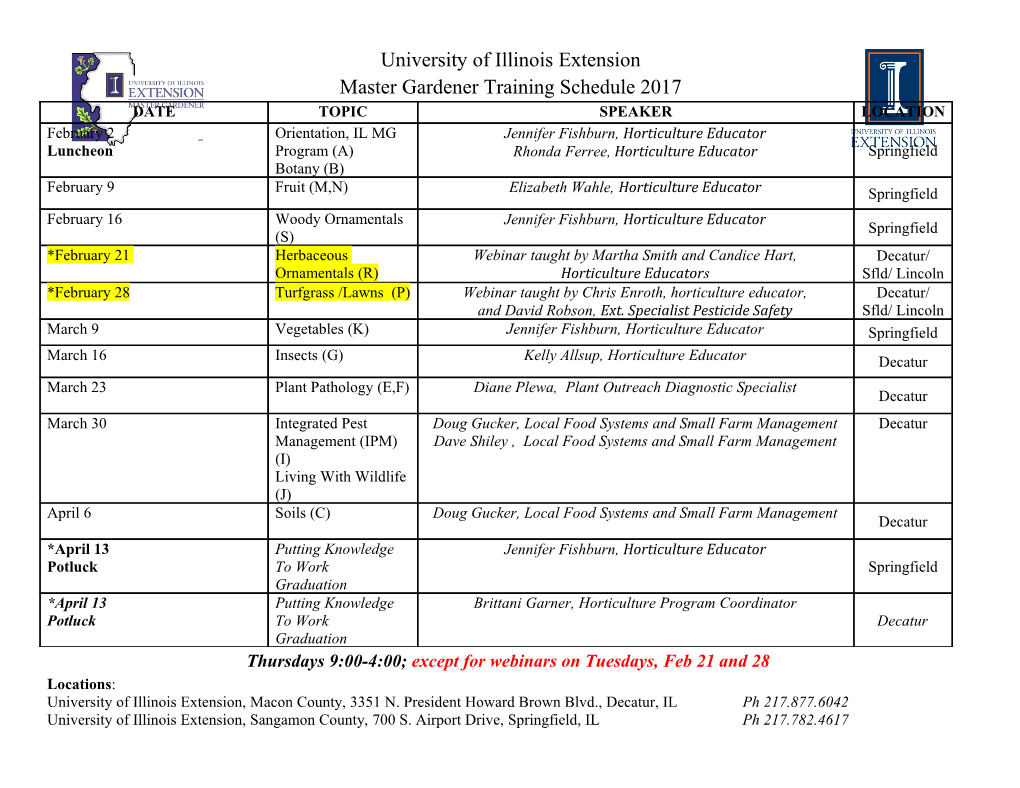
Index basis (topology), Ôç injective, ç basis (vector space), ç interior, Ôò isomorphic, ¥ ∞ C , Ôý, ÔÔ isomorphism, ç Ck, Ôý, ÔÔ change of variables (integration), ÔÔ kernel, ç closed, Ôò closed form, Þ linear, ç closure, Ôò linear combination, ç coboundary, Þ linearly independent, ç coboundary map, Þ nullspace, ç cochain complex, Þ cochain homotopic, open cover, Ôò cochain homotopy, open set, Ôý cochain map, Þ cocycle, Þ partial derivative, Ôý cohomology, Þ compact, Ôò quotient space, â component function, À range, ç continuous, Ôç rank-nullity theorem, coordinate function, Ôý relative topology, Ôò dierential, Þ resolution, ¥ dimension, ç resolution of the identity, À direct sum, second-countable, Ôç directional derivative, Ôý short exact sequence, discrete topology, Ôò smooth homotopy, dual basis, À span, ç dual space, À standard topology on Rn, Ôò exact form, Þ subspace, ç exact sequence, ¥ subspace topology, Ôò support, Ô¥ Hausdor, Ôç surjective, ç homotopic, symmetry of partial derivatives, ÔÔ homotopy, topological space, Ôò image, ç topology, Ôò induced topology, Ôò total derivative, ÔÔ Ô trivial topology, Ôò vector space, ç well-dened, â ò Glossary Linear Algebra Denition. A real vector space V is a set equipped with an addition operation , and a scalar (R) multiplication operation satisfying the usual identities. + Denition. A subset W ⋅ V is a subspace if W is a vector space under the same operations as V. Denition. A linear combination⊂ of a subset S V is a sum of the form a v, where each a , and only nitely many of the a are nonzero. v v R ⊂ v v∈S v∈S ⋅ ∈ { } Denition.Qe span of a subset S V is the subspace span S of linear combinations of S. Denition. A subset S V is linearly⊂ independent if ( ) ⊂ av v 0 each av 0. v∈S ⋅ = Ô⇒ = Denition. A subset S V is a basisQ if it is linearly independent and spans V. We assume the axiom of⊂ choice. Every vector space has a basis. Denition. If a vector space has a nite basis of size n, we say that dim V n. Otherwise, we say that V is innite-dimensional. = is makes sense because if dim V n, then all bases have size n. If W V is a subspace, and if dim W dim V, then V W. = Denition.⊂ A map L V W between= two vector spaces= V and W is linear if ∶ → L αv1 βv2 αL v1 βL v2 . Denition. e kernel or nullspace( of a+ linear) map= L( V) + W( is) the subspace ker L V dened by ker L v V L∶ v → 0 . ⊂ Denition. A linear map L V W is( injective) = { ∈if kerS (L ) 0.= } Denition. e image or range∶ →of a linear map L V =W is the subspace image L W dened by image L L v∶ W→ v V . ( ) ⊂ Denition. A linear map L V W is(surjective) = { ( if) image∈ S L∈ }W. Denition. A linear map L ∶ V→ W is an isomorphism if( there) = exists a linear map L−1 W V −1 −1 such that L L IdV and LL IdW . ∶ → ∶ → = = ç A linear map is an isomorphism i it is both injective and surjective. Denition. Vector spaces V and W are isomorphic if there exists an isomorphism from V to W. Note that V and W are isomorphic i dim V dim W. Denition. A sequence of linear maps = L−2 L−1 L0 L1 L2 L3 V−1 V0 V1 V2 V3 is exact if ker Li imageLi−1 ⋯Vi→. A nite→ sequence→ → of the→ form→ ⋯ = ⊂ L0 L1 L2 Ln−1 V0 V1 V2 Vn is exact if this condition holds wherever→ it makes→ sense,→ ⋯ i.e.→ for i 1, . , n 1 . Denition. Given a subspace W V0, a resolution of the subspace∈ { W is an− exact} sequence of the form ⊂ L0 L1 L2 Ln−1 Ln V0 V1 V2 Vn 0 such that ker L W. 0 → → → ⋯ → → Example. By the= Poincaré lemma, the de Rham complex of a star-shaped region U Rn is a reso- lution of the subspace of constant functions R Ω0 U . ⊂ eorem. If ⊂ ( ) L 0 V1 V2 is exact, then L is injective. → → Proof. ker L V0 0. = = eorem. If L V1 V2 0 is exact, then L is surjective. → → Proof. imageL ker V2 0 V2, so L is surjective. = ( → ) = Corollary. If L 0 V1 V2 0 is exact, then L is an isomorphism. → → → Proof. Since L is both injective and surjective, L is an isomorphism. ¥ Denition. A short exact sequence is an exact sequence of the form L1 L2 0 V1 V2 V3 0. eorem. For any short exact sequence of→ nite-dimensional→ → → vector spaces, dim V2 dim V1 dim V3. Proof. is proof may seem elaborate, but it follows the usual recipes of linear= algebra.+ Suppose dim V n. en we may take a basis e n of V . us L e n span the image of L . Since 1 i i=1 1 1 i i=1 1 n r n L1 is injective, L1 e are independent in V2. We can nd vectors f so that L1 e i i=1 j j=1 i i=1 r = { } { ( )} n f becomes a basis of V2. us dim V2 n r. Note that L1 e image L1 ker L2 , j j=1 { ( )} i i=1 { ( )} ∪ r so L2 L1 e 0. Now L2 is surjective, so the L2 f must span V3. If we can show that i = + j j=1 { ( )} ⊂ ( ) = ( ) r L2 f are independent, then dim V3 r, and the theorem follows. (j (j=1 )) = ( ) To show( ) independence, suppose a jL2 f=j 0. en 0 L2 a j f j a j f j ker L2 image L . Since L e n span the image of L , we have 1 1 i i=1 1 ∑ ( ) = = ∑ Ô⇒ ∑ ∈ = ( ) { ( )} r n a j f j bi L1 ei , j=1 i=1 Q = Q ( ) for some constants b n . In particular, i R i=1 { ∈ } n r bi L1 ei a j f j 0. i=1 j=1 Q − ( ) + Q = n r Since L1 e f are independent in V2, all the coecients b and a must be zero. i i=1 j j=1 i j We wanted to show that all the a j 0, so we are done. { ( )} ∪ { } eorem. If L V W, then = ∶ → 0 ker L i V L image L 0 is a short exact sequence, where i ker→ L V→ is the→ inclusion( ) map.→ Corollary (Rank-nullity). If L V∶ W→ is a linear map of nite-dimensional vector spaces, then ∶ dim→ V dim ker L dim imageL. Denition. e direct sum of V W of two= vector spaces+ is the vector space of pairs v, w v V, w W , where the operations are v1, w1 v2, w2 v1 v2, w1 w2 and α v1, w1 αv1, αw1 . ⊕ {( )S ∈ ∈ } eorem. If A, B V are( subspaces) + ( such that) ∶= V( span+ A +B ,) then ( ) = ( ) ⊂ 0 A B =A B ( V∪ )0 is a short exact sequence, where A B → A ∩B is→ the⊕ diagonal→ map→ v v, v , and the map A B V is the dierence map a, b a b. ∩ → ⊕ ↦ ( ) ⊕ → ( ) ↦ − Corollary. dim V dim A dim B dim A B . Denition. If W V is a vector subspace, then the quotient space is the set of equivalence classes = + − ( ∩ ) V W v v V and v v v v W . ⊂ 1 2 1 2 e equivalence classes~ are∶= thus{[ of] S the∈ form v[ ]v= [W], i.e.⇐⇒ translates− of∈ the} subspace W. Example. Suppose W x , 0, 0 3 x . en 3 W x , x , x where we ignore 1 R 1[ ]R= + R 1 2 3 the x1-coordinate. e x1-coordinate is still there, but it gets ignored. For example, ∶= {( ) ∈ S ∈ } ~ = {[( )]} 5, 3, 7 0, 3, 7 . We could always set the x -coordinate to zero, but we don’t have to. 1 [( )] = [( )] eorem. If W V is a subspace, then i q ⊂ 0 W V V W 0 is exact, where i is the inclusion map, and q is the quotient map q v v . → → → ~ → Corollary. ( ) = [ ] dim V W dim V dim W. Suppose that X and Y are sets, and that X~ has= an equivalence− relation . Given an element x X, let x denote its equivalence class. Let X denote the “quotient set” of equivalence classes. ∼ ∈ Denition. A function f X Y is well-dened on the quotient set if [ ] ~ ∼ f x f x whenever x x . ∶ → 1 2 1 2 When f is well-dened on X , it( makes) = ( sense) to dene a function∼ f X Y according to the rule ~ ∼ f x f x . ∶ ( ~ ∼) → Example. Suppose V1 and V2 are vector spaces, and W V1 is a subspace. In order to dene a linear map ([ ]) ∶= [ ( )] V1 W V2⊂, it suces to dene a linear map ~ → L V1 V2 and show that L is well-dened on the quotient space. We must check the condition ∶ → L v1 L v2 whenever v1 v2 L v1 v2 0 whenever v1 v2 W ( ) = ( ) ∼ L w 0 whenever w W ⇐⇒ ( − ) = − ∈ W ker L. ⇐⇒ ( ) = ∈ Since W is the subspace of elements which represent zero in the quotient, ⇐⇒ ⊂ a linear map is well-dened i everything which represents zero actually maps to zero. Whenever this holds, it makes sense to dene L V1 W V2 by L v L v . ∶ ( ~ ) → ([ ]) ∶=â [ ( )] Chain complexes Denition. A sequence of linear maps p−2 p−1 p p+1 p+2 p+3 d C p−1 d C p d C p+1 d C p+2 d C p+3 d is called a cochain complex⋯ →if image d→p−1 →ker d p →C p.
Details
-
File Typepdf
-
Upload Time-
-
Content LanguagesEnglish
-
Upload UserAnonymous/Not logged-in
-
File Pages14 Page
-
File Size-