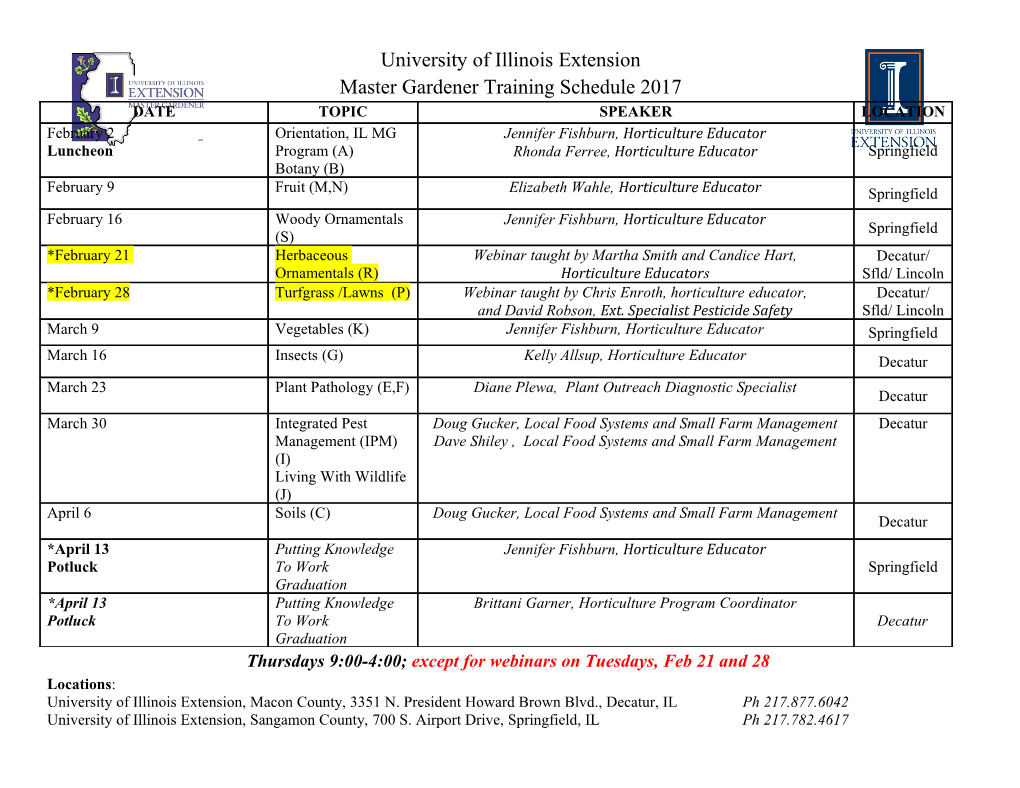
<p> Methane Digesters Anaerobic fermentation or digestion is the most promising process for converting organic materials to methane and other gases. A simple apparatus can be constructed to produce bio-gas. Bio-gas usually contains about 60 to 70 percent methane, 30 to 40 percent carbon dioxide, and other gases. The heat value of raw bio-gas is approximately half that of natural gas under typical Colorado conditions. Take precautions when processing and handling the gas. It is highly explosive and difficult to detect. </p><p>Kinetic Studies of Biogas Evolved from Water hyacinth</p><p>ABSTRACT Water Hyacinth - a native of South America is abundantly found in India, Bangladesh, South East Asia and in the Philippines Islands. Under favorable conditions a growth rate as high as 17.5 metric tons of wet Water hyacinth per hectare per day has been reported. Due to vegetative reproduction it spreads rapidly clogging drainage, ditches, shedding out other vegetations and interfering with shipping and recreation. </p><p>The concept of using aquatic plants for conversion to energy (methane) is gaining attention in tropical and sub tropical regions of the world where warm climate is conductive to the plant growth through out the year. Anaerobic digestion of organic matter is the oldest method for disposing the waste. The anaerobic digestion of animal, agricultural and industrial wastes has been widely studied. However, very little work has been done using aquatic plants particularly Water Hyacinth. </p><p>The present paper deals with the kinetics of gas produced from Water Hyacinth. The study was done in a batch fed digester. Attempts have been made to reach at an optimum condition for the production of maximum amount of gas by the addition of lower volatile fatty acids, Cow dung and inoculums etc. The important and useful results that was drawn from the study is that we can run the biogas plants even in the cold winter nights by using certain additives. After digestion of Water Hyacinth inoculums can be used as good manure for soil fertility, which is free from harmful chemicals, which is a boon for sustainable agriculture practices. INTRODUCTION TO METHANE GAS PRODUCTION - AL RUTAN Methane recovery from organic waste is appealing for several reasons. One is that the "raw" material is just laying around waiting for someone to do something with it. Unlike alcohol which has to be grown first as a starch grain and then processed, animal manure is "coming at you" if you have animals - whether you want it or not. It only makes sense to convert this "difficult resource" into something that is not only "socially acceptable" but also very profitable.</p><p>For instance, Fairgrove Farms in Sturgis, Michigan, milks 720 head. The manure from this herd is conveyed to a digester. The biogas produced fuels a caterpillar generator. The generator produces for the power grid in excess of $140.00 worth of electricity every 24 hours. So the farm receives from the local electrical utility a check in excess of $4,000.00 each month. Actually, it is probably more at the present time because this was the figure four years ago Producing biogas from animal manure is like making bread, beer or wine. All are examples of the process of fermentation. And it is obvious to anyone who has experience that these skills are an art as well as a science. Anyone can bake bread or make wine. But to be really good at these skills requires common sense and sensitivity to the parameters of nature working to succeed. Working with manure is similar. One cannot treat it like "shit" and expect the best results. It takes an abundance of common sense and sensitivity to what the methagenic organisms require to achieve the best results.</p><p>Methane, Biogas, or Gobar Gas (Gobar is the Nepali term for manure) is made by the anaerobic (in the absence of oxygen) digestion of manure and plant life. The purpose is to convert this manure into methane to use as cooking fuel. One method is to use a circular pit made of concrete, that is sealed and manure is added over time. Pipes lead from this container into the house, where gas is emitted at the cooking location. Residue from the combustion process comes out at a different location in a concentrated form and is used for fertilizer. Human waste may also be used as the bacteria is killed in the combustion process. Methane is a gas made up of one molecule of carbon and four molecules of hydrogen. It is the major component of the "natural" gas used in many homes for cooking and heating. It is odorless, colorless, and yields about 1,000 British Thermal Units (Btu) [252 kilocalories (kcal)] of heat energy per cubic foot (0.028 cubic meters) when burned. Natural gas is a fossil fuel that was created eons ago by the anaerobic decomposition of organic materials. It is often found in association with oil and coal. The same types of anaerobic bacteria that produced natural gas also produce methane today. Anaerobic bacteria are some of the oldest forms of life on earth. They evolved before the photosynthesis of green plants released large quantities of oxygen into the atmosphere. Anaerobic bacteria break down or "digest" organic material in the absence of oxygen and produce "biogas" as a waste product. (Aerobic decomposition, or composting, requires large amounts of oxygen and produces heat.) Anaerobic decomposition occurs naturally in swamps, water-logged soils and rice fields, deep bodies of water, and in the digestive systems of termites and large animals. Anaerobic processes can be managed in a "digester" (an airtight tank) or a covered lagoon (a pond used to store manure) for waste treatment. The primary benefits of anaerobic digestion are nutrient recycling, waste treatment, and odor control. Except in very large systems, biogas production is a highly useful but secondary benefit. Biogas produced in anaerobic digesters consists of methane (50%-80%), carbon dioxide (20%-50%), and trace levels of other gases such as hydrogen, carbon monoxide, nitrogen, oxygen, and hydrogen sulfide. The relative percentage of these gases in biogas depends on the feed material and management of the process. When burned, a cubic foot (0.028 cubic meters) of biogas yields about 10 Btu (2.52 kcal) of heat energy per percentage of methane composition. For example, biogas composed of 65% methane yields 650 Btu per cubic foot (5,857 kcal/cubic meter).</p><p>Electricity from cow dung By G.S. Dhillon IN a welcome move PEDA, the nodal agency of the Punjab Government for undertaking development of non-conventional sources of power, has embarked on the utilisation of cow dung or the cattle waste originating from the dairy units in the Haebowal complex in Ludhiana, for the production of electricity, in addition to organic manure. A modern plant is proposed to be put up which with an initial investment of around Rs 10 crore will supply about 1 MW of power, in addition to yielding organic manure. In the Haebowal complex there are about 200 private dairy units meeting the milk needs of the city’s population. The cattle waste or cow dung resulting from these dairy units are causing pollution problems. Most of the cow dung is “washed down” into the Budha Nullah on whose bank these dairy units are located.</p><p>The proposed scheme of PEDA will be putting up a state-of-the-art plant which has not been adopted in the country before. It is proposed to discuss the “improved mode” of anaerobic digestion of cattle waste (cow dung) in this write-up. Gobar gas plants: In the presently sponsored models of biogas units, both for “family scale” and the “community scale” units, the mode used is “mesophilic” which works at temperatures around 30°C to 40°C and the consortium bacteria gives better efficiency in the above temperature range. If the temperature drops below, as is the case in winter months, these units stop producing biogas. In this mode the detention period is very lary, so vessel or digestor has to have a large capacity. In the current models used, as both acidic and methagenoic stages which require alkaline environment takes place in one vessel, the whole process is very sensitive and the acid boilin conditions occur most often. The biogas produced is also stored in the same vessel, so problems result and the conversion efficiency of solids is low and so is the calorific value of the gas produced which requires a special burning unit. Thermophilic mode: In this mode the consortium of bacteria deployed for bio-degradation of solids is different from that deployed in the mesophilic mode. This bacteria work at higher temperatures of 50°C to 60°C and operate at a much faster rate and with better efficiency of solid digestion. The control provided in this mode is better than the other mode working at a lower temperature range i.e. mesophilic mode. The detention period required is also smaller, so the size of reactor is smaller. Usually separate vessels are used to accomplish both acidic and alkaline stages in different vessels and the gas produced from each stage is collected and stored separately. The gas obtained is mainly carbon dioxide and separation of this gas improves the quality and calorific value of the gas. To improve the quality of produced gas, it is scrubbed to remove hydrogen sulphide gas present which imparts obnoxious odour (rotten eggs). The improved gas has comparable characteristics to that of the LPG fuel and the same burners can be used for the so-improved biogas. From the collected cow dung, the first operation involved is removal of dirt, sand, stones, bricks, etc. before loading the digestor, which is suitably insulated to preserve the temperature of the process. For this removal a rotary drum sieve is utilised. The slurry comprises carbohydrates, proteins and fats, which are worked upon by the consortium of bacteria to produce biogas comprising carbon dioxide, nitrogen, methane and traces of hydrogen sulphide, etc. The ambient range of pH required to prevail in the mix is between 6.2 to 7.8. At the first stage, the digestion of solids into fatty acids takes place which are later worked upon by the methagenic bacteria into the methane gas. If the latter process proceeds at a slower rate, then there is a gradual accumulation of the fatty acids and drop of pH value occurs leading to acidic conditions prevailing which, in turn, needing buffering action to restore the alkaline environment. The effluent from the process contains a large amount of water, in addition to particles which would produce manure. So the effluents need to be dewatered for obtaining manure and this is accomplished in the screw press and solids are recovered from the effluent. Thereafter, the liquid is aerobically stabilised before disposal. The effluent flowing out conforms to the standards set by the pollution control agency. The manure obtained is aerobically stabilised and provides an ideal soil conditioner and organic fertiliser. Power generation is carried out by using the biogas obtained as fuel in compression ignited internal combustion engines which operate entirely on the biogas. The engines are coupled to the AC generators to produce electricity.</p><p>Conclusion:</p><p>This mode is regarded as eco-friendly because if the methane resulting from anaeorobic digestion of cow dung while composting is allowed to escape to atmosphere, it would add to the accumulative green house gases but when burnt it produces carbon dioxide which results in the abatement of climatic changes. The unit being set up at Ludhiana, if successful, would lead to setting up many similar units in other towns of Punjab. The experiment being carried out at Ludhiana is thus a trend-setter. History of Biogas Development The pioneer of biogas in Nepal was father B.R Sauboll, a Belgian teacher at Godavari St. Xavier's school. He built a demonstration plant in 1955. In 1968 Khadi and Village Industries Commission (KVIC) built a plant for an exhibition in Kathmandu. The Department of Agriculture installed 250 biogas plants during the fiscal year of 1975/76. The Agriculture Development Bank of Nepal (ADB/N) offered interest free loans for the installation of biagas plants. In 1974, Development and Consulting Service (DCS) built four biogas plants according to KVIC design. Gobar Gas and Agriculture Equipment Development Company Pvt. Ltd. was formed in 1977 with joint investment of the United Mission to Nepal (UMN), ADB/N and Nepal Fuel Corporation (which later on merged into the Timber Corporation of Nepal) based on DCS biogas extension organization. As it was difficult to introduce new technology, biogas in rural areas programme of the company was not encouraging in comparison to national potentialities. However, research on various design of biogas plant such as floating steel drum design, concrete fixed dome design, pre-cased tunnel design, plastic bag biodigester, ferrocement gas holders, brick mortar dome, mud dome were tested and experimented. However, fixed dome design is the only one recognized design and became more popular in Nepal. COMMERCIALISATION OF BIOGAS IN NEPAL </p><p>Biogas technology is becoming one of the reliable alternative energy sources in Nepal. As a result more than 48500 biogas plants have been installed in the country. However, it is only about 3.7% of its technical potentials. Biogas technology has been commercially introduced since the establishment of Gobar Gas Tatha Krishi Yantra Vikash (P) Ltd. in the year 1977. Various research have been carried out in designing and developing a biogas plant, biogas appliances, alternative feedstocks, maximising gas production especially in winter months and end use applications of gas and slurry. Even though these technologies have not came into practice and are limited only in papers, due to which the progress were not attractive as the planners planned. For commercialisation of biogas plants in Nepal, this paper has analysed potentiality of biogas plants in the country, sources and mechanisms of funding, construction capacity of the companies and users buying capacity with cost calculations. It has also highlighted some biogas promotional activities such as development and distribution of extension and promotion materials, marketing and slurry extension programme. It has focused on research and development, training, quality control and monitoring and evaluation of the programme. It has also highlighted the importance of co-ordination between its partners such as Biogas Companies, Nepal Biogas Promotion Group and other active NGOs, Biogas Appliances Manufacturers, Banks, BSP and AEPC with their clear responsibilities for the success of the programme. Ultimately, emphasis has been given for introducing a community trust fund concept, diversified end use applications of gas as well as slurry and integrated approach of biogas system for commercialisation of the technology in the country. In this way conclusions and recommendations are presented. </p><p>Basic Digester Process</p><p>Methane is produced by bacteria. The bacteria are anaerobes and operate only in anaerobic environments (no free oxygen). Constant temperature, pH and fresh organic matter promote maximum methane production. Temperatures usually are maintained at approximately 95 degrees F. Other temperatures can be used if held constant. For each 20 degrees F decrease, gas production will be cut approximately one half or will take twice as long. A constant temperature is critical. Temperature variations of as little as 5 degrees F can inhibit the methane-formers enough to cause acid accumulation and possible digester failure.</p><p>Anaerobic digestion is a two-part process and each part is performed by a specific group of organisms. The first part is the breakdown of complex organic matter (manure) into simple organic compounds by acid-forming bacteria. The second group of microorganisms, the methane-formers, break down the acids into methane and carbon dioxide. In a properly functioning digester, the two groups of bacteria must balance so that the methane-formers use just the acids produced by the acid-formers.</p><p>A simple apparatus can produce bio-gas. The amount of the gas and the reliability desired have a great influence on the cost and complexity of the system. A simple batch- loaded digester requires an oxygen-free container, relatively constant temperature, a means of collecting gas, and some mixing. Because methane gas is explosive, appropriate safety precautions are needed. Tank size is controlled by the number, size and type of animals served, dilution water added, and detention time. The factor that can be most easily changed with regard to tank size is detention time. Ten days is the minimum, but a longer period can be used. The longer the detention time, the larger the tank must be. Longer detention times allow more complete decomposition of the wastes. Fifteen days is a frequently used detention time. Table 1 shows some recommended sizes, dilution ratios and loading rates for different types of animals. Little volume reduction occurs in an anaerobic digester. Waste fed into the digester will be more than 90 to 95 percent water. The only part that can be reduced is a portion of the solids (about 50 to 60 percent). The processed material will have less odor. Because it still contains most of the original nitrogen, phosphorus and potassium, and is still highly polluted, the waste cannot enter a stream after it leaves the digester. Lagoons are commonly used to hold the waste until it can be disposed of by either hauling or pumping onto agricultural land. Table 1: Loading rate guidelines for heated, mixed anaerobic digesters at 95 degrees F being fed fresh livestock manures.*</p><p>Factor Swine Dairy Beef under Poultry layer Poultry (growing- 700 lbs broiler finishing) Dilution 1:2.9 Undiluted 1:2.5 1:8.3 1:10.2 ratio manure (manure to water) Estimated 15 0` 11 47 79 dilution water (gal water/1,000 lbs body wgt)** Hydraulic 12.5 17.5 12.5 10 10 detention time (days) Loading rate 0.14 0.37 0.37 0.13 0.1 (lbs volatile solids/cubic foot/day)** Digester 30 24 14 72 120 volume (cubic feet/1,000 lbs animal wgt)** *(From R.J. Smith, The Anaerobic Digestion of Livestock Wastes and the Prospects for Methane Production, Midwest Livestock Waste Management Conference, ISU, Ames, Iowa, Nov. 27-29, 1973) **To convert to metrics use the following equivalents: 1 gal = 3.8 l; 1 lb = .45 kg; 1 cu ft = .03 cu m. The volume of effluent actually may be greater than the volume of manure prior to digestion. This increase is due to the dilution water added to liquefy the manure to the desired solid content for the digester. There is no increase in the amount of nitrogen, phosphorus or potassium in this material, although it may be in a more available form. A small portion of the nitrogen may be lost to the gas portion of the system, thus reducing the amount of nitrogen initially available. Gas Production Total bio-gas production varies depending on the organic material digested, the digester loading rate, and the environmental conditions in the digester. Under ideal conditions (95 degrees F temperature and proper pH), it is possible to produce about 45 cubic feet of gas at atmospheric pressure from one day's manure from a 1,000 pound cow. Not all of the bio-gas energy is available for use. Energy is required to heat and mix the digester, pump the effluent, and perhaps compress the gas. Table 2 summarizes the estimated gas production from various animal wastes. Table 2: Bio-gas production (60% methane and 40% carbon dioxide) from animal wastes per 1,000 pounds body weight. Animal Volatile solids (lb per animal per day) Probable volatile solids destruction (percent)1 Gas (cu ft per day) Btu (per day)2 Beef 5.9 45 30 18,000 Dairy 8.6 48 44 26,000 Poultry, layers 9.4 60 72 43,000 Poultry, broilers 12.0 60 92 55,000 Swine (growing-finishing) 4.8 50 29 17,400 1Percent destruction of volatile solids varies depending primarily on detention time and digester temperature. 2Calculated at 600 Btu/ft3* (heat content varies depending on quality of gas). For comparison, some other heating values are: gasoline, 124,000 Btu/gal; diesel fuel, 133,000 Btu/gal; natural gas, 850 to 1,000 Btu/ft3; propane, 92,000 Btu/gal. *To convert to metrics, use the following equivalents: 1 lb = .45 kg; 1 cu ft = .03 cu m; 1 gal = 3.8 1.</p><p>Basic Elements</p><p>Figure 1 shows the basic elements of a single-stage anaerobic digester. Submerged inflow and outflow lines are needed to prevent gas from escaping. Either a mechanical mixer can be used, or the liquid or gas can be recirculated for mixing. A heat exchanger and thermostat maintain the proper temperature. The heat exchanger can be either internal or external.</p><p>Methane is drawn off the top of the digester. For gas utilization, a compressor and storage tank are used, along with the hardware to provide flame traps, regulators, pressure gauges, hydrogen sulfide scrubber, carbon dioxide removal and pressure relief valves. A common facility for gas storage is the floating cover that floats upward while maintaining essentially constant pressure.</p><p>Methane or bio-gas cannot be converted to a liquid under normal temperatures as can LP gas (LP gas liquefies at 160 psi). Under constant temperature, volume reduction is inversely proportional to the pressure; that is, as the pressure doubles, the volume becomes half as large. The more the gas is compressed, the more energy it takes to compress it.</p><p>Figure 1: Basic components of anaerobic digester. </p><p>Liquefaction of methane requires pressures of nearly 5,000 psi and is not practical. If the gas is compressed to just 1,000 psi, it requires about 1,320 Btu of energy to put 6,350 Btu into a storage container.</p><p>Because bio-gas cannot be liquefied, it is best suited for stationary uses, such as cooking, heating water and buildings, air conditioning, grain drying, or operating stationary engines. It is not feasible as a tractor fuel. One cubic foot of compressed bio-gas at 3,000 psi would run a 100-horsepower tractor approximately 7 1/2 minutes. Most tractor fuel tanks occupy about 8 cubic feet. A special high-pressure tank with 8 cubic feet of gas and 3,000 psi would run the tractor approximately one hour. A 3,000-psi tank bouncing around on a tractor would present a serious safety hazard. The tractor would run 6 minutes on 8 cubic feet of gas compressed to 300 psi, a more realistic pressure.</p><p>A well-insulated, three-bedroom home takes about 900,000 Btu per day for heating during cold weather. Because 50 percent of the bio-gas goes back into maintaining the necessary temperature of the digester, it would take the manure from 50 cows to produce enough bio-gas each day for home heating.</p><p>Bio-gas is produced on a relatively constant basis. Most applications are somewhat intermittent; therefore, storage is required. The amount of storage depends on the storage time and pressure. High demand applications, such as grain drying, normally are impractical due to the excessive storage capacity required.</p><p>Hazards</p><p>Methane in a concentration of 6 to 15 percent with air is an explosive mixture. Since it is lighter than air, it will collect in rooftops and other enclosed areas. It is relatively odorless and detection may be difficult. Extreme caution and special safety features are necessary in the digester design and storage tank, especially if the gas is compressed.</p><p>Summary</p><p>Concerns for energy conservation, environmental pollution, and the fact that agricultural organic wastes account for a major portion of our waste materials, has created renewed interest in the processing of these wastes for energy recovery. </p><p>Of the several types of energy capturing processes available, anaerobic digestion appears to be the most feasible for the majority of agricultural operations. Anaerobic digestion can stabilize most agricultural wastes while producing bio-gas or methane gas. This concept has been extensively applied in Europe and India during energy shortages. Similar equipment has been used for gas production with domestic wastes.</p><p>Primarily, disadvantages are the amount of management required due to the sensitivity of the digesters, the high initial investment required for equipment, and the fact that the wastes still must be disposed of after digestion.</p><p>Research is in progress to make the process more practical for energy production. Bacteriologists are investigating new strains of bacteria and culturing techniques for producing methane. Engineers are investigating digester designs and operation to reduce construction and operational requirements and costs.</p><p>Kinetic Studies of Biogas Evolved from Water hyacinth</p><p>F. Shoeb, H. J. Sing City Convent School Miyan Bazar (East) Gorakhpur – 273 001 (UP) INDIA</p><p>ABSTRACT</p><p>Water Hyacinth - a native of South America is abundantly found in India, Bangladesh, South East Asia and in the Philippines Islands. Under favorable conditions a growth rate as high as 17.5 metric tons of wet Water hyacinth per hectare per day has been reported. Due to vegetative reproduction it spreads rapidly clogging drainage, ditches, shedding out other vegetations and interfering with shipping and recreation. The concept of using aquatic plants for conversion to energy (methane) is gaining attention in tropical and sub tropical regions of the world where warm climate is conductive to the plant growth through out the year. Anaerobic digestion of organic matter is the oldest method for disposing the waste. The anaerobic digestion of animal, agricultural and industrial wastes has been widely studied. However, very little work has been done using aquatic plants particularly Water Hyacinth. The present paper deals with the kinetics of gas produced from Water Hyacinth. The study was done in a batch fed digester. Attempts have been made to reach at an optimum condition for the production of maximum amount of gas by the addition of lower volatile fatty acids, Cow dung and inoculums etc. The important and useful results that was drawn from the study is that we can run the biogas plants even in the cold winter nights by using certain additives. After digestion of Water Hyacinth inoculums can be used as good manure for soil fertility, which is free from harmful chemicals, which is a boon for sustainable agriculture practices.</p><p>KEY WORDS: BIOMASS, BIOGAS, ANAEORABIC DIGESTION, WATER HYACINTH, KINETICS, BIOENERGY, NON CONVENTIONAL ENERGY, RENEWABLE ENERGY.</p><p>INTRODUCTION</p><p>The socio-economic development of a country largely depends on the availability and consumption of energy. The available sources of energy can be classified into two categories viz., non – renewable and renewable. The non -renewable Sources of energy are finite deposits of coal, natural gas, U235 and deuterium which are also called fossil fuels. The renewable sources of energy are photosynthesis, solar energy, hydroelectric and tidal powers. In the renewable account energy is being deposited everyday whereas in the non- renewable account energy deposits are continuously depleted by our withdrawals. Because of continuous depletion in the natural resources by an increased consumption of the energy, alternatives of fossil fuels has to be searched out. Therefore, bioenergy is the only alternative and cheap source of energy which can be made available to the rural areas of the country.</p><p>Biomass </p><p>The term biomass is understood to mean all land and water plants, their wastes or by- products resulting from the transformation of these plants. There are different kinds of biomasses like animal waste, crop and agricultural residues, forest products and forestry residues and industrial wastes. The others are marine and aquatic biomass. Aquatic macrophytes grow rapidly and are considered as nuisance plants. Among floating macrophytes, Water hyacinth (Eichornia crassipes), water lettuce and pennywort are found to be most productive compared to small leaf floating plants. </p><p>Water Hyacinth as a Source of Energy</p><p>Due to vegetative reproduction and extremely high growth rate Water hyacinth spread rapidly clogging drainage, ditches, shading out other aquatic vegetation and interfering with shipping and recreation. Water hyacinth has attracted the attention of scientists to use it as a potential biomass for he production of biogas because of its high growth yield and availability in large amount throughout the year and allover the world.</p><p>Anaerobic digestion of organic matter is the oldest method for disposing the waste. The anaerobic digestion of animal (Jain, Singh, Tauro, 1981; Shelat, 1983) agricultural (Dunlop, 1975; Jewell, Dell’ Orto, Fanfoni, Fast, Jackson, Kabrick, Gottung, 1981; Jewell, Dell’ Orto, Fanfoni, Fast, Gottung, Jackson, Kabrick, 1982; Jewell, 1981; Wujcik, Jewell, 1981; Edi Iswanto Wiloso, Triadi Basuki, Syahrul Aiman, 1995) and industrial wastes(Unni, Pillai, Singh, 1987) has been widely studied. However, very little work has been done using aquatic plants particularly the Water hyacinth.</p><p>The kinetics for a blend of Water hyacinth – grass municipal wastes has been studied by Ghosh et al (Ghosh, Henry, Klass, 1980). Wolverton and Mc Donald ( Wolverton, Mc Donald, 1981) have suggested that anaerobic filter technique provides a large surface area for the anaerobic bacteria to establish and maintain an optimal balance of facultative, acid forming and methane producing bacteria. Unni et al (Unni, Pillai, Nigam, singh, Baruah, 1981) have reported that in the anaerobic digestion of Water hyacinth imperfect mixing of the content of the digester has no impact on the overall digestion rate. Vaidyanathan et al (Vaidyanathan, Kavadia, Shroff, Mahajan, 1985) have reported that higher methane production rate and lower retention time are obtained with ground Water hyacinth in comparison to chopped. Further they have found that the gas obtained from ground Water hyacinth had higher methane content.</p><p>During the present investigation a systematic study on the kinetics of biogas evolution from Water hyacinth is undertaken. The study was further elaborated by using a blend of Water hyacinth and Cow dung in different proportion and attempts have been made to reach at an optimum condition for the production of maximum amount of biogas.</p><p>EXPERIMENTAL</p><p>Kinetics of biogas evolved during the anaerobic degradation of Water hyacinth was done in a batch-fed digester. A schematic diagram of the experimental set up used is shown in figure 1. It consists of a 500 ml capacity glass bottle A which serves as a digester. The glass bottle A is connected to a gas collector B through a three way stop cock. The collector B is made from a glass tube of 1.0” i.d. and 4’ length and is connected to a water leveler C with the help of a polythene tubing. A narrow strip of graph paper was pasted on glass collector B and the later was calibrated between the two points B1 and B2. In order to do the calibration the leveler C was detached at the bottom and a rubber tube with a pinch- cock was fitted. Water was filled from the top of the column so that the water level could reach the point B1 was noted with cathetometer. 100 ml of water was taken out from column B by opening the pinch-cock at the bottom and the new level of water column was read. The difference of the two readings on the cathetometer would give the corresponding length measured on the glass column for 100 ml. Subsequent withdrawals of 100 mls were made and the positions of the levels on the glass columns B were determined and marked on the graph paper strip. The process was repeated till the water level reached just above the point B2. The calibration was repeated three times. A plot between the water column height measured from the reference point B1 and volume was made. Plot shows a linear relationship between the column heights and the volume. After getting the calibration done the leveler C was attached with the glass column B at the bottom. The volume of gas evolved during a particular time interval was measured over water at atmospheric pressure by leveling the water column levels in columns B and C. The distance between the two levels was noted on the graph paper and converted into corresponding volumes by multiplying with the calibration factor. The digester was kept in an air thermostat maintained at a particular temperature. A series of batch-fed reactors were placed together for studying the kinetics under varying conditions. The following parameters have been studied:</p><p>Figure 1: Experiment Setup for Studying the Biogas Evolution</p><p>Effect of Dilution of Water Hyacinth</p><p>In a fixed amount of Water hyacinth water was added in the ratio (by wt.) Water hyacinth: water as 1:1, 1:3 and 1:5.</p><p>Effect of Addition of Lower Volatile Fatty acids</p><p>Acetic acid was chosen as a representative lower volatile fatty acid. Different amount of acetic acid was added in the system containing Water hyacinth.</p><p>Effect of Addition of Cow Dung</p><p>Different amounts of Cow dung (Gobar) in the range of 2-10 gm were added in the systems containing 50 gm of chopped Water hyacinth in 250 ml of water. The kinetics has also been followed by keeping the total volatile solid (VS) content of Water hyacinth and Cow dung (Gobar) fixed.</p><p>Effect of Inocculum</p><p>Kinetics has also been studied by using inoculums of different incubation period. In order to prepare inocculum the following procedure was adopted. About 20 liters of slurry was made by mixing Cow dung and water in the ratio 4:5. About 2 litres of the slurry was distributed in 6 glass bottles of about 2.5 litres capacities. The glass bottles were fitted with a rubber cork having one hole. In the hole a glass tube was inserted which remained above the layer of the slurry. The other end was connected with Teflon tubing, the outlet of which was dipped in a container filled with water. With this set up the gas produced during the incubation period could bubble through water but no air would enter into the slurry thus, maintaining the anaerobic condition. This arrangement was made for all the six bottles. These bottles were kept in an air thermostat maintained at 40° C. After the expiry of the desired incubation period the bottles were opened and the contents were filtered through 60-mesh sieve. The filtrate was used as inoculums of 5, 15, 20, 30 and 40 days incubation periods were prepared and the following systems were made for detailed investigation.</p><p>Water Hyacinth 50gm + Inocculum 250ml (Incubation Period –5 days) Water Hyacinth 50gm + Inocculum 250ml (Incubation Period –15 days) Water Hyacinth 50gm + Inocculum 250ml (Incubation Period –20 days) Water Hyacinth 50gm + Inocculum 250ml (Incubation Period –30 days) Water Hyacinth 50gm + Inocculum 250ml (Incubation Period –40 days)</p><p>Further, varying amounts of inoculums of 15 and 20 days were taken at a fixed amount of Water hyacinth and the kinetics was followed. pH Measurement</p><p>The change in the pH of the biomass during anaerobic degradation was measured. The experimental setup consists of a 500 ml capacity conical flask. 250 ml slurry of a particular composition was added into it. The flask was capped with a rubber cork having two holes. Through one hole the electrode (combination type) was inserted and was dipped in the slurry. The electrode was connected to a pH metre. In the other hole a corning glass tube was fitted in such a way that the end of it remained above the slurry. The other end was connected to a gas collector. The flask was placed in an air thermostat maintained at 40º C. The production of biogas and pH were noted simultaneously as a function of time.</p><p>RESULTS AND DISCUSSION</p><p>Results plotted in fig. 2 show that blending of Water hyacinth in water is critical for producing maximum amount of biogas in a minimum time period. The ratio of 1:5 by weight for Water hyacinth and water has been found to produce maximum amount of gas. The Water hyacinth has a leafy structure. If sufficient amount of water is not added the biomass would not be soaked enough to go through the degradation process efficiently and hence a less amount of biogas is produced. Thus, detailed kinetic studies were performed using a blend of Water hyacinth and water in the ratio of 1:5 by weight. The results obtained from Cow dung slurry under the same condition are also plotted in fig. 2. The results show that Water hyacinth produce more gas per gm biomass fed as compared to Cow dung. This is true if the results are plotted per gm volatile solid because the percentage of total volatile solid in Water hyacinth and Cow dung has been determined to be almost the same. This is due to the fact that substrates for methanogenic bacteria are readily available from leafy plants such as Water hyacinth. The production rate of biogas from the same amount of Cow dung and Water hyacinth is plotted in fig.3. The results show that gas production rate from Water hyacinth is higher as compared to pure Cow dung slurry. However, the time period for attaining the maximum production rate is longer (about 40 – 50 days) for Water hyacinth as compared to Cow dung (20 – 30 days). This is due to the fact that bacteria needed for biogas production in the case of Water hyacinth takes a longer time period to grow whereas in ruminants waste such as Cow dung pathogens are already present and bacterial growth takes a little time for biogas production.</p><p>Figure 2: Effect of Dilution of Biomass on Gas Evolution Kinetics.</p><p> x, 50gm Water Hyacinth + 50 ml Water ∆ , 50gm Water Hyacinth + 100 ml Water¡, 50 gm. Water Hyacinth + 250 ml. Water, ? Cow Dung 50 gm+ 250ml Water</p><p>Figure 3: Rate of Biogas Production from Water Hyacinth and </p><p>Cow Dung with 50 gm Biomass in 250 ml Water.•, Water Hyacinth, x , Cow Dung</p><p>The addition of lower volatile fatty acid such as acetic acid has a remarkable effect on the total amount of gas produced during a particular time interval as well as on the gas production rate. The results plotted in fig. 4 show that an amount as little as 0.6 ml (10% by volume) of acetic acid in 250 ml of the blend of 50 gm of Water hyacinth and water increases the gas production by about one and a half time. The rate of production of biogas is also increased by more than twice as shown in fig. 5. However, addition of a larger amount of acetic acid (2 ml of 10% by volume) reduces the gas production rate as shown in fig. 5. This may be assigned to the fact that most of the biogas comes from the methyl group of the lower volatile fatty acid and the process is accomplished under methanogenic condition (Klass, 1984). The survival of the methanogenic bacteria largely depends on the pH of the medium. A larger addition of acetic acid thus makes the medium more acidic reducing the activity of methanogens and hence a lower rate of gas production has been observed. This is in accordance with the general observation made by Mc Carty and Mc Kinney (Mc Carty, Mc Kinney, 1961) and Andrews (Andrews, Sanit, 1969) that methanogenesis is facilitated in the presence of lower volatile fatty acids in a certain range of concentration. </p><p>Figure 4: Effect of Addition of Acetic Acid on Biogas Production from Water Hyacinth.</p><p> x, 50gm Water Hyacinth + 50 ml Water∆ , 50gm Water Hyacinth + 2.0 ml Acetic Acid (10 % by volume) + 250 ml Water ¡, 50 gm. Water Hyacinth + 0.6 ml. Acetic Acid (10 % by volume)+ 250 ml Water</p><p>Figure 5: Effect of addition of acetic acid on Biogas Production Rate from Water Hyacinth.</p><p> l, 50 gm Water Hyacinth + 250 ml water; ¡, 50 gm Water Hyacinth + 2.0 ml Acetic Acid (10 % by volume) + 250 ml water; x, 50 gm. Water Hyacinth + 0.6 ml. Acetic Acid (10 % by volume)+ 250 ml water</p><p>Results are also plotted to show the effect of mixing of Cow dung in the blend of Water hyacinth and water. It is found that the addition of Cow dung increases the gas production as well as it lowers the induction period. This may be assigned to the fact that the total volatile solid content of the system increases with the increased amount of Cow dung. The lowering of the induction period in the case of Cow dung + Water hyacinth system is due to the fact that the bacteria responsible for the degradation of biomass is facilitated by the addition of Cow dung.</p><p>Thus, the studies performed on the systems keeping the total volatile solid content fixed in the blend of Water hyacinth + Cow dung + water, the ratio of 2:3 by weight of Water hyacinth to Cow dung is found to be most suitable for the biogas production. The results plotted show that the production rate of such a system is more than double as compared to the Water hyacinth or the Cow dung systems taken separately under identical conditions.</p><p>Inoculums of different incubation periods have remarkable effect on biogas production. The total amount of gas produced at the end of decomposition is higher with inoculums of 15 and 20 days as shown in fig. 6. However, the rates of production of biogas while using inoculums of 20 and 30 days are higher as shown in fig. 7. Thus, it is inferred that inoculums of incubation period of 20 and 30 days are the best for the maximum production of biogas during a particular time period. It attains a maximum rate of 0.25 liter/day during the 5th day of gas production in comparison to a much longer time period of 20 to 30 days at a much lower rate of 0.045 litre / day in the case of Cow dung + Water hyacinth system with a 50 gm of biomass in each case. The total amount of gas produced depends on the amount of volatile fatty acid decomposed, which in turn would depend on the number of methanogenic bacteria present in the system. If the inoculums are kept for a longer period the amount of substrate does not increase further. However, there is a possibility of decreasing number of methanogenic bacteria in the inoculums of longer period because they are active only during a particular time period (Hobson, Bousfield, Summers, 1983). The less the bacterial count the lesser would be the gas production. The results plotted show that the gas production increases as the amount of inoculums is increased. The tendency of the curves towards a constant value shows the total amount of the gas available from the systems chosen for detailed studies. The values are different for each system and increase as the amount of inocculum is increased. This is probably due to the fact that in the inocculum prepared from Cow dung slurry some lower volatile fatty acids are also present and a large amount of inocculum would have greater amount of such acids which in turn produces biogas during methanogenesis. However, the rate of production of biogas does not depend on the amount of inocculum used. </p><p>Figure 6: Effect of Incubation Period of Inocculum on Gas Evolution Kinetics</p><p>¡, WH 50gm + Inocculum 250ml – Incubation Period 5 days r, WH 50gm + Inocculum 250ml – Incubation Period 15 days x, WH 50gm + Inocculum 250ml – Incubation Period 20 days £, WH 50gm + Inocculum 250ml – Incubation Period 30days ?, WH 50gm + Inocculum 250ml – Incubation Period 40days</p><p>Figure 7: Biogas Production Rate (Conditions are the same as in figure 6)</p><p>The pH change during the course of the degradation of Water hyacinth is shown in fig. 8. During the initial period of degradation there is a decrease in the pH showing thereby that first stage of anaerobic degradation leads to the production of acids. The results plotted in figure 8 also show that most of the biogas in the system containing 50 g Water hyacinth/250 ml water is produced once the pH of the system stabilizes around 7.0. </p><p>Figure 8: pH Change and Gas Evaluation During Anaerobic Degradation of Water Hyacinth.</p><p>System contains 50 gm W.H. + 250 ml water</p><p>The kinetic date has been fitted with the help of an integrated form of an equation.</p><p>[1]</p><p>Where a, b and c are constants. The analysis of the kinetic data with the help of the above equation was done in two parts. </p><p>Ø The system undergoing its own incubation for the generation of microbes, and Ø The systems in which microbes of different incubation periods were added which reduced the incubation period of biogas generation to almost zero.</p><p>In the first case microbial growth is the limiting factor. Under such a condition cV << 1, where c is related to the number of microbes present. In such a situation Eq. [1] can be written as </p><p>[2]</p><p> which could be reduced to </p><p>[3]</p><p>Plots of (1/V) (dV/dt) vs. V are made for the systems viz., (1) Water hyacinth + water, (2) Cow dung slurry and (3) Water hyacinth + Cow dung + water as shown in figs. 9-11. Straight lines are obtained which yielded the values of constants a and b as listed in Table 1. A close examination of the results show that a is related to the amount of substrate available for conversion into methane and b is related to the total amount of biodegradable substance available from feed for acidogenesis/hydrolysis leading to the substrates for conversion into biogas. In the case of pure Water hyacinth and pure Cow dung under the same condition the value of a is almost the same. However, the value of b in the case of Water hyacinth is almost two times less. This is in accordance with the analysis of Water hyacinth and Cow dung. The easily biodegradable matter particularly hemi-cellulose is higher in Water hyacinth than Cow dung (Robbins, Armold, Weiel, 1983). Further, the lignin content, which is not easily degradable, is higher in Cow dung slurry and thus yielding a lower value of b.</p><p>Figure 9: Plot of 1/V (dV/dt) Vs V for the System Containing Water Hyacinth 50gm in 250ml Water</p><p>Figure 10: Plot of 1/V (dV/dt) Vs V for the System Containing Cow Dung 50gm in 250ml Water</p><p>Figure 11: Plot of 1/V (dV/dt) Vs V for the System Containing Water Hyacinth and Cow Dung in the Ratio of 2:3 in 250ml Water</p><p>Table 1: Values of a and b for Various Systems</p><p>System Water hyacinth + Cow dung + Water a b (gm) (gm) (ml)</p><p>50 0 250 0.07 0.051 20 30 250 0.09 0.048 0.0 50 250 0.08 0.105</p><p>The second situation arises when the microbes are already present in plenty such as the systems containing inoculums. In such a situation cV >> 1 where the availability of substrate by acidogenesis / hydrolysis is the limiting factor. Thus, Eq. [1] reduces to the form </p><p>[4]</p><p>Where a’ = a/c and b’ = b/c</p><p>Plots of dV/dt vs. V are plotted. Straight lines are obtained showing the validity of Eq. [4] in the case of systems utilizing inocculums of different incubation periods. From these plots values of a’ and b’ are evaluated and listed in Tables 2 a-c for various systems.</p><p>Table 2a: Values of Constants a' and b' Using Inocculums of Different Incubation Periods Water Hyacinth = 50 gm; Amount of Inocculum = 250 ml Incubation period (Days) a’ b’</p><p>15 0.22 0.056 20 0.27 0.075 30 0.23 0.074 40 0.23 0.075</p><p>Table 2b: Values of Constants a' and b' at Varying Amount of Inocculums of 15 days Incubation Periods, Water Hyacinth = 50 gm</p><p>Amount of Inocculum (ml) a’ b’</p><p>100 0.142 0.045 200 0.190 0.045 250 0.220 0.056 300 0.240 0.060</p><p>Table 2c: Values of Constants a' and b' at Varying Amount of Inocculums of 20 days Incubation Period,</p><p>Water Hyacinth = 50 gm</p><p>Amount of Inocculum (ml)</p><p> a’</p><p> b’</p><p>100 0.192 0.060 200 0.258 0.075 250 0.272 0.075 300 0.308 0.075</p><p>The values of a’ are almost the same for the inoculums of different incubation periods provided the amount of inoculums used is the same. This is due to the fact that the numbers of microbes present in the system are sufficient high to convert the substrate available into biogas. However, increasing the amount of inocculum at a fixed incubation period increases the values of a’ and b’. This may be assigned to the fact that along with the microbes some substrates are present because inoculums are taken from Cow dung slurry, the content of which increases with increasing amount. </p><p>CONCLUSIONS</p><p>1. The total amount of gas produced from Water hyacinth is about one and a- half time higher than the Cow dung per gm volatile solid. </p><p>2. A blend of Water hyacinth and Cow dung in the ratio of 2:3 by weight is most suitable for biogas production.</p><p>3. Addition of very little amount of lower volatile fatty acid particularly acetic acid facilitates the gas production. This finding is very much helpful at the village level for the farmers using biogas plants. They face a great difficulty in production during the cold winter nights. During the same season most of the farmers in the sugar belt crush cane to produce GUD or thick syrup. If the leftover of the process of making Gud or cane sugar juice is kept for fermentation for a few days the content will be highly rich in acetic acid. The addition of this left over would circumvent the problem of lower gas production during the cold winter nights and biogas plants could run successfully during all the seasons. </p><p>4. The rate of production of biogas from Water hyacinth is higher as compared to Cow dung slurry. However, the fermentation process takes a longer time period in the case of Water hyacinth. The kinetic studies performed with Water hyacinth + inocculum show that gas production rate increases twelve times in a very short period of five days in comparison to Cow dung + Water hyacinth (20 – 40 days) systems.</p><p>Methane (Biogas) from Anaerobic Digesters</p><p>Methane is a gas made up of one molecule of carbon and four molecules of hydrogen. It is the major component of the "natural" gas used in many homes for cooking and heating. It is odorless, colorless, and yields about 1,000 British Thermal Units (Btu) [252 kilocalories (kcal)] of heat energy per cubic foot (0.028 cubic meters) when burned. Natural gas is a fossil fuel that was created eons ago by the anaerobic decomposition of organic materials. It is often found in association with oil and coal.</p><p>The same types of anaerobic bacteria that produced natural gas also produce methane today. Anaerobic bacteria are some of the oldest forms of life on earth. They evolved before the photosynthesis of green plants released large quantities of oxygen into the atmosphere. Anaerobic bacteria break down or "digest" organic material in the absence of oxygen and produce ‘biogas" as a waste product. (Aerobic decomposition, or composting, requires large amounts of oxygen and produces heat.) Anaerobic decomposition occurs naturally in swamps, water-logged soils and rice fields, deep bodies of water, and in the digestive systems of termites and large animals. Anaerobic processes can be managed in a "digester" (an airtight tank) or a covered lagoon (a pond used to store manure) for waste treatment. The primary benefits of anaerobic digestion are nutrient recycling, waste treatment, and odor control. Except in very large systems, biogas production is a highly useful but secondary benefit. </p><p>Biogas produced in anaerobic digesters consists of methane (50%-80%), carbon dioxide (20%-50%), and trace levels of other gases such as hydrogen, carbon monoxide, nitrogen, oxygen, and hydrogen sulfide. The relative percentage of these gases in biogas depends on the feed material and management of the process. When burned, a cubic foot (0.028 cubic meters) of biogas yields about 10 Btu (2.52 kcal) of heat energy per percentage of methane composition. For example, biogas composed of 65% methane yields 650 Btu per cubic foot (5,857 kcal/cubic meter). </p><p>Digester Designs</p><p>Anaerobic digesters are made out of concrete, steel, brick, or plastic. They are shaped like silos, troughs, basins or ponds, and may be placed underground or on the surface. All designs incorporate the same basic components: a pre- mixing area or tank, a digester vessel(s), a system for using the biogas, and a system for distributing or spreading the effluent (the remaining digested material). </p><p>There are two basic types of digesters: batch and continuous. Batch-type digesters are the simplest to build. Their operation consists of loading the digester with organic materials and allowing it to digest. The retention time depends on temperature and other factors. Once the digestion is complete, the effluent is removed and the process is repeated. </p><p>In a continuous digester, organic material is constantly or regularly fed into the digester. The material moves through the digester either mechanically or by the force of the new feed pushing out digested material. Unlike batch-type digesters, continuous digesters produce biogas without the interruption of loading material and unloading effluent. They may be better suited for large-scale operations. There are three types of continuous digesters: vertical tank systems, horizontal tank or plug-flow systems, and multiple tank systems. Proper design, operation, and maintenance of continuous digesters produce a steady and predictable supply of usable biogas. </p><p>Many livestock operations store the manure they produce in waste lagoons, or ponds. A growing number of these operations are placing floating covers on their lagoons to capture the biogas. They use it to run an engine/generator to produce electricity. </p><p>The Digestion Process</p><p>Anaerobic decomposition is a complex process. It occurs in three basic stages as the result of the activity of a variety of microorganisms. Initially, a group of microorganisms converts organic material to a form that a second group of organisms utilizes to form organic acids. Methane-producing (methanogenic) anaerobic bacteria utilize these acids and complete the decomposition process. </p><p>A variety of factors affect the rate of digestion and biogas production. The most important is temperature. Anaerobic bacteria communities can endure temperatures ranging from below freezing to above 135° Fahrenheit (F) (57.2° Centigrade [C]), but they thrive best at temperatures of about 98°F (36.7°C) (mesophilic) and 130° F (54.4°C) (thermophilic). Bacteria activity, and thus biogas production, falls off significantly between about 103° and 125°F (39.4° and 51.7°C) and gradually from 95° to 32°F (35° to 0° C). </p><p>In the thermophilic range, decomposition and biogas production occur more rapidly than in the mesophilic range. However, the process is highly sensitive to disturbances such as changes in feed materials or temperature. While all anaerobic digesters reduce the viability of weed seeds and disease-producing (pathogenic) organisms, the higher temperatures of thermophilic digestion result in more complete destruction. Although digesters operated in the mesophilic range must be larger (to accommodate a longer period of decomposition within the tank [residence time]), the process is less sensitive to upset or change in operating regimen. </p><p>To optimize the digestion process, the digester must be kept at a consistent temperature, as rapid changes will upset bacterial activity. In most areas of the United States, digestion vessels require some level of insulation and/or heating. Some installations circulate the coolant from their biogas-powered engines in or around the digester to keep it warm, while others burn part of the biogas to heat the digester. In a properly designed system, heating generally results in an increase in biogas production during colder periods. The trade-offs in maintaining optimum digester temperatures to maximize gas production while minimizing expenses are somewhat complex. Studies on digesters in the north-central areas of the country indicate that maximum net biogas production can occur in digesters maintained at temperatures as low as 72°F (22.2°C). </p><p>Other factors affect the rate and amount of biogas output. These include pH, water/solids ratio, carbon/nitrogen ratio, mixing of the digesting material, the particle size of the material being digested, and retention time. Pre-sizing and mixing of the feed material for a uniform consistency allows the bacteria to work more quickly. The pH is self-regulating in most cases. Bicarbonate of soda can be added to maintain a consistent pH, for example when too much "green" or material high in nitrogen content is added. It may be necessary to add water to the feed material if it is too dry, or if the nitrogen content is very high. A carbon/nitrogen ratio of 20/1 to 30/1 is best. Occasional mixing or agitation of the digesting material can aid the digestion process. Antibiotics in livestock feed have been known to kill the anaerobic bacteria in digesters. Complete digestion, and retention times, depend on all of the above factors. </p><p>Producing and Using Biogas</p><p>As long as proper conditions are present, anaerobic bacteria will continuously produce biogas. Minor fluctuations may occur that reflect the loading routine. Biogas can be used for heating, cooking, and to operate an internal combustion engine for mechanical and electric power. For engine applications, it may be advisable to scrub out hydrogen sulfide (a highly corrosive and toxic gas). Very large-scale systems/producers may be able to sell the gas to natural gas companies, but this may require scrubbing out the carbon dioxide. </p><p>Using the Effluent</p><p>The material drawn from the digester is called sludge, or effluent. It is rich in nutrients (ammonia, phosphorus, potassium, and more than a dozen trace elements) and is an excellent soil conditioner. It can also be used as a livestock feed additive when dried. Any toxic compounds (pesticides, etc.) that are in the digester feedstock material may become concentrated in the effluent. Therefore, it is importatn to test the effluent before using it on a large scale.</p><p>Economics</p><p>Anaerobic digester system costs vary widely. Systems can be put together using off-the- shelf materials. There are also a few companies that build system components. Sophisticated systems have been designed by professionals whose major focus is research, not low cost. Factors to consider when building a digester are cost, size, the local climate, and the availability and type of organic feedstock material. </p><p>In the United States, the availability of inexpensive fossil fuels has limited the use of digesters solely for biogas production. However, the waste treatment and odor reduction benefits of controlled anaerobic digestion are receiving increasing interest, especially for large-scale livestock operations such as dairies, feedlots, and slaughterhouses. Where costs are high for sewage, agricultural, or animal waste disposal, and the effluent has economic value, anaerobic digestion and biogas production can reduce overall operating costs. Biogas production for generating cost effective electricity requires manure from more than 150 large animals. </p><p>Below-ground, concrete anaerobic digesters have proven to be especially useful to agricultural communities in parts of the world such as China, where fossil fuels and electricity are expensive or unavailable. The primary purpose of these anaerobic digesters is waste (sewage) treatment and fertilizer production. Biogas production is secondary. </p><p>Natural Resource Agricultural Engineering Service Cooperative Extension 152 Riley-Robb Hall Ithaca, NY 14853-5701 Phone: (607) 255-7654; Fax: 607) 254-8770 Email: [email protected] World Wide Web: www.nraes.org This service has numerous publications related to livestock manure management, including a 68 page booklet, Anaerobic Digestion for Dairy Farms, which includes information on how to build them, how much output to expect per pound of manure, and how to modify gas appliances to use the gas. Single copies are $10.00, plus $3.75 shipping and handling. </p><p>GATE-ISAT Post Box 5180 Dag-Hammarskjöld-Weg 1-5 D-65726 Eschborn Federal Republic of Germany Phone: 49 6196 793185; Fax: 49 6196 797352 Email: [email protected] World Wide Web: http://gate.gtz.de/isat/ ISAT, the Information and Advisory Service on Appropriate Technology is a GATE project. GATE is a service provided by Deutsche Gesellschaft für Technische Zusammenarbeit (GTZ). They have five publications related to biogas digesters, including Biogas Plants (L. Sasse, 1982, 81 pp., DM 19.80), which provides design and construction details for simple biogas plants of the type widely used in China. An overview of biogas digesters is available on their Web site. </p><p>Volunteers in Technical Assistance (VITA) 1600 Wilson Boulevard, Suite 710 Arlington, VA 22209 Phone: (703) 276-1800 World Wide Web: www.vita.org VITA has various publications on small-scale biogas systems, including blueprints and designs of digesters used in China and India, which can be downloaded from their Web site. </p><p>This brief was updated in February 2001. </p><p>EREC is operated by NCI Information Systems, Inc. for the National Renewable Energy Laboratory/U.S. Department of Energy. The content of this brief is based on information known to EREC at the time of prepartion. No recommendation or endorsement of any non-US Government product or service is implied if mentioned by EREC.</p><p>Your interest in energy efficiency and renewable energy is greatly appreciated. If we can be of further assistance, please feel free to contact us again. </p><p>Energy Efficiency and Renewable Energy Clearinghouse (EREC) P.O. Box 3048 Merrifield, VA 22116 Voice (USA only): 800-DOE-EREC (363-3732) Email: [email protected]</p><p>NOTICE This report was prepared as an account of work sponsored by an agency of the United States government. Neither the United States government nor any agency thereof, nor any of their employees, makes any warranty, express or implied, or assumes any legal liability or responsibility for the accuracy, completeness, or usefulness of any information, apparatus, product, or process disclosed, or represents that its use would not infringe privately owned rights. Reference herein to any specific commercial product, process, or service by trade name, trademark, manufacturer, or otherwise does not necessarily constitute or imply its endorsement, recommendation, or favoring by the United States government or any agency thereof. The views and opinions of authors expressed herein do not necessarily state or reflect those of the United States government or any agency thereof. Consumer Energy Information Home | EREN Home | Ask an Energy Expert | Webmaster Appropriate Biogas Technology to Achcieve Sustainable Livelihoods</p><p>LEAD Indonesia M. Junaidi Chasani/DEWATS Octorber,2000</p><p>1. Introduction</p><p>Indonesia is a country with a population of ± 200 Million, 70 % of the population live in villages. The main income comes from the agricultural sector. Biomass potential (Cassava, manure, Cellulose, wood, staw, rice husks) is about 6.900.000 tons/year. Biomass can be transformed into energy resources (ethanol and methan gas), fertilizer, and other products. At present farmers commonly use chemical fertilizer in the low land areas. In the medium and high upland areas beside organic fertilizer, chemical fertilizers are used to plant vegetables. Farmers buy dried chicken dung as organic fertilizer from other areas. They prefer simple and appropriate solutions. Agricultural waste is not yet used, even though organic fertilizer is needed. Most of the organic materials such as leafs, branches are burned.</p><p>Besides fertilizers, farmers need energy for cooking, lighting, and home industries (tofu, brick, tile making, etc). Firewood, kerosene, rice husk, sawdust are mainly used as energy resources in the villages. Organic waste from livestock is regarded as dirty, but is a potential renewable energy resource. The problem is how can livestock waste be transformed into organic fertilizer and energy resources without polluting the environment?</p><p>Temperature in Indonesia averages 30-33 oCelcius, suitable for methanogen bacteria to grow. A biogas program in Indonesia is promoted by a local NGO, Institute for Rural Technology Development, (LPTP), in collaboration with BORDA (Bremen Overseas Research and Development Association) Germany. In many countries such as China, India, Tanzania, Burundi, Kenya, Nepal, Iran, Fiji, Indonesia, etc., biogas programs are already implemented. Major positive effects of these programs are protecting soil erosion, saving on possible energy resources, saving time for cooking, kitchen cleanes, animal/cattle more healthy, good milk quality, income increasing, decreasing pollution, eventually sustaining people’s livelihoods and environment. How to implemented a biogas program succesfully depends on many factors such as technology, climate, investment, policy, social, cultural, human resources, other alternative resources available (firewood, karosene, rice husk, sawdust, natural gas, electricity), etc. What kind of promotion and strategy has to be chosen in order to achieve the objectives?</p><p>Even if a biogas program is beneficial for environment and economy there are always some problems related to implementing the program This paper gives an account of implementing biogas program in Indonesian. It shows that biogas dissemination with community is feasible wherever agricultural conditions are suitable.</p><p>The Strategy in Implementing Biogas-plant with community </p><p>Base line data together with community Integrated biogas-agricultural-livestock Appropriate technology Equipment long life time, simple and strong Low maintenance</p><p>Beside that, support from government (policy) and investment are needed. </p><p>2. Baseline Data </p><p>Before biogas program is implemented, the first step is to collect baseline data. Baseline data is important data as it shows the major factor. The method to collect data can be RRA (Rapid Rural Appraisal) if the data collected together with farmers and the program decided by project. Another good method is PRA (Participatory Rural Appraisal) if the data is collected with farmer and the program decided by farmers themselves. With PRA the farmers are more involved in the project activities, so the farmers feel more responsible. This project using PRA but not pure PRA. In the government project, usually the data and program collected by government, which is one reason farmer does not feel responsible abaut that program, although the program is mean for farmers. It doe happen in Indonesia, that why the biogas research did by government is not succeeded. </p><p>3. Integrated Biogas-Agriculture-Livestock </p><p>In a tropical country like Indonesia, the temperature (30-33)ocelcius is suitable for methane bacteria but the farmer has many choises to use natural resources. In order biogas used by farmer in the rural area the biogas technology should be integrated with in existing agriculture farming and livestock system. Most faults in biogas units are caused by planning mistakes. Siting of the Bio-Digesters and layout of the Biogas-Agricultural-Livestock is as important as the construction itself. A good Bio- digester at the wrong place is a useless installation. It's same, as filling a digester with unsuitable materials will result in unproductive unit. </p><p>The Recycle process is: </p><p>Animal waste (cattle dung +urine) usually as waste and dirty polluted to the environment, with biogas technology, product methane gas as energy resource and slurry. Slurry as organic fertilizer need for agriculture and the effect to the land more porous to protect soil erosion. </p><p>In Indonesia (Java) land own by farmer about 600-800 m2/person, the distant some time far from their houses. Using slurry as fertilizer is more economic than only using methane gas (see addition). The problem of slurry is liquid, heavy and dirty. In order the farmer prefer to use slurry, it is necessary to have appropriate technology to handle slurry. If the technology is complicated with high cost the farmer doesn’t like to use it, because they have other alternatif of organic fertilizer (chicken dung), or others. The technology to separate liquid slurry with sand filter pit/dewatering pit. </p><p>4. Appropriate Technology </p><p>In tropical countries from the gasholder side, there are 3 types of digester in principle: 1.Fixed dome digester, or rectangular; 2. Floating dome digester. 3. Plastic cover digester. </p><p>1. Fixed dome digester </p><p>2.Floating drum digester </p><p>3. Plastic Cover Digester In Indonesia the 3 types of digester mention above already are tried in cattle farming. Because of the high scum problem, the type 1 (fixed dome digester is a choice), compared to others, the cost construction is higher. As metter of principle, sustainability has first priority over cost reduction. This means that everything must be arranged in such away that it is less work to feed the digester than not. The technology accepted by farmers is simple low maintenance and appropriate. The slurry flowing directed to the field is by gravitation. The materials used are selected by project staff together with the farmer. If the mason is careful when plastering the wall, and uses good quality material, no leaking problem will occur. Usually a fixed dome digester with a volume = 9 m3 (4 cattles dung modell), is sufficient for households energy consumption. Methan gas production is ± 600 liter/day/cattle. Household gas consumption is energy ± 1.200-2.000 liter biogas/day.</p><p>Digester type selection is the important step, before biogas program is disseminated, because if digester type can not be integrated into farming system, dissemination will be problematical.</p><p>5. Equipment Long lifetime, Simple and Strong </p><p>Biogas tools should be appropriate for the farmer. They must be long lasting and have to be regarded as a part of agricultural infrastucture (very different with computer technology where upgrades appear every 2 years). The farmers prefer simple biogas burners and not sophisticated luxury stoves as in the city. If the burner is complicated it is prone to breakdown more often. If that is happens farmers will use old energy sources.</p><p>6. Low Maintenance </p><p>Biogas is an appropriate technology. However the most difficult maintenance aspect is slurry handling. If the distant fields are located far away from stable, and biogas plant that should have dewatering pit. Fiborous slurry put in composting pit and liquid slurry goes into fishpond or absorption pit. To be more practical the composting pit must be near as possible to the dewatering pit. If the bio-digester activity has low maintenance the farmer will use properly, better environment condition, increasing their income, sustainable live hood will be achieved. </p><p>Cconclusion </p><p>Bio-digester can be a clever solution if the farmers use slurry as agriculture fertilizer. The most difficult maintenance is slurry handling, and scum problem. Suitable design, and location of the biogas plant is as important as construction Sustainability has first priority, this means that the biogas plant must become an integrated part of the farming system.</p><p>References:</p><p>1.Solon Barraclough, Krishna Ghimire, Hans Meliczek, "Rural Development Environment", english edition, UNRISD, Switzerland, 1997 2.Metcalf & Eddy, "Wastewater Engineering Treatment Disposal Reuse", third edition, Tata McGraw-hill Publishing Company LTD, New Delhi, 1996 3.------, "Making Development Sustainable", The World Bank, Washington, D.C., 1994. 4.Tata Budisatwika, "Paduan Pengelolaan Slury Bagi Petani Bimu (Bio Manure Unit) di Kabupaten Boyolali", IRDP LPTP-BORDA, Boyolali, 1994 5.Liao Xianming, "Anaerob Digester for Stabilizing Domestic Sewage", BRTC, Chengdu, 1993 6.M. Junaidi Chasani , "Petunjuk Perawatan Dan Pemakaian Biogas Unit", IRDP LPTP-BORDA, Boyolali, 1993 7.Anaerobic Technology and Use of Slury as Fertilizer Report, Study and Information Seminar for Project Staff, Borda, Bremen, 1992 8.------, "Participatory Rural Appraisal Handbook: Conducting PRAs in Kenya", National Environment Secretariat, Center for International Development and Environment of the World Resources Institute, 1991 9.Ludwig Sasse, "The Bio Digester at the hills of Central Java", BORDA-LPTP, Bremen, 1991 10.Dr. Michael S. Switzenbaum, "Overview of Anaerobic Treatment", Civil Engineering Department University of Massachusetts, Amhert, 1991. 11.Uli Werner/Ulrich Stohr/Nicolai Hees, "Biogas Plants in Animal Husbandry", GTZ, Germany, 1989. 12.L. Sasse, Dr. G. Mackensen, "Report on the project Biogas Survey 1988", BORDA, Bremen, 1989. 13.Prof. Ir. Hardjoso Projopangarso, "Teknologi Pedesaan", Laboratorium P4S F.T. – UGM Yogyakarta. 14.Khan, A.R, and E. Lee(eds.), "Proverty in Rural Asia", ILO-RTEP, Bangkok, 1984 Back to: Midterm Session Materials</p><p>Integrated Farming in Human Development - Proceedings of a Workshop </p><p>The Introduction of Low-Cost Polyethylene Tube Biodigesters on Small-Scale Farms in Vietnam</p><p>Bui Xuan An* , Thomas R. Preston** and Frands Dolberg***</p><p>* University of Agriculture & Forestry,</p><p>Thu Duc, Ho Chi Minh City, Viet Nam</p><p>E-mail: an%sarec%[email protected]</p><p>** University of Agriculture & Forestry,</p><p>Thu Duc, Ho Chi Minh City, Viet Nam</p><p>E-mail: thomas.preston%sarec%[email protected]</p><p>*** Department of Political Science,</p><p>University of Arhus, 8000c, Denmark</p><p>E-mail: [email protected]</p><p>Summary</p><p>In order to evaluate polyethylene tubular digester development in Vietnam, interviews were carried out in Thuan An district and at two extension centers. Data of design parameters, gas production, economic aspects, farmers' participation, technical problems and methodologies of biogas development were collected. The technology was appealing to the rural people because of its low costs, fast payback, simplicity and positive effect on pollution. The finding pointed to the importance of farmers’ participation for technical feedback, plant maintenance, plant repair and teaching of other farmers. The dissemination of the technology needs the selection of real farmers with high fuel demands as demonstrators, the support of a credit system for the poor farmers and strengthening farmer-extension-scientist relations. Follow-up research should be focused on on-farm studies, particularly the use of the slurry.</p><p>Key words: Plastic tube biodigester, Low cost, farmer impact, women’s lives, sustainable development.</p><p>Introduction</p><p>In recent years, the conversion of biomass to methane for use as an energy source has excited interest throughout the world. Biogas digestion was introduced into developing countries as a low-cost alternative source of energy to partially alleviate the problem of acute energy shortage for households. However, few farmers used biogas in practice. The poor acceptability of the digesters is believed to be due mainly to the high cost of the digesters, difficulty in installing them and difficulty in getting spare parts. The biogas programs developed quickly in some developing countries only under substantial support from governments and aid agencies (Gunnerson,, 1986; Kristoferson and Bokalders, 1991; Marchaim,, 1992; Karki,, 1996). Besides, the replacement of worn-out parts posed another technical problem, in addition to the fact that such spare parts were not always locally available.</p><p>Many developing countries, such as Colombia, Ethiopia, Tanzania, Vietnam, Cambodia and Bangladesh promoted the low-cost biodigester technology aiming at reducing the production cost by using local materials and simplifying its installation and operation (Solarte, 1995; Chater, 1986; Hieu et al, 1994; Sarwatt, 1995; Soeurn, 1994; Khan, 1996). To this end it was decided to use a continuous-flow flexible tube biodigester based on the bag digester model as described by Pound et al (1981) and later simplified by Preston and co-workers first in Ethiopia (Preston, unpubl.), Colombia (Botero and Preston, 1987) and later in Vietnam (An et al, 1994). Within three years, more than 800 polyethylene digesters were installed in Vietnam, mainly paid for by farmers (An and Preston, 1995). </p><p>The objectives of this study were to assess the effects of low-cost biodigesters in small farms in Vietnam and to identify experiences, effects, constraints and problems associated with this technology.</p><p>Materials and methods </p><p>Parameters of digesters</p><p>Data of design parameters and cost were collected from 194 biodigesters installed from April 1993 to December 1995 around Ho Chi Minh City.</p><p>Influence of biodigesters on the farmers’ lives</p><p>Open-ended interviews (Casley and Kumar, 1988) were carried out at 35 small farms with biodigesters in the Thuan An district, 40 km north of Ho Chi Minh City. In the selected area there were both upland and lowland ecosystems with 1800 mm rainfall, an average temperature of 27oC with small difference between seasons, sugarcane and cassava as the main crops and pigs as the most common animal (Thuan An people’s committee report, 1994; unpubl.). The questionnaire for farmers and their wives contained questions such as:</p><p>Use of the biodigesters: cooking parameters, economics of biogas uses, uses of effluent for fertilizer and other uses. </p><p>Farmers' participation: where did they get the information, payment of the digester, conditions before and after biogas use, opinions and suggestions. </p><p>Digester life: technical problems, when did problems occur, who fixed them, how was it done, what materials were needed. </p><p>Input and output from digesters </p><p>Manure inputs were weighed directly on most of the farms. In cases when weighing was impossible, the amounts were estimated by comparing with others farms with similar numbers and ages of animals. The amounts of water were weighed directly or estimated from multiplying water speed and washing time. </p><p>Gas production: by industrial gas-meter model k-875-1, Yazaki Keiki Co., Japan connecting to the gas outlet of digesters. </p><p> pH: by digital pH-meter. </p><p>Dry matter (DM) of the manure was measured by drying at 105oC until constant weight in a forced drought oven. </p><p>COD: Chemical Oxygen Demand (the amount of oxygen consumed for the oxidation of the reductive substances contained in a liter of liquid waste sample), using standard methods (HMSO, 1986). </p><p>The data and samples were taken for two consecutive days in the rainy season (from May to October) from 31 biodigesters randomly selected around Ho Chi Minh City. The average temperature in the area was 27.5oC and temperature difference between day and night was 10oC.</p><p>Interviews on extension and demonstration farms</p><p>The topic-focused interviews (Casley and Kumar, 1988) were carried out with two extensionist groups, one at BaVi, HaTay province and the other at ThuDuc, Ho Chi Minh City. The topics concerned selection and status of demonstration farms, list and rank of problems in the biogas development. The data were then verified by farmer’s interviews and field observations.</p><p>Results and discussion</p><p>Design parameters and cost of digesters around Ho Chi Minh City</p><p>The data are presented in table 1. The average length of the digesters was 10.2 m with an estimated digesta volume of approximately 5.1 m3 (length x 0.5 m3). The material cost was slightly more than US$25 for a family digester.</p><p>Table 1. Mean values for some design parameters and cost of 194 digesters installed around Ho Chi Minh City </p><p>Mean Range Length (m)</p><p>Digester liquid volume (m3)</p><p>Distance to kitchen (m) Material cost (US$)</p><p>Time to first gas production (days)</p><p>Digesters in rural areas(%)</p><p>Floating digesters (%) 10.2</p><p>5.1</p><p>23</p><p>25.4</p><p>17</p><p>91</p><p>5 4 - 30</p><p>2 - 15</p><p>8 - 71</p><p>14 - 82</p><p>1 - 60</p><p>In most developing countries, when the subsidies from governments are reduced the number of plants built each year falls dramatically (Ellis and Hanson, 1989; Qiu et al 1990; Desai, 1992; Karki et al, 1994). The most important problem in biogas programs in developing countries has been the prices of digester plants. For exempla: The price of a concrete digester plant installed for an average family in Vietnam varied from 180 to 340 US$ (Thong, 1989). This size of investment is considered unaffordable by average farm families (An et al, 1994). Chinese designers tried to reduce the cost of red-mud digesters to 25-30 US$/m3 (Gunnerson and Stuckey 1986), but it was still high in comparison with the polyethylene, digesters (5 US$/m3). This is obviously one important feature which makes the polyethylene digesters attractive and no farmer in the present study complained about the price. Among the polyethylene digesters installed, 5% of them were floated in ponds adding an innovative feature to the development. According to Khoi et al (1989), in the Mekong Delta where most land is low, the application of concrete digesters was very difficult especially when the water level went up. The floating digesters solved this problem and as they also required little space they were very well suited for low-lying areas. More than 90% of the plants were installed in rural areas indicating the good fit of the technology under these conditions.</p><p>Influence of biogas on small farms in the ThuanAn district</p><p>The effects of the introduction of digesters on small farms are presented in tables 2-4.</p><p>Table 2. Economic aspect of biogas introduction in 31 small farms in ThuanAn district, Vietnam </p><p>Mean Range Cooking time (hour)</p><p>Fuel saved in cooking (US$/month)</p><p>Biogas plant cost (US$/unit)</p><p>Number of pigs/farm</p><p>Payback time (month) 4.4</p><p>6.5</p><p>34.8</p><p>10.7</p><p>5.4 1- 9</p><p>1.8-13.6</p><p>18 - 53</p><p>0 - 40 2- 19</p><p>Table 3. Farmers’ participation and opinions on plastic biodigesters in ThuanAn district, Vietnam </p><p>Alternatives No.a Getting first information from Neighbours or relatives</p><p>Mass media 32</p><p>3 Payment of the digester plants Farmers paid totally</p><p>Partially (demonstration) 33</p><p>2 Using slurry for Plants</p><p>Ponds</p><p>Nothing 3</p><p>3</p><p>31 Status of gas production Enough gas</p><p>Little gas</p><p>No gas 26</p><p>5 4 Advantages of biogas Saves money</p><p>Less pollution</p><p>Easy cooking 34</p><p>35</p><p>35 a) Number of farmers</p><p>Table 4. Input and output of 31 digesters working at small farms around Ho Chi Minh City, Vietnam </p><p>Mean Range Size of family</p><p>Manure loading (kg/d)</p><p>Ratio Water/manure</p><p>Loading rates (kgDM/m3)</p><p>Temperature of loading( oC)</p><p>Temperature of effluent (oC)</p><p> pH of loading</p><p> pH of effluent</p><p>Gas production (l/unit/day)</p><p>Vol. Gas/capita (l/person/day)</p><p>Methane ratio (%)a CODb of loading (g/litre)</p><p>COD of effluent (mg/litre)</p><p>COD removal rate (%) 5.9</p><p>16</p><p>5.1</p><p>0.7</p><p>26.4</p><p>27.0</p><p>6.7</p><p>7.2</p><p>1235</p><p>223</p><p>56</p><p>35.6</p><p>13.5</p><p>62 3 - 12</p><p>2 - 27</p><p>2.9 - 8.1</p><p>0.1 -1.2</p><p>25.7 - 28.5</p><p>26.0 - 29.1</p><p>6.4 - 7.1 6.8 - 7.5</p><p>689 - 2237</p><p>68 - 377</p><p>45 - 62</p><p>22.4- 46.0</p><p>8.8 - 23.9</p><p>42 - 79 a From 9 digesters b COD = Chemical Oxygen Demand (the amount of oxygen consumed for the oxidation of the reductive substances contained in a litre of liquid waste sample by a strong oxidizer).</p><p>According to the annual report of ThuanAn people’s committee (1994, unpubl.), most of the farms with biodigesters belonged to the medium income group (sufficient food all year round). In this group animal production is a very important component the farming systems and a sufficient number of animals is important for the dissemination of biodigesters. The expense for the digester plant was paid back within slightly more than 5 months, so most of the farmers found a great benefit from installing them.</p><p>Among 35 farmers interviewed, four of them were poor (not enough food in certain months) The most important thing for them is food and they could not afford a sufficient number of animals for feeding manure to the digester. They wanted to borrow money to be able to raise animals. Four farmers had no gas when the interview was carried out. Three of them did not have animals because they found raising animals unprofitable if they had to borrow money from local lenders at 5-10% monthly interest. This was an important aspect as especially resource-poor farmers cannot support the digester installation and keep animals, although they know</p>
Details
-
File Typepdf
-
Upload Time-
-
Content LanguagesEnglish
-
Upload UserAnonymous/Not logged-in
-
File Pages44 Page
-
File Size-