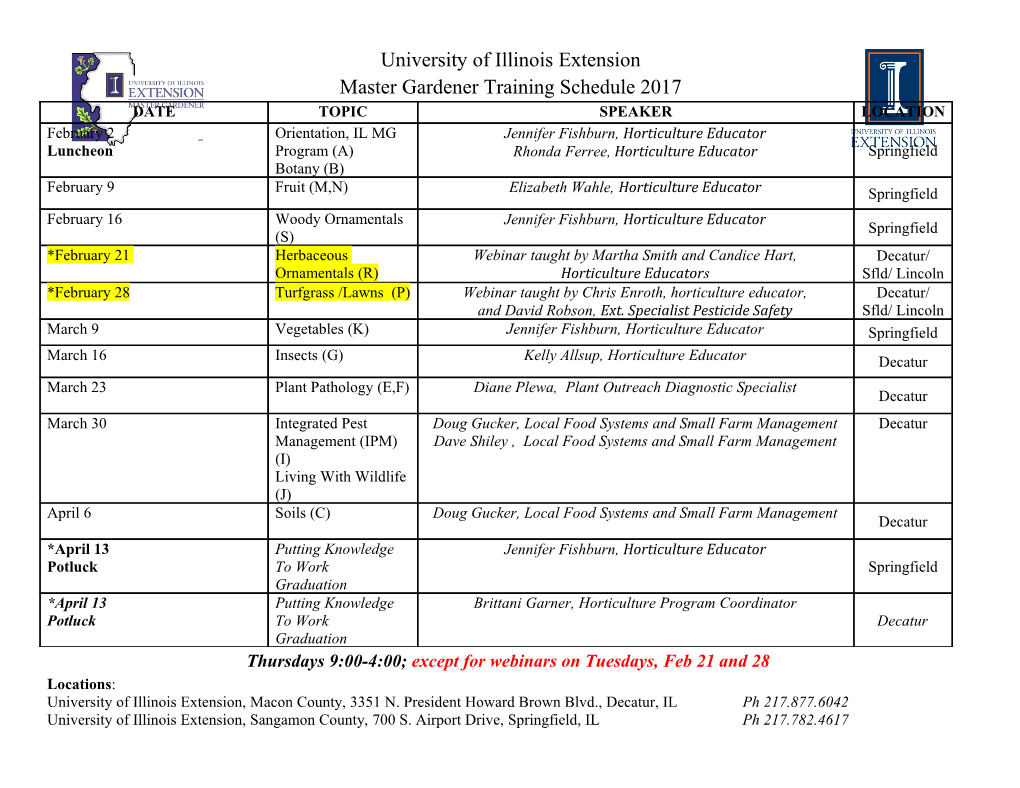
<p> 1 Morphological variation of the caudal fossa of domestic cat skulls assessed with computed</p><p>2 tomography and geometric morphometrics analysis </p><p>3</p><p>4 Caroline R Gordon1, Thomas W Marchant2, Joanna Lodzinska1, Jeffery J Schoenebeck2, Tobias</p><p>5 Schwarz1</p><p>6</p><p>7 1Royal (Dick) School of Veterinary Studies, University of Edinburgh, Roslin, United Kingdom</p><p>8 2Roslin Institute, University of Edinburgh, Roslin, United Kingdom</p><p>9</p><p>10 Corresponding author: Caroline R Gordon BVSc MRCVS </p><p>11 [email protected] </p><p>12 Hospital for Small Animals, Royal (Dick) School of Veterinary Studies, University of Edinburgh</p><p>13 Midlothian EH25 9RG, United Kingdom</p><p>14 Tel: +44 (0) 7729 246706</p><p>15</p><p>16 Keywords: caudal fossa, foramen magnum, computed tomography, geometric morphometrics</p><p>17 analysis, feline, occipital</p><p>18</p><p>19 Author Note</p><p>20 Preliminary results of this study were presented at the 2016 European Veterinary Diagnostic Imaging </p><p>21 Conference in Wroclaw, Poland. </p><p>22</p><p>23</p><p>24 25 Abstract</p><p>26 Objectives This study aimed to investigate differences and demonstrate a normal range of</p><p>27 morphological variation of the caudal fossa of the cranium of domestic cats. </p><p>28 Methods Computed tomographic scans of thirty-two domestic cat heads of eleven breeds were</p><p>29 included. Isosurfaces from skulls were characterised through three-dimensional geometric</p><p>30 morphometrics using geographic landmarks placed on the internal surface of the caudal fossa and</p><p>31 foramen magnum. Raw data was transformed with a Procrustes fit and coordinate covariance was</p><p>32 analysed by principal components to establish breed- and sex-level differences. Skulls were also</p><p>33 classified according to the number of concavities along the mid sagittal vermiform impression.</p><p>34 Differences were investigated between breed groups and sex, and correlation was sought with age. </p><p>35 Results Analyses revealed size-independent differences in occipital bone morphology across breeds</p><p>36 and sex, however no clustering was evident. Most variability was observed at the exoccipital bones,</p><p>37 ventral portion of the supraoccipital bone, dorsum sellae of the basisphenoid, and the osseous</p><p>38 tentorium cerebelli. No statistically significant differences were identified via 2-sample t-tests</p><p>39 between breed groups or sexes. No statistically significant correlation using Spearman Rho</p><p>40 correlation coefficient was identified with age. </p><p>41 Conclusions and relevance The feline caudal fossa displays a wide range of intra- and inter-breed </p><p>42 variation, not linked to age or sex. Concavities along the vermiform impression have not previously </p><p>43 been described. As advanced imaging modalities are becoming more frequently used for domestic </p><p>44 felids, an established range of normality is important for discriminating pathological changes from </p><p>45 anatomical variances.</p><p>46</p><p>47</p><p>48</p><p>49 50 Introduction</p><p>51 The caudal fossa has been the focus of extensive research in humans and canids, in part due to its role</p><p>52 in the pathogenesis of various disorders such as Chiari and Chiari-like malformations as well as </p><p>53 occipital dysplasia.1-8 There is, however, a paucity of information regarding variability in skull </p><p>54 morphology amongst existing breeds of the domestic cat (Felis catus). With the increasing use of </p><p>55 advanced diagnostic imaging in this species, recognition of the breadth of normal anatomical </p><p>56 variation is crucial. In the authors’ experience, cats display a wide variability in caudal fossa shape in </p><p>57 the absence of associated clinical signs.</p><p>58</p><p>59 The caudal fossa of the cranial cavity encompasses the midbrain, pons, and medulla oblongata </p><p>60 ventrally and the cerebellum dorsally. Rostrally it extends to the dorsum sellae of the sphenoid bone </p><p>61 and the osseous tentorium cerebelli of the occipital bone. Caudally it is enclosed by the supraoccipital </p><p>62 bone, which forms the dorsal border of the foramen magnum.9 The internal surface of the </p><p>63 supraoccipital bone displays shallow depressions which conform to the surface of the cerebellum; the </p><p>64 vermiform impression.9 The basioccipital bone forms the ventral border of the foramen magnum and </p><p>65 joins the basisphenoid via a cartilaginous suture. The exoccipital bones form the lateral portions of the</p><p>66 foramen magnum and bear the convex occipital condyles.9 </p><p>67</p><p>68 The objectives of this study were to investigate and describe differences in caudal fossa shape and </p><p>69 determine whether these differences are stereotyped by breed or sex. To detect shape trends of the </p><p>70 caudal fossa, the authors used computed tomography, an imaging modality that is amenable to </p><p>71 geometric morphometrics analysis. </p><p>72</p><p>73 Materials and methods</p><p>74 Sample population 75 Imaging records of the authors’ academic veterinary referral hospital were searched retrospectively </p><p>76 between January 2011 and December 2015 to identify adult cats (>12 months of age) that had </p><p>77 undergone a CT examination of the entire head to at least the level of the second cervical vertebra. </p><p>78 Studies were excluded if there were any pathological cranial or neural abnormalities identified on the </p><p>79 imaging study or clinical records. All CT examinations were acquired with the same 4-slice CT unit </p><p>80 (Siemens Somatom) using a 1mm slice width, 1.5 pitch, 130mAs, 120kV, 0.75s tube rotation time, and </p><p>81 high resolution image kernels (proprietary term U90).</p><p>82</p><p>83 Three-dimensional landmarking</p><p>84 Skull isosurfaces were generated from DICOM images using Stratovan Checkpoint </p><p>85 (v2016.11.21.0711). Twelve geographical landmarks were placed on the internal surface of the caudal </p><p>86 fossa and foramen magnum of each skull (Figure 1a,b) to represent the overall shape and dimensions.</p><p>87 Specifically, landmarks were placed at the osseous tentorium cerebelli, along the internal sagittal </p><p>88 curvature of the caudal fossa, opisthion, basion, the basioccipital bone, dorsum sellae, and the lateral-</p><p>89 most points of the internal surface of the foramen magnum.</p><p>90</p><p>91 Geometric morphometric analysis</p><p>92 Landmark coordinates and classifier data were imported into MorphoJ (v1.06d)10 for geometric </p><p>93 morphometric analysis. Superimposition, translation, rotation, and scaling of landmark </p><p>94 configurations were performed via a generalised Procrustes fit prior to generating a covariance </p><p>95 matrix. A one-way analysis of variance (ANOVA) was used to evaluate differences in centroid size </p><p>96 (the square root of the sum of squared distances of landmarks from the central point) amongst breed </p><p>97 groups. A multivariate regression of the symmetrical component of shape versus log centroid size </p><p>98 was performed to remove the influence of allometry, the shape changes correlating to changes in size.</p><p>99 Principal components analysis (PCA) is a mathematical algorithm which reduces and expresses a 100 dataset in terms of vectors along which variation is maximal. A PCA of the resulting regression </p><p>101 residuals11 was used to explore patterns of covariance amongst landmarks. </p><p>102</p><p>103 Classification</p><p>104 A midline sagittal CT image was created for each cat using multi-planar reconstruction on freely </p><p>105 available DICOM viewing software (Horos v2.0). The skulls were classified into groups based on the </p><p>106 number of concavities along the vermiform impression of the caudal fossa. One reader, a second year </p><p>107 radiology resident, categorised each image, blinded to the signalment of each case. A two-sample t-</p><p>108 test was used to evaluate differences in concavity number between sexes, and between breed groups. </p><p>109 A Spearman rank correlation coefficient (rs) sought correlations between the number of concavities </p><p>110 and age, PC1, PC2, and centroid size of the caudal fossa. A p-value of < 0.05 was considered </p><p>111 significant. </p><p>112</p><p>113 Results</p><p>114 The study population comprised of thirty-two CT scans of domestic cat heads. There were two entire </p><p>115 females, one entire male, eleven neutered females, and eighteen neutered males. Entire and neutered </p><p>116 cats were grouped together for statistical purposes; thus the overall study numbers were thirteen </p><p>117 (41%) female and nineteen (59%) male. Eleven breeds were represented including Abyssinian (n=1), </p><p>118 British shorthair (n=1), Bengal (n=1), Burmese (n=2), Chinchilla (1) domestic long hair (DLH) (n=3), </p><p>119 domestic short hair (DSH) (n=15), Maine Coon (n=4), Ragdoll (n=1), Siamese (n=2), and Tonkinese </p><p>120 (n=1). For statistical purposes, cats were grouped according to phylogenetic origin into Asian cats </p><p>121 (Burmese, Siamese, Ragdoll, Tonkinese; n=6), Western European cats (Abyssinian, Maine Coon, </p><p>122 British shorthair, Chinchilla; n=7), and mixed breeds (DLH, DSH, Bengal; n=19)12. CT images of all </p><p>123 cats were sufficiently detailed to allow consistent placement of all landmarks on stereotyped locations</p><p>124 of the caudal fossa and foramen magnum. </p><p>125 126 Geometric morphometrics</p><p>127 For the symmetric component of shape with allometry, principal component (PC) 1 accounted for </p><p>128 21.6% of the variation amongst all cats. PC2 accounted for 15.4% of variation. PC1 and PC2 thus </p><p>129 cumulatively accounted for 37.0% of the observed variation. Most variability explained by PC1 was </p><p>130 observed at the exoccipital bones and dorsum sellae. As the exoccipital markers shifted caudally, the </p><p>131 dorsum sellae shifted dorsally, whilst the roof of the cranial fossa shifted, less markedly, in an overall </p><p>132 rostral direction. Neighbouring landmarks along the roof of the caudal fossa varied between </p><p>133 rostroventral and rostrodorsal trends. Variability explained by PC2 was observed at the osseous </p><p>134 tentorium cerebelli and foramen magnum. As the tentorium shifted ventrally, the basion and ventral </p><p>135 portion of the supraoccipital bone shifted dorsally and caudally, respectively. None of the PCs </p><p>136 discriminated subpopulations based on sex (Figure 2a) or breed groups (Figure 2b). Western </p><p>137 European cat breeds demonstrated the largest mean caudal fossa size and Asian cats the smallest, </p><p>138 however centroid sizes were not significantly different between breed groups (p = 0.432) (Figure 3). </p><p>139</p><p>140 After controlling allometry by regressing out isometric size, PC1 accounted for 20.3%, and PC2 16.0% </p><p>141 of the shape variation, cumulatively 36.3%. Overall shape trends matched the raw data in terms of </p><p>142 direction, however varied slightly in increments. Size-corrected PCA revealed no segregation of PC </p><p>143 scores between males and females (Figure 4a), nor was there any clustering amongst breeds (Figure </p><p>144 4b). </p><p>145</p><p>146 Occipital bone classification</p><p>147 Three cats displayed zero internal concavities along the vermiform impression, fourteen displayed </p><p>148 one concavity, eight cats displayed two, and seven cats displayed three (Figure 5a,b,c,d and Tables 1 </p><p>149 and 2). No statistically significant differences were identified between the number of concavities </p><p>150 along the mid sagittal vermiform impression of males compared to females (p = 0.917), nor was there </p><p>151 significant difference between the mixed breeds and purebreds (p = 0.634). No significant correlation 152 was identified between the number of concavities and age (p = 0.527), PC1 (p = 0.562), PC2 (p = 0.873), </p><p>153 and centroid size (p = 0.086). </p><p>154</p><p>155 Table 1 Relationship between vermiform impression morphology and sex</p><p>Concavities Male Female 156</p><p>0 2 (11) 1 (8) 1 8 (42) 6 (46) 2 5 (26) 3 (23) 3 4 (21) 3 (23) Total 19 (100) 13 (100) 157 Data are n (%) unless otherwise specified</p><p>158</p><p>159 Table 2 Relationship between vermiform impression morphology and breed group</p><p>Concavities Purebred Mixed breed 160</p><p>0 2 (15) 1 (5) 1 5 (38) 9 (47) 2 3 (23) 5 (26) 3 3 (23) 4 (21) Total 13 (99) 19 (99) 161 Data are n (%) unless otherwise specified</p><p>162</p><p>163 Discussion</p><p>164 A wide range of morphometric variation of the caudal fossa and foramen magnum was observed in </p><p>165 this study population of neurologically normal cats, with no identified links to breed, sex, or age. </p><p>166 Because PC shape trends were similar between the raw data and the regression residuals, and there </p><p>167 were no significant differences in centroid sizes between breed groups, the authors conclude that </p><p>168 allometry had negligible influence on shape differences of the caudal fossa. </p><p>169</p><p>170 Shape variability demonstrated by PC1 may be interpreted anatomically as a lengthening of the </p><p>171 exoccipital bones occurring alongside a relative dorsoventral flattening of the entrance to the caudal </p><p>172 fossa caused by dorsal shifting of the dorsum sellae. Rostral shifting of the roof of the caudal fossa 173 reflects an alteration in space distribution. A previous morphometric study categorised feline skulls </p><p>174 into three phenotypically different skull formations; rounded, triangular, and cuneiform.13 This study </p><p>175 identified a frequent variant of the round-shaped skulls where the foramen magnum extended more </p><p>176 distantly between the occipital condyles thereby acquiring a bilaterally narrowed oval shape, similar </p><p>177 to the findings of the current study. Variability of rostroventral versus rostrodorsal directions of this </p><p>178 shift amongst neighbouring landmarks of the caudal roof of the fossa demonstrates an irregular </p><p>179 contour of this internal bony surface. The vermiform impression is described in dogs as an irregular </p><p>180 excavation of the median portion on the cerebellar surface of the squamous part of the occipital </p><p>181 bone.14 This is, to the authors’ knowledge, the first description of this anatomical feature specifically </p><p>182 in the cat.</p><p>183</p><p>184 Shape variability demonstrated by PC2 may be interpreted anatomically as an overall dorsoventral </p><p>185 flattening of the entrance to the caudal fossa owing to ventral shifting of the osseous tentorium </p><p>186 cerebelli. Caudal extension of the foramen magnum coinciding with relative dorsoventral narrowing </p><p>187 of the foramen reflects an overall redistribution of space. The ventral portion of the supraoccipital </p><p>188 bone shifted caudally, independently of the more dorsal landmarks, demonstrating a variable caudal </p><p>189 angulation of this site relative to the internal contour of the caudal fossa. Similar findings have been </p><p>190 reported in a recent MRI study of cats which described variants of the ventral aspect of the </p><p>191 supraoccipital bone of cats being straight throughout, caudally angulated, blunt ended, and/or </p><p>192 ventrally thickened.15 The same study also found that cats could be divided into those with a thin and </p><p>193 those with a thick occipital bone. Compared with MRI, CT has a higher sensitivity for accurately </p><p>194 displaying osseous contours, and adds further validation to these findings.</p><p>195</p><p>196 Morphology of the external squamous part of the occipital bone and foramen magnum of cats has </p><p>197 been described in a study involving gross morphology of 50 European cat cadavers.16 Two </p><p>198 morphological patterns of the squamous part of the occipital bone were identified; a triangular shape 199 and a semi-oval shape, denoting normal variation of this external surface amongst European cats. </p><p>200 None of the specimens in this previously reported study displayed a dorsal notch, nor any other </p><p>201 defect at the foramen magnum. The authors concluded that, in the European cat, the foramen </p><p>202 magnum is free from pathology and its shape is conservative. In contrast, a morphometric study of 69</p><p>203 purebred cat skulls identified two individuals with a keyhole shaped foramen.13 Dogs display a wide </p><p>204 diversity of shape of the foramen magnum, many of which are considered as physiological variations </p><p>205 of morphology.8,17 It is apparent that a degree of physiological variation is also present in the cat and </p><p>206 that the spectrum of morphologies observed in purebreds is also seen in mixed-breed cats. From a </p><p>207 clinical perspective, this variation is important to recognise so that pathological processes are not </p><p>208 falsely assigned. </p><p>209</p><p>210 The majority (69%) of the study population displayed one or two concavities along the vermiform </p><p>211 impression on median plane CT images. No sexual dimorphism was identified, nor did these </p><p>212 concavities differ between purebreds and mixed breeds, nor correlate with age. Concavities along the </p><p>213 vermiform impression have not previously been described. A certain degree of care must be taken </p><p>214 comparing the appearance of the caudal fossa on CT with the anatomy. Post mortem examinations to </p><p>215 confirm the shape of the caudal fossa were not performed because none of these clinical cases were </p><p>216 available for necropsy examination. The high spatial frequency allowed by CT, however, ensures a </p><p>217 detailed and accurate representation of the osseous surface.18 Although the current study did not </p><p>218 identify significant differences between breed groups or sexes, or correlate with age, it is evident that </p><p>219 the shape of this vermiform impression is not uniform amongst cats and these variants should be </p><p>220 clinically recognised. </p><p>221 </p><p>222 A limitation of this study is the relatively small sample size. The lack of segregation in the PCA and, </p><p>223 likewise, the lack of differences amongst vermiform impression groups may reflect the small numbers</p><p>224 of individuals representing each breed, age, and sex, and thus the inability to achieve statistical 225 significance. Categorisation of vermiform impression concavities was somewhat subjective and prone</p><p>226 to bias due to the use of only one evaluator. Additional studies inclusive of larger groups of feline </p><p>227 breeds would be recommended to further investigate interbreed morphology differences. Breeds of </p><p>228 particular interest are those that are brachycephalic, as brachycephaly has been shown in dogs to be </p><p>229 associated with Chiari-like malformation19 and occipital dysplasia.17 Further studies could also </p><p>230 include lateralised landmarks along the caudal fossa, not included in the current study, which may </p><p>231 uncover further variants. </p><p>232</p><p>233 Conclusions </p><p>234 A wide range of inter-breed morphometric variation of the feline caudal fossa was observed in this </p><p>235 population, which was not linked to age or sex, demonstrating that caudal fossa anatomy is not </p><p>236 homogeneous amongst cats. The findings support and expand on those previously reported13,15,16. An </p><p>237 established range of normality is important for accurate discrimination between pathological changes </p><p>238 and normal anatomical variants, and for the recognition of potential trends associated with disease in </p><p>239 this popular companion animal. </p><p>240</p><p>241 Conflict of Interest</p><p>242 The authors declared no potential conflicts of interest with respect to the research, authorship, and/or </p><p>243 publication of this article.</p><p>244</p><p>245 Funding</p><p>246 The authors received no financial support for the research, authorship, and/or publication of this </p><p>247 article.</p><p>248 249 Figure 1 Landmarks along the internal surface of the caudal fossa (a) and foramen magnum (b). </p><p>250 Images are not to scale. In (b), a portion of the occipital bone has been cropped out.</p><p>251 Figure 2 PC1 vs PC2 of the symmetric component displaying PC scores classified by sex (a) and </p><p>252 breed (b)</p><p>253 Figure 3 Box and whisker diagram of centroid size amongst breed groups. The horizontal line within </p><p>254 each box represents the median. </p><p>255 Figure 4 PC1 vs PC2 after controlling for allometry displaying PC scores classified by sex (a) and </p><p>256 breed (b)</p><p>257 Figure 5 Concavities along the vermiform impression; (a) zero (b) one (c) two (d) three</p><p>258</p><p>259 References</p><p>260 1. Noudel R, Jovenin N, Eap C, et al. Incidence of basioccipital hypoplasia in Chiari </p><p>261 malformation Type I: comparative morphometric study of the posterior cranial fossa. J </p><p>262 Neurosurg 2009; 111: 1046-1052.</p><p>263</p><p>264 2. Urbizu A, Poca MA, Vidal X, et al. MRI-based morphometric analysis of posterior cranial </p><p>265 fossa in the diagnosis of Chiari malformation type I J Neuroimaging 2013; 24: 250-256.</p><p>266</p><p>267 3. Yan H, Han X, Jin M, et al. Morphometric features of posterior cranial fossa are different </p><p>268 between Chiari I malformation with and without syringomyelia Eur Spine J 2016; 25: 2202-</p><p>269 2209.</p><p>270</p><p>271 4. Shaw TA, McGonnell IM, Driver CJ, et al. Caudal cranial fossa partitioning in cavalier King </p><p>272 Charles spaniels Vet Rec 2013; 172: 341.</p><p>273</p><p>274 5. Knowler SP, McFayden AK, Freeman C, et al. Quantitative analysis of Chiari-like </p><p>275 malformation and syringomyelia in the Griffon Bruxellois dog PLoS ONE 2014; 9: e88120. 276</p><p>277 6. Knowler SP, Kiviranta AM, MacFayden AK et al. Craniometric analysis of the hindbrain </p><p>278 and craniocervical junction of Chihuahua, Affenpinscher, and cavalier King Charles </p><p>279 spaniel dogs with and without syringomyelia secondary to Chiari-like malformation PLoS </p><p>280 ONE 2017; 12: e0169898.</p><p>281</p><p>282 7. Rusbridge C, Knowler SP. Coexistence of occipital dysplasia and occipital </p><p>283 hypoplasia/syringomyelia in the cavalier King Charles spaniel J Small Anim Pract 2006; 47: </p><p>284 603-606.</p><p>285</p><p>286 8. Watson AG, de Lahunta A, Evan HE. Dorsal notch of the foramen magnum due to </p><p>287 incomplete ossification of the supraoccipital bone in dogs J Small Anim Pract 1989; 30: 666-</p><p>288 673.</p><p>289</p><p>290 9. König HE and Liebich HG (eds). Veterinary Anatomy of Domestic Mammals. Stuttgart, </p><p>291 Germany: Schattauer GmbH, 2004. </p><p>292</p><p>293 10. C. P. Klingenberg. 2011. MorphoJ: an integrated software package for geometric </p><p>294 morphometrics. Molecular Ecology Resources 11: 353-357.</p><p>295</p><p>296 11. Klingenberg CP, Barluenga M, Meyer A. Shape analysis of symmetric structures: </p><p>297 quantifying variation among individuals and asymmetry Evolution 2002; 56: 1909-1920.</p><p>298</p><p>299 12. Lipinski MJ, Froenicke L, Baysac KC et al. The ascent of cat breeds: Genetic evaluations of </p><p>300 breeds and worldwide random-bred population Genomics 2008; 91: 12-21.</p><p>301 302 13. Kunzel W, Breit S, Oppel M. Morphometric investigations of breed-specific features in </p><p>303 feline skulls and considerations on their functional implications Anat Histol Embryol 2003; </p><p>304 32: 218-223.</p><p>305</p><p>306 14. Evans HE and de Lahunta A (eds). Miller's anatomy of the dog. 4th Edition. St Louis, MO: </p><p>307 Elsevier, 2013.</p><p>308</p><p>309 15. Huizing X, Sparkes A, Dennis R. Shape of the feline cerebellum and occipital bone related </p><p>310 to breed on MRI of 200 cats J Feline Med Surg Prepublished November 10 2016, DOI: </p><p>311 10.1177/1098612X16676022.</p><p>312</p><p>313 16. Wasowicz M, Kupczyńska M, Wielądek A et al. Morphometric analysis of occipital bone in </p><p>314 the domestic cat in comparison with selected skull size parameter and with special regard </p><p>315 to skull morphotype Pol J Vet Sci 2009; 12: 251-258.</p><p>316</p><p>317 17. Kupczyńska M, Czubaj N, Barszcz K et al. Prevalence of dorsal notch and variations in the </p><p>318 foramen magnum in dogs of different breeds and morphotypes Biologia 2017; 72: 230-237.</p><p>319</p><p>320 18. Ruff CB and Leo FP. Use of computed tomography in skeletal structure research Yearb Phys </p><p>321 Anthropol 1986; 29: 181-196.</p><p>322</p><p>323 19. Schmidt MJ, Neumann AC, Amort KH et al. Cephalometric measurements and </p><p>324 determination of general skull type of cavalier King Charles spaniels Vet Radiol Ultrasound </p><p>325 2011; 52: 436-440.</p>
Details
-
File Typepdf
-
Upload Time-
-
Content LanguagesEnglish
-
Upload UserAnonymous/Not logged-in
-
File Pages13 Page
-
File Size-