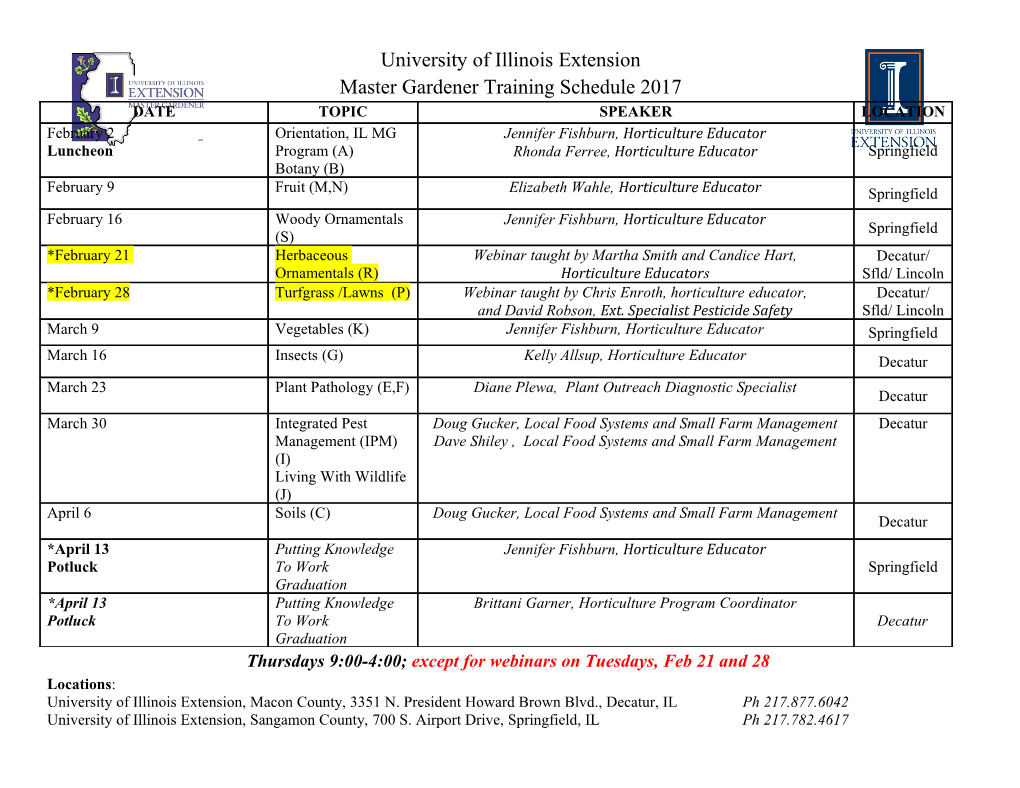
<p> 1 1Modeling apple snail population dynamics on the Everglades Landscape</p><p>2</p><p>3Philip C. Darby, Donald L. DeAngelis, Stephanie Romañach, Kevin Suir, and Joshua </p><p>4Bridevaux</p><p>5 Supplementary Material</p><p>6Appendix 1. Detailed description of EverSnail model parameterization, submodels, and</p><p>7description of their derivation</p><p>8</p><p>9The estimation of parameters for the EverSnail model is described below, following a </p><p>10description of pertinent apple snail life history and ecology details. Additional details and </p><p>11references on apple snail life history and ecology are available in a literature review by the </p><p>12Pomacea Project (2013). </p><p>13</p><p>14Summary of Apple Snail Life History and Ecology</p><p>15Estimates for apple snail life span were reported from several lab and field studies, and based </p><p>16on different types of observations. Hanning (1979) concluded that Florida apple snails had a </p><p>171-1.5 year life span based on a shift in the size-frequency distribution (with a disappearance </p><p>18of larger size classes) as well as finding an increase in the number of dead adult-sized snails </p><p>19in a concentrated time period in June. Ferrer et al. (1990) reported a significant increase in </p><p>20mortality in 15-20 month old P. paludosa directly observed in situ in wetlands in Cuba. </p><p>21Darby et al. (2003) observed a steep decline in survival in May-June for both snails in the </p><p>22field (bearing transmitters) and in the lab, regardless of water level. Additional study showed</p><p>23the steep decline in survival immediately followed peak egg cluster production (i.e., a post-</p><p>24reproductive die-off) (Darby et al. 2008). The concentration of egg production in spring the </p><p>25previous year (see details below), and the increased mortality observed in summer the </p><p>2 1 3 26following year (~12-15 months later), are consistent with a 1-1.5 year life span for P. </p><p>27paludosa. </p><p>28 As with all Pomacea snails studied to date, the Florida apple snail can survive </p><p>29periodic dry downs typical of the tropical and subtropical wetlands they inhabit (Cowie 2002,</p><p>30Darby et al. 2008). Water depths recede, with possible temporary reversals, during the dry </p><p>31season, which generally occurs from January-June. Dry marsh conditions, in years they </p><p>32occur at all, are most often found in April-June (Kushlan 1990, Darby et al. 2008). Dry down</p><p>33survival rates depend on reproductive status of adults-sized snails, and snail size in general. </p><p>34Reproductive and post-reproductive adults die regardless of water depths (including dry </p><p>35downs) as a result of a life cycle that culminates in a post-reproductive die-off (Darby et al </p><p>362003). Adult-sized snails that had not reproduced (average shell width 31 ± 4.2 mm) survive </p><p>374 weeks in dry conditions with survival at 98%; this declines to 71% at 12 weeks and 27% at </p><p>3818 weeks (Darby et al. 2008). Juvenile-sized snails exhibited significantly lower survival </p><p>39rates, where 10-15 mm and 6-9 mm size class survival was ~80% after four weeks in dry </p><p>40conditions. After 8 weeks 10-15 mm snail survival was 43% and 6-9 mm snail survival was </p><p>4113%. Newly hatched snail survival (<6 mm) was more variable, but still significantly lower </p><p>42than the larger juvenile size classes (50% or fewer of the newly hatched snails survived after </p><p>434 weeks and all were dead at 8 weeks) (Darby et al. 2008).</p><p>44 All Pomacea species are dioecious (Cowie 2002), and Hanning (1979) estimated a 1:1</p><p>45sex ratio for the majority of samples he collected on Lake Okeechobee, which we apply to </p><p>46our population model. Hanning (1979) found that snails were sexually mature when shells </p><p>47reached approximately 35 mm in size. Darby et al. (2008) reported egg-laying females and </p><p>48mating males of somewhat smaller size (~25-30 mm) in the Upper St. Johns basin. Females </p><p>49deposit clusters of cleidoic eggs above the water line on emergent vegetation; each cluster </p><p>50typically consists of 20-40 eggs (Hanning 1979, Turner 1996). Hanning (1979) reported an </p><p>4 2 51average of one cluster produced per female per week, and Hurdle (1974) recorded 21 and 25 </p><p>52total clusters produced by two Florida apple snails (total fecundity, and what influences it, </p><p>53requires further study). Egg clusters can be found year round; however, from November </p><p>54through January or early February few or no eggs are found (Hanning 1979, Darby et al. </p><p>552008). Darby et al. (2008) reported ~80% of annual egg cluster production in April-June </p><p>56(greatest annual egg counts also occurred in April, May, or June); in the seven transects </p><p>57reported, none experienced a drying event. This peak period corresponds to the late dry </p><p>58season and typically receding water levels (see Darby et al. 2008). In a drying event, snails </p><p>59do not move, mate or lay eggs (Darby et al. 2008). Spring dry downs can skew peak egg </p><p>60production toward the summer (i.e., wet) season (O’Hare 2010, Darby 2012 unpublished </p><p>61data). Hanning (1979) reported the incubation period for egg clusters as approximately 16-22</p><p>62days.</p><p>63 Although P. paludosa typically deposit their eggs 10-20 cm above the water line </p><p>64(Turner 1996, Hanning 1979, O’Hare 2010), they can be vulnerable to rising water that </p><p>65results in egg submersion. [Oviposition height can be higher, depending on the vegetation </p><p>66type available (Hanning 1979, Darby unpublished data).] Turner (1998) found that 1- to 8-</p><p>67day old eggs failed to hatch when submerged. Sixty-five percent of 12-day-old (fully </p><p>68hardened and calcified eggs) hatched despite being submerged. Based on egg height data </p><p>69from the Everglades (Darby unpublished data), a water level rise of more than 18 cm would </p><p>70be required to submerge most unhatched egg clusters.</p><p>71 Although a water level rise may not be problematic for snail eggs already deposited, </p><p>72there appears to be a negative effect of relatively deep water on egg cluster production. </p><p>73Gleason et al. (1975) described an inverse relationship between a hydrologic index (average </p><p>74depth*hydroperiod) and snail eggs counted, although snail density estimates, which might </p><p>75have explained at least part of the variation in egg production, were not available. Darby </p><p>5 3 76(unpublished) calculated a per capita egg cluster count (PCE) (number of egg clusters divided</p><p>77by snail densities) and found higher PCE associated with lower depths (as long as depths </p><p>78were greater than approximately 10 cm). Egg cluster production dropped by an order of </p><p>79magnitude, despite relatively abundant snails, in a relatively wet year in WCA3A (2003, </p><p>80where water depths for the year never fell below 40 cm) compared to the previous year </p><p>81(2002) in which the study sites had depths of 10-40 cm during the spring reproductive season </p><p>82(Darby et al. 2005). In addition, the PCE dropped by an order of magnitude from 2002 to </p><p>832003 (Darby, unpublished calculations). The following year (2004) the snail population in </p><p>84this part of WCA3A dropped by over 80% (Darby et al. 2005). Although the depth range </p><p>85best suited for apple snail recruitment requires further study, especially for the upper depth </p><p>86limits (see Pomacea Project 2013), there is sufficient evidence to suggest that a negative </p><p>87influence of some upper depth limit on reproduction should be incorporated into the apple </p><p>88snail population model.</p><p>89 Apple snails hatch out at three to six millimeters in diameter (Hanning 1979, Darby et</p><p>90al. 2008). They grow approximately 10-13 mm per month during the first two months </p><p>91(Hanning 1979, Glass and Darby 2009); therefore, snails that hatch in spring can reach adult </p><p>92size (and sizes consumed by kites) by early to mid-summer. Growth rates slow down </p><p>93considerably once the snails reach ~25 mm (Hanning 1979). Although numerous </p><p>94environmental factors likely influence growth rates, they have not been sufficiently quantified</p><p>95to be parameterized in our model.</p><p>96 The sub-tropical climate of southern Florida results in sufficient temperature </p><p>97fluctuations to significantly influence apple snail activity in general. Stevens et al. (2002) </p><p>98found no activity in apple snails exposed to water temperatures <13°C, and below 10°C 92% </p><p>99of snails burrowed into the sediments. These observations of snails were consistent with </p><p>100Cary (1985), having found no snail captures by kites attempting to forage when air </p><p>6 4 101temperatures fell below 10°C. From 11°C up to 30°C, prey capture rates by kites increased </p><p>102linearly, most likely a function of increased rates of general activity and surfacing by snails </p><p>103(Cary 1985). McClary (1964) reported a 2.7-fold increase in the number of surface </p><p>104inspirations by Pomacea snails as temperatures increased from 12°C to 26°C. Stevens et al. </p><p>105(2002) observed that the greatest increase (nearly exponential) in general activity occurred </p><p>106between 20°C and 22°C degrees. Given the over-riding effect of low temperatures on </p><p>107activity, relatively low dry-season temperatures would be expected to reduce rates of egg </p><p>108cluster production (see Hanning 1979), and this effect may be amplified by a depth-</p><p>109temperature interaction (Pomacea Project 2013).</p><p>110 Several studies have made associations between snail density or relative abundance </p><p>111and attributes of plant community structure (Bryan 1990, Turner 1996, Darby et al. 2004, </p><p>112Karunarante et al. 2006); however, the current version of our apple snail model does not </p><p>113parameterize the complex influence of habitat components (other than water depth) on snail </p><p>114demographic metrics. In laboratory studies, diet (Sharfstein and Steinman 2001, Shuford et </p><p>115al. 2005), and calcium and pH (Glass and Darby 2009) have also been shown to influence P. </p><p>116paludosa growth and survival; however, these parameters have not yet been incorporated into</p><p>117the model.</p><p>118</p><p>119EverSnail Model Description Following Grimm et al. (2006, 2010) ODD protocol</p><p>120Purpose and Parameterization </p><p>121The purpose of EverSnail is to describe the dynamics of the apple snail population on the </p><p>122Everglades landscape over a period of years, in order to interpret effects of year-to-year </p><p>123variability in landscape hydrology on apple snail abundance. In particular, the numbers and </p><p>124size distributions of the snails are simulated by following cohorts of snails through their life </p><p>125cycles, which allows total numbers within any given size range to be calculated for any day </p><p>7 5 126within a year. The effects of scenarios based on historical environmental data, as well as </p><p>127scenarios based on artificially varied sequences of environmental conditions, can be </p><p>128employed in the model to study the effects on apple snail population distributions across the </p><p>129landscape.</p><p>130</p><p>131State variables and scales</p><p>132The age- and size-structured apple snail population is represented as a vector consisting of </p><p>133500 daily age-classes, with 500 d representing the approximate life span of Florida apple </p><p>134snails;</p><p>135 N = [N1(t), N2(t), …, Ni(t), … , N500(t)] (A.1)</p><p>136where Ni(t) is the number of apple snails in age class i in a given spatial cell at time t. The </p><p>137snails in each age class have an attribute of size (linear dimension in mm), which increases </p><p>138deterministically. Space is represented by 253125 spatial cells, each 400 x 400 m, which are </p><p>139contiguous and represent the Water Conservation Areas and ENP (see Figure 1, main text) </p><p>140and some surrounding wetlands. The temporal scale of resolution is one day, and simulations</p><p>141can be run for any period beginning January 1st, 1991 (details on water depth input, </p><p>142Appendix 3). The individual spatial cells are assumed independent (no movement of snails </p><p>143between cells is assumed), so simulations can be performed either on the whole spatial extent,</p><p>144or on individual cells or subsets of cells, such as those for which empirical data on snail </p><p>145densities are available. The analyses presented here emphasized WCA3A (see Figure 1), </p><p>146where the majority of Everglades snail density data has been collected (Pomacea Project </p><p>1472013). </p><p>148</p><p>149Process overview and scheduling</p><p>8 6 150As there is no immigration to or emigration from a spatial cell, the dynamics of the age-</p><p>151structured subpopulation in each spatial cell is entirely governed by a Markov chain matrix </p><p>152model, A, shown below. </p><p>0 0 0 . fi f i1 f i2 . f 499 f500</p><p> a12 0 0 . 0 0 0 . 0 0</p><p>0 a23 0 . 0 0 0 . 0 0 ...... 0 0 0 . 0 0 0 . 0 0 153 A (A.2) 0 0 0 . ai,i1 0 0 . 0 0</p><p>0 0 0 . 0 ai1,i2 0 . 0 0 ...... 0 0 0 . 0 0 0 . 0 0</p><p>0 0 0 . 0 0 0 . a499,500 0</p><p>154</p><p>155where ai,i+1 represents the fractional survival of a given one-day age class cohort from age </p><p>156class i to age class i+1, and fi is fecundity (per capita offspring) of age class i. The number of</p><p>157new 1-day old snails on each day is given by</p><p>158 N1 (t+20) = f iN i (t) + f i+1N i+1(t) … + … f 500 N500(t), (A.3)</p><p>159starting from the first age class capable of reproduction, where the ‘20’ represents a 20-day </p><p>160delay for eggs to hatch. Survival from one age class to the next is given by</p><p>161 Ni+1(t+1) = ai,i+1Ni(t) (i = 1, 2….., 500) (A.4)</p><p>162</p><p>163Initialization </p><p>164At the start of each simulation, an equal number of snails is assumed present in each age class</p><p>165and in all spatial cells. Simulations are run over preliminary periods of several years to allow</p><p>166transient effects of the initialization to disappear, which occurs after about three years.</p><p>167</p><p>168Environmental conditions </p><p>9 7 169We assume a grid of 400 x 400 meter cells covering the area of the simulation. For each cell,</p><p>170we assume that we can assign:</p><p>171 daily values of water depth from Everglades Depth Estimator Network (EDEN)</p><p>172 daily values of ambient air temperature.</p><p>173</p><p>174Daily water depth values for each spatial cell are input each day using data from the </p><p>175Everglades Depth Estimation Network (see Appendix 3 for details). Regional temperature </p><p>176values were linearly interpolated from existing data points across the whole set of spatial cells</p><p>177(Appendix 3). The specific spatial area to be simulated (the whole area or a subset of cells </p><p>178examined for sensitivity analyses) and the number of years simulated are prescribed as input. </p><p>179</p><p>180Submodels</p><p>181There are three basic submodels that determine the simulated snail population; growth, </p><p>182survival, and reproduction, each of which is based on empirical information. The matrix A </p><p>183(equation A.2) contains the elements ai,i+1(t) and fi(t), for age class survival and reproduction, </p><p>184respectively. Both of these elements are functions of sizes of the snails, so the relationship </p><p>185between age and size, or ‘growth’ is first described. Parameter values are given in Table A1-</p><p>1861.</p><p>187</p><p>188Growth. A logistic function is used to describe the mean individual size with age for every </p><p>189snail cohort;</p><p> kgrowth Age Sizemine 190 Sizei , (A.5) kgrowth Age 1 ( Sizemin / Sizemax )( e 1)</p><p>191where Sizemin = linear size of newly hatched snail, Sizemax = maximum size of snail, and </p><p>192kgrowth = daily growth rate (see Figure A1-1). Growth is assumed to follow the pattern of </p><p>10 8 193Equation (A.5) regardless of changing environmental conditions, and thus every cohort </p><p>194follows the same growth pattern. The influence of environmental conditions such as </p><p>195temperature requires additional empirical data on P. paludosa to support further </p><p>196parameterization of the growth component of EverSnail. </p><p>197</p><p>198</p><p>199Survival. The values of day-to-day survival, ai,i+1, depend on snail size. Four survival </p><p>200categories were selected based on empirical data that show distinct survival rates between </p><p>201three small juvenile categories as well as a fourth category that includes juveniles >16 mm </p><p>202and reproductively mature adults. Survival can be divided into two conditions, wet (water </p><p>203depth > 0 cm) and dry (see Table A1-1 and Figure A1-2).</p><p>204</p><p>205 Size Wet conditions Dry conditions</p><p>206 size ≤ 6 mm Survwet1 Survdry1</p><p>207 6 mm ≤ size < 10 mm Survwet2 Survdry2</p><p>208 10 mm ≤ size < 16 mm Survwet3 Survdry3</p><p>209 16 mm ≤ size Survwet4 Survdry4</p><p>210</p><p>211The maximum lifespan of adults does not extend much beyond 500 days. In order to achieve </p><p>212rapid die-off around this age, we substitute the following expressions for mortality of snails </p><p>213of size > 16 mm under wet and dry conditions, respectively.</p><p>kage ( Agemortage) 214 Survadult,wet Survwet 4 /1 e (A.6)</p><p>kage ( Agemortage) 215 Survadult,dry Survdry4 /1 e (A.7)</p><p>216</p><p>11 9 217Reproduction. The values of fi(t), or the numbers of offspring produced per female snail per </p><p>218day, depend on a number of factors specific to the snails and the environmental conditions. </p><p>219We can write fi(t) as a product of factors:</p><p>220 fi(t) = (fraction of snails of age i that are female) </p><p>221 x (fraction of females of age i that are sexually mature) x (clutch size) </p><p>222 x (effects of time of year) x (effects of temperature) x (effects of water depth)</p><p>223 x (effects of egg submersion) (A.8)</p><p>224where ‘egg submersion’ means that the snail eggs are under water for some time, whereas </p><p>225‘effects of water depth’ refer to the effects of water depth independent of submersion of the </p><p>226eggs.</p><p>227We consider each of these reproduction factors in turn.</p><p>228 1. Fraction of snails of age i that are female; we assume Female fraction = 0.5 </p><p>229</p><p>230 2. Fraction of females of age i that are sexually mature. Sexual maturity depends on snail</p><p>231 size, which is calculated as a function of age in equation (A.5). We assume there is a </p><p>232 mean size of arrival at maturity (MaturityThreshold), 27.5 mm, but that there is also </p><p>233 variation about this value, described by the equation</p><p> e krepr (Sizei MaturityThreshold ) 234 Fraction of mature females at agei ’ (A.9) 1 ekrepr (Sizei MaturityThreshold )</p><p>235 where Sizei is the size of females in age class i (see Figure A1-3). A value of krepr = </p><p>236 1.0 will result in virtually all females being sexually mature by Size = 30 mm.</p><p>237</p><p>238 3. Clutch size, Clutch. A clutch size of Clutch = 30 eggs is assumed.</p><p>239</p><p>12 10 240 4. Effects of time of year, f(time of year). There is some seasonality in reproduction for </p><p>241 which we do not yet have a mechanistic explanation. To account for this seasonal </p><p>242 variation, we assume (see Figure A1-4)</p><p>243 f(time of year) = 1.0 Day 15 ≤ time of year < Day 189</p><p>244 f(time of year) = 0.3 Day 190 ≤ time of year < Day 328</p><p>245 f(time of year) = 0.0 otherwise</p><p>246</p><p>247 5. Effects of water depth, f(water depth). Apple snails do not reproduce when water </p><p>248 depth is too low, because of difficulty in moving. High water depths also inhibit </p><p>249 reproduction. We represent the effects of water depth by a parabolic function between</p><p>250 upper and lower bounds, Depthmin and Depthmax, respectively, with a midpoint </p><p>251 Depthmid (see Figure A1-5),</p><p>252 f(water depth) = 0.0 water depth < Depthmin</p><p>2 2 253 f(water depth) = 1.0 - (water depth –Depthmid ) /Wh Depthmin ≤ water depth<Depthmax</p><p>254 f(water depth) = 0.0 Depthmax ≤ water depth </p><p>255</p><p>256 6. Effects of temperature on reproduction. The probability of reproduction decreases </p><p>257 rapidly as temperature decreases below 17° C and reaches a maximum (plateau) at </p><p>258 21° C and above. We can represent this as, f(temperature) (see Figure A1-6)</p><p>1 259 f(temperature) = (A.10) 1 e ktemp ( TemperatureTempraturethreshold )</p><p>260</p><p>261 7. Effects of egg submersion, f(egg submersion). Note that this is different from #5 </p><p>262 ‘Effects of water depth’, which does not take into account egg submersion. Eggs may </p><p>263 die if submersed for some period following oviposition during the time of 20 days that</p><p>264 they take to hatch. The egg clusters are oviposited about 18 cm above the water level.</p><p>13 11 265 Rule 1. If they are not submersed, they will hatch within 20 days, with the assumption</p><p>266 that 5% of the original number of eggs hatch each day, such that 100% hatch after 20 </p><p>267 days. Rule 2. If the eggs are submersed for longer than 14 days, then only 5% of the </p><p>268 remaining unhatched eggs survive to hatching. It is assumed in the model that all </p><p>269 surviving eggs hatch in the model 20 days after the egg cluster is oviposited.</p><p>270</p><p>271Carrying capacity. A carrying capacity is imposed on the population of cells in any given </p><p>272cell by assuming there is an upper limit on the number of eggs that can exist on a given day, </p><p>273as reproductive sites are limited to emergent vegetation. This acts as the ‘density-dependent’ </p><p>274regulation in the model. The upper limit on total eggs at a given time is assumed to be</p><p>-1 275 Egglimit = 35,000 ha </p><p>276If this limit is reached, no more eggs are assumed to be oviposited. It is possible that this </p><p>277limit will not be reached under realistic conditions.</p><p>278</p><p>279References</p><p>280Bryan DC (1990) Apple snail densities at Alexander Springs, Lake County, and observations </p><p>281 on snail ecology. Fla Sci 53:13 </p><p>282Cary DM (1985) Climatological factors affecting the foraging behavior and ecology of snail </p><p>283 kites (Rostrhamus sociabilis plumbeus Ridgway) in Florida. Thesis. University of Miami, </p><p>284 Florida</p><p>285Cowie RH (2002) Apple snail (Ampullariidae) as agricultural pests: their biology, impacts, </p><p>286 and management In Barker GM (ed) Molluscs as Crop Pests. CABI Publishing, </p><p>287 Wallingford, UK, pp 145-192 </p><p>288Darby PC, Valentine-Darby PL, Percival HF (2003) Dry season survival in a Florida apple </p><p>289 snail (Pomacea paludosa Say) population. Malacologia 45:179-184 </p><p>14 12 290Darby PC, Valentine-Darby PL, Percival HF, Kitchens WM (2004) Florida apple snail </p><p>291 (Pomacea paludosa Say) responses to lake habitat restoration activity. Arch Hydrobiol </p><p>292 161:561-575 </p><p>293Darby PC, Karunaratne LB, Bennetts RE (2005) The influence of hydrology and associated </p><p>294 habitat structure on spatial and temporal patterns of apple snail abundance and </p><p>295 recruitment. Final Report to U.S. Geological Survey. University of West Florida, </p><p>296 Pensacola </p><p>297Darby PC, Bennetts RE, Percival HF (2008) Dry down impacts on apple snail (Pomacea </p><p>298 paludosa) demography: Implications for wetland water management. Wetlands 28:204-</p><p>299 214 </p><p>300Ferrer JR, Perera G, Yong M (1990) Life tables of Pomacea paludosa (say) in natural </p><p>301 conditions. Fla Sci 53 (supplement):15 </p><p>302Glass NH, Darby PC (2009) The effect of calcium and pH on Florida apple snail Pomacea </p><p>303 paludosa (Gastropoda: Ampullariidae) shell growth and crush weight. Aquat Ecol </p><p>304 43:1085-1093 </p><p>305Gleason PJ, Stone PA, Rhoads P, Davis SM, Zaffke M, Harris L (1975) The impact of </p><p>306 agricultural runoff on the everglades marsh located in the conservation areas of the </p><p>307 central and southern Florida flood control district. South Florida Water Management </p><p>308 District, West Palm Beach</p><p>309Grimm V, Berger U, Bastiansen F, Eliassen S, Ginot V, Giske J, Goss-Custard J, Grand T, </p><p>310 Heinz SK, Huse G, Huth A, Jepsen JU, Jørgensen C, Mooij WM, Müller B, Pe΄er G, Piou</p><p>311 C, Railsback SF, Robbins AM, Robbins MM, Rossmanith E, Rüger N, Strand E, Souissi </p><p>312 S, Stillman RA, Vabø R, Visser U, DeAngelis DL (2006) A standard protocol for </p><p>313 describing individual-based and agent-based models. Ecol Model 198:115-126</p><p>15 13 314Grimm V, Berger U, DeAngelis DL, Polhill JG, Giske J, Railsback SF (2010) The ODD </p><p>315 protocol: A review and first update. Ecol Model 221:2760-2768</p><p>316Hanning GW (1979) Aspects of reproduction in Pomacea paludosa (Mesogastropods: </p><p>317 Pillidae). Thesis, University of Florida, Gainesville</p><p>318Hurdle MT (1974) Life history studies and habitat requirements of the apple snail at Lake </p><p>319 Woodruff National Wildlife Refuge. Proc Ann Conf Southeastern Assoc Game Fish </p><p>320 Commissioners 27:215-224 </p><p>321Karunaratne LB, Darby PC, Bennetts RE (2006) The effects of wetland habitat structure on </p><p>322 Florida apple snail density. Wetlands 26:1143-1150 </p><p>323Kushlan JA (1990) Freshwater marshes. In: Myers RL, Ewel JJ (eds) Ecosystems of Florida. </p><p>324 University of Central Florida Press, Orlando, pp 324-363</p><p>325McClary A (1964) Surface inspiration and ciliary feeding in Pomacea paludosa </p><p>326 (Prosobrachia: Mesogastropoda: Ampullariidae). Malacologia 2:87-104</p><p>327O’Hare NK (2010) Pomacea paludosa (Florida apple snail) reproduction in restored and </p><p>328 natural seasonal wetlands in the Everglades. Wetlands 30:1045-1052 </p><p>329Pomacea Project, Inc. (2013) Literature review of Florida apple snails and snail kites, and </p><p>330 recommendations for their adaptive management. Final Report. Submitted to the </p><p>331 National Park Service, Everglades National Park. Available from </p><p>332 http://www.nps.gov/ever/naturescience/upload/ASS10-3FinalReportSecure.pdf. Accessed</p><p>333 22 June 2014.</p><p>334Sharfstein B, Steinman AD (2001) Growth and survival of the Florida apple snail (Pomacea </p><p>335 paludosa) fed 3 naturally occurring macrophyte assemblages. J North Am Benthol Soc </p><p>336 20:84-95</p><p>337Shuford III RBE, McCormick PV, Magson J (2005) Habitat related growth of juvenile </p><p>338 Florida applesnails (Pomacea Paludosa). Fla Sci 68:11-19 </p><p>16 14 339Stevens AJ, Welch ZC, Darby PC, Percival HF (2002) Temperature effects on Florida </p><p>340 applesnail activity: Implications for snail kite foraging success and distribution. Wildl Soc</p><p>341 Bull 30:75-81 </p><p>342Turner RL (1996) Use of stems of emergent plants for oviposition by the Florida applesnail, </p><p>343 Pomacea paludosa, and implications for marsh management. Fla Sci 59:34-49</p><p>344Turner RL (1998) Effects of submergence on embryonic survival and development rate of the</p><p>345 Florida applesnail, Pomacea paludosa: Implications for egg predation and marsh </p><p>346 management. Fla Sci 61:118-129</p><p>347</p><p>17 15 348Table A1-1. Life history parameters and their baseline values </p><p>349______</p><p>350Sizemin = 3 mm</p><p>351Sizemax = 50 mm</p><p>352kgrowth = 0.05</p><p>-1 353Survwet1 = 0.987 day</p><p>-1 354Survwet2 = 0.987 day</p><p>-1 355Survwet3 = 0.987 day</p><p>356Survwet4 = 0.99 </p><p>-1 357Survdry1 = 0.976 day</p><p>-1 358Survdry2 = 0.984 day</p><p>-1 359Survdry3 = 0.989 day</p><p>-1 360Survdry4 = 0.99 day </p><p>361Agemort = 500. day</p><p>-1 362kage = 0.1 day</p><p>363MaturityThreshold = 27.5 mm</p><p>364krepr = 1.0</p><p>365Clutch = 30</p><p>366Depthmid = 50 cm</p><p>367Wh = 40 cm</p><p>368Depthmin = 10 cm</p><p>369Depthmax = 90. cm.</p><p>370ktemp = 1.0 per º C</p><p>371Temperaturethreshold = 17°C</p><p>-1 372Egglimit = 35,000 ha </p><p>18 16 373Appendix 1 Figure Captions </p><p>374</p><p>375Figure A1-1. Snail size versus age in days (Equation A1).</p><p>376Figure A1-2. Daily survival rate as a function of snail size for both wet and dry conditions.</p><p>377Figure A1-3. Cumulative fraction of sexually mature snails, which is used to determine the </p><p>378fraction of snails of a given age class that are mature.</p><p>379Figure A1-4. Maximum possible rate of reproduction as a function of time of year.</p><p>380Figure A1-5. Effect of water depth on reproduction.</p><p>381Figure A1-6. Affect of temperature on snail reproduction (Equation A6).</p><p>19 17 382</p><p>383</p><p>384 385Figure A1-1. Snail size versus age in days (Equation A1)</p><p>386</p><p>387</p><p>388</p><p>389</p><p>390</p><p>391</p><p>392</p><p>393</p><p>394</p><p>395</p><p>20 18 396</p><p>397</p><p>398 399</p><p>400Figure A1-2. Daily survival rate as a function of snail size for both wet and dry conditions.</p><p>401</p><p>402</p><p>403</p><p>404</p><p>405</p><p>406</p><p>407</p><p>408</p><p>409</p><p>410</p><p>411</p><p>21 19 412</p><p>413Figure A1-3. Cumulative fraction of sexually mature snails, which is used to determine the </p><p>414fraction of snails of a given age class that are mature.</p><p>415</p><p>416</p><p>417</p><p>418</p><p>419</p><p>420</p><p>421</p><p>422</p><p>423</p><p>424</p><p>425</p><p>22 20 426</p><p>427 428Figure A1-4. Maximum possible rate of reproduction as a function of time of year.</p><p>429</p><p>430</p><p>431</p><p>432</p><p>433</p><p>434</p><p>435</p><p>436</p><p>437</p><p>438</p><p>439</p><p>440</p><p>23 21 441</p><p>24 22 443Figure A1-5. Effect of water depth on reproduction.</p><p>444</p><p>445</p><p>446</p><p>447</p><p>448</p><p>449</p><p>450</p><p>451</p><p>452</p><p>453</p><p>454</p><p>455</p><p>456</p><p>25 23 457Figure A1-6. Affect of temperature on snail reproduction (Equation A6).</p><p>458</p><p>459</p><p>460</p><p>26 24 461Appendix 2. EverSnail programming and file formats</p><p>462</p><p>463In order to facilitate parameterization and execution of simulations, a software program </p><p>464encapsulating the apple snail population model logic was developed in the Java™ </p><p>465programming language. The software operates against water depth and temperature inputs </p><p>466that are stored in the Network Common Data Form (NetCDF) file format and conform to the </p><p>467Comprehensive Everglades Restoration Plan (CERP) NetCDF Metadata Conventions (JEM </p><p>4682011). Each input must be organized as a regular grid of cells in three dimensions: 2D </p><p>469coordinate space plus time. A graphical user interface allows users to supply the location of </p><p>470the inputs on the file system and the simulation time period, as well as adjust any of 25 model</p><p>471parameters to support model validation and sensitivity analysis. All selections made by the </p><p>472user are stored in an internal data structure which is accessed by the model logic during </p><p>473runtime. Output is a CERP conventions-compliant NetCDF file containing daily simulated </p><p>474population values per spatial cell for total snails (adults plus juveniles), adults only, juveniles </p><p>475only, and eggs. These data were then analyzed both graphically, by using the EverVIEW </p><p>476Data Viewer (Romañach et al. 2014), and in tabular format with Microsoft Excel.</p><p>477</p><p>478References</p><p>479Romañach SS, McKelvy M, Conzelmann C, Suir K (2014) EverVIEW Data Viewer: A </p><p>480 visualization framework and exploration tool for decision support of large-scale </p><p>481 biological planning. Environ Model Software 62:221-229 </p><p>482 doi:10.1016/j.envsoft.2014.09.008</p><p>483JEM (Joint Ecosystem Modeling) (2011) CERP NetCDF standard. Available from </p><p>484 http://www.jem.gov/Standards/NetCDF. Accessed 30 June 2014</p><p>485</p><p>27 25 486Appendix 3. Detailed description of the air temperature and EDEN water depth inputs </p><p>487to EverSnail </p><p>488</p><p>489The two input variables to EverSnail are daily average air temperature (°C) and estimated </p><p>490daily water depths from the Everglades Depth Estimation Network (EDEN). The spatial </p><p>491extent of the EverSnail model is dictated by the EDEN coverage. The Everglades Depth </p><p>492Estimation Network (EDEN) is a system more than 250 real-time water level monitoring </p><p>493stations throughout the Greater Everglades landscape. Water level data are combined with a </p><p>494ground surface elevation model to create a water surface simulation model. Water surface </p><p>495data are available from 1991 to present for the entire freshwater Everglades region. Data can </p><p>496be downloaded from http://sofia.usgs.gov/eden/models/watersurfacemod_download.php. </p><p>497EDEN daily water depth estimates can be manipulated to create artificial sequences of </p><p>498hydrologic conditions using the Slice and Dice tool available at </p><p>499http://jem.gov/Modeling/SliceAndDice. The South Florida Water Management District, </p><p>500south Florida’s water governing authority, has been collecting surface water, ground water, </p><p>501and meteorological data for many decades. For this model, we used daily air temperature </p><p>502values from 16 stations located throughout the model domain (applicable temperature data </p><p>503are presented in the main manuscript) dating back to 1991. Data can be downloaded from </p><p>504http://www.sfwmd.gov/dbhydroplsql/show_dbkey_info.main_menu. </p><p>505 Intra- and inter-annual fluctuations in water depths drive the observed changes in the </p><p>506EverSnail population output. Temperature influences were also evident, in EverSnail they </p><p>507impact reproduction (especially at lower temperatures), with applicable details presented in </p><p>508the main manuscript. The primary areas of interest for examining hydrologic influences on </p><p>509apple snail populations, as presented in the manuscript, are Water Conservation Area 3A </p><p>510(WCA3A), WCA3B, and northern portions of Everglades National Park (ENP) (see Figure 1,</p><p>28 26 511main manuscript, and Figure A3-1, below). In order to characterize hydrologic impacts </p><p>512described in the manuscript, we obtained EDEN data for representative sites. WCA3A has a </p><p>513hydrologic gradient represented by Sites 13 (lower ground elevation, southern WCA3A) and </p><p>51418 (higher elevations further north), and one site each was selected for comparison in </p><p>515WCA3B and ENP (Figure A3-1). </p><p>516 For the Everglades region, the driest year for the period of interest (2000-2004 </p><p>517hydrology, which influenced 2001-2005 snail abundance, see main manuscript) occurred in </p><p>5182001. In order to represent low water and high water extremes for the period of interest, we </p><p>519focused on WCA3A, but considered conditions for adjoining wetlands, WCA3B and ENP, as </p><p>520well (Figure A3-1). In 2001, portions of WCA3A, WCA3B and ENP experienced dry </p><p>521conditions (Figures A3-2, A3-3 and A3-4). For DrySim we copied the 2001 hydrologic data </p><p>522into simulation years 2002 and 2003 to artificially create 3 consecutive low water years. For </p><p>523WetSim we copied the 2003 hydrologic data into simulation years 2001 and 2002 to </p><p>524artificially create 3 consecutive high water years. Note that 2003 exhibited the highest dry </p><p>525season (winter-spring) water depths for the period of interest (Figures A3-2, A3-3, A3-4). </p><p>29 27 527Appendix 3 Figure Headings</p><p>528</p><p>529Figure A3-1. Everglades sites (*) for which daily water depths for 2000-2004 are presented </p><p>530in Figures A3-2, A3-3 and A3-4. Wetland units (as labeled in main text Figure 1) are </p><p>531L=WCA3A (showing sites 13 and 18), M=WCA3B (one site) and N=ENP (one site). </p><p>532</p><p>533Figure A3-2. WCA3A sites 13 (black diamonds) and 18 (white circles, higher ground </p><p>534elevation) water depths from 2000-2004. 2001 conditions are noted as being used to create 3 </p><p>535consecutive low water years for DrySim and 2003 conditions are noted as being used to </p><p>536create 3 consecutive high water years for WetSim. For site locations see Figure A3-1. </p><p>537Dashed horizontal line represents the depth (10 cm) below which Florida apple snails stop </p><p>538moving, mating or laying eggs (see Appendix 1).</p><p>539</p><p>540Figure A3-3. WCA3B site water depths from 2000-2004. 2001 conditions are noted as being</p><p>541used to create 3 consecutive low water years for DrySim and 2003 conditions are noted as </p><p>542being used to create 3 consecutive high water years for WetSim. For site locations see Figure</p><p>543A3-1. Dashed horizontal line represents the depth (10 cm) below which Florida apple snails </p><p>544stop moving, mating or laying eggs (see Appendix 1).</p><p>545</p><p>546Figure A3-4. ENP site water depths from 2000-2004. 2001 conditions are noted as being </p><p>547used to create 3 consecutive low water years for DrySim and 2003 conditions are noted as </p><p>548being used to create 3 consecutive high water years for WetSim. For site locations see Figure</p><p>549A3-1. Dashed horizontal line represents the depth (10 cm) below which Florida apple snails </p><p>550stop moving, mating or laying eggs (see Appendix 1).</p><p>30 28 551Figure A3-1. </p><p>552</p><p>* *</p><p>553</p><p>31 29 554Fig A3-2. </p><p>555</p><p>120 2000 2001 2002 2003 2004 (used in DrySim) (used in WetSim) 100 ) 80 m c (</p><p> h</p><p> t 60 p e D</p><p>40 r e t a 20 W</p><p>0 Site 13 WCA3A Site 18 -20 556</p><p>32 30 557Fig A3-3.</p><p>558</p><p>100</p><p>2000 2001 2002 2003 2004 (used in DrySim) (used in WetSim) 80</p><p>60</p><p>40</p><p>20</p><p>0</p><p>-20</p><p>-40 WCA3B</p><p>559 -60</p><p>33 31 560Fig A3-4.</p><p>561</p><p>80 2000 2001 2002 2003 2004 (used in DrySim) (used in WetSim)</p><p>60 )</p><p> m 40 c (</p><p> h t</p><p> p 20 e D</p><p> r e t 0 a W</p><p>-20</p><p>ENP</p><p>562 -40</p><p>34 32 563Appendix 4. Detailed results of EverSnail sensitivity analyses</p><p>564</p><p>-1 -1 565The model was very sensitive to changing Survwet4 from 0.99 d down to 0.988 d ; this </p><p>566resulted in the snail population quickly falling to zero (Table A4-1). Increasing Survwet4 to </p><p>5670.992 d-1 had no effect on the snail population. Sensitivity for all the remaining parameter </p><p>568changes ranged from −62.0% to +5.5%, and in some cases there were marked differences in </p><p>569sensitivity between sites (13 and 18) for the same parameter (Table A4-1). Sensitivity was </p><p>570less than ±10% of baseline snail abundance for kgrowth, ktemp, Survwet1, Survwet2, Survwet3. A 20% </p><p>571decrease in Tempthreshold (down to 13.6 °C) resulted in <6% increase in the population (Table </p><p>572A4-1).</p><p>573 A +20% increase in Tempthreshold (up to 20.4 °C) resulted in ~≈20-22% decrease, based </p><p>574on quarterly averages, in the snail population. This population decline resulted from the input</p><p>575temperatures having to rise to a higher level to stimulate reproduction. Very small declines in</p><p>576air temperature below a threshold set at 20.4 °C (+20% of baseline) resulted in substantial </p><p>577changes in sensitivity (Figure A4-1). Changing Depthmax for reproduction from 90 cm </p><p>578(baseline) down to 72 cm (−20% of baseline) resulted in significant changes to snail </p><p>579abundance, but varied with topography. Parameterizing the model to prevent snails from </p><p>580reproducing at depths >72 cm greatly constrained egg production in the breeding season. The </p><p>581relative impact of setting Depthmax to 72 cm across a depth gradient was quantified by </p><p>582comparing output from WCA3A Site 13 vs 18; for site 13 (lower ground elevation) the </p><p>583EDEN depth data more frequently exceeded 72 cm and resulted in a population decline of </p><p>584−62%, compared to Site 18 at −12% (Figure A4-2). Due to overall lower depths in Site 18, </p><p>585the negative influence of lowering Depthmax was much less, compared to Site 13, for the site-</p><p>586specific snail population. At only one point in time did mean quarterly depth in Site 18 </p><p>587exceed 72 cm (in 2005), and this resulted in a significant negative effect on the snail </p><p>35 33 588population (−51% relative to the baseline) (Figure A4-2). The fact that raising Depthmax to </p><p>589108 cm resulted in no change in the population relative to baseline (Table A4-1) reflects the </p><p>590fact that quarterly mean depths in Sites 13 and 18 never exceeded 108 cm, and at this setting </p><p>591the simulated snail population continued to produce eggs. When EDEN depth inputs stayed </p><p>592between 10 and 90 cm under baseline conditions the population remained relatively stable </p><p>593and the annual cycle was driven by seasonal variation in egg cluster production (see main </p><p>594text).</p><p>595 Since a change in Depthmax for reproduction resulted in a significant impact in the </p><p>596sensitivity analyses, and since Everglades restoration and management focuses primarily on </p><p>597restoring certain hydrologic conditions, we will elaborate on the response of the population to</p><p>598changes in seasonal depths. The overall lowest water depths (falling below 10 cm in Site 18) </p><p>599were observed in 2001 during the dry season (Figure A4-2, also see Appendix 3). The </p><p>600highest dry-season water depths were observed in 2003; during that year depths stayed above </p><p>60125 cm in site 18 and in site 13 depths never fell below 59 cm. For any given year, the </p><p>602greatest depths occurred in the fall, and 2005 fall depths were the greatest overall (Figure A4-</p><p>6032). Generally lower Site 18 water depths reflect a higher ground elevation than Site 13 (for </p><p>60475% of the simulation days, depths were ~20-40 cm lower than site 13, see Appendix 3). </p><p>605These differences in water depth differentially impacted the sensitivity to lowering the </p><p>606Depthmax for reproduction down to 72 cm. From 2001-2005, Site 18 depths exceeded 72 cm </p><p>607only once in 20 quarters, so sensitivity was <20% the majority of this period. In contrast, </p><p>608Site 13 depths exceeded 72 cm one to two quarters each year, and sensitivity ranged from </p><p>609-29% to -86%. The relationship between egg cluster production and seasonal water depth, </p><p>610represented here as Depthmax, needs to be addressed with more empirical data (see Discussion,</p><p>611main text), since the value of this parameter in EverSnail has a substantial impact on </p><p>612predicted snail abundance over the Everglades landscape (Figure A4-3).</p><p>36 34 613Table A4-1. EverSnail sensitivity expressed as percent change in total snail density when </p><p>614comparing simulations set at baseline parameter values to those with -20% and +20% </p><p>615changes for four model parameters shown, and a change of ±0.002 for daily survival rate (for </p><p>616four size categories) in flooded conditions (equivalent to ±20% of the mortality rate). Sites </p><p>61713 and 18 are shown to illustrate differences as a function of location within WCA3A (i.e., </p><p>618site 13 has a lower ground elevation). The mean snail density was calculated for each quarter</p><p>619of the simulation period (2001-2005), with the standard deviation (SD) (n= 20 total quarterly </p><p>620calculations). </p><p>621 622 Quarterly Quarterly</p><p>Baseline % change % change</p><p>Parameter Snail density Snail density</p><p>Parameter* Value Site w/-20% BPV w/+20% BPV</p><p>(submodel) (BPV) Mean ± SD (n=20) Mean ± SD (n=20)</p><p> kgrowth (size) 0.05 13 -4.2 ± 2.7 1.6 ± 1.5</p><p>18 -3.1 ± 2.1 0.6 ± 1.6</p><p> ktemp (reproduction) -1.0 / °C 13 1.1 ± 1.0 -0.2 ± 0.80</p><p>18 0.2 ± 1.0 -1.3 ± 1.0</p><p>Tempthreshold 17 °C 13 5.5 ± 7.6 -21.7 ± 15.7</p><p>(reproduction) 18 2.5 ± 3.4 -19.9 ± 12.1</p><p>Depthmax 90 cm 13 -62.0 ± 17.1 0.0 ± 0.0</p><p>(reproduction) 18 -12.1 ± 12.0 0.0 ± 0.0 w/-0.002 w/+0.002 (survival) (survival)</p><p>Survwet1 0.987 / d 13 -1.4 ± 1.0 1.8 ± 0.9</p><p>(survival) 18 -2.1 ± 1.3 1.3 ± 1.6</p><p>Survwet2 0.987 / d 13 -1.1 ± 0.6 1.7 ± 1.0</p><p>(survival) 18 -1.9 ± 1.4 0.9 ± 1.0</p><p>Survwet3 0.987 / d 13 -1.0 ± 0.6 1.6 ± 1.0</p><p>(survival) 18 -1.9 ± 1.4 0.9 ± 1.0</p><p>Survwet4 0.990 / d 13 -100 ± 0.0 0.0 ± 0.0</p><p>(survival) 18 -100 ± 0.1 0.0 ± 0.0 37 35 623*See Appendix 1 for description of parameters</p><p>624</p><p>625</p><p>626</p><p>627</p><p>628</p><p>629</p><p>630</p><p>631</p><p>632</p><p>633</p><p>634</p><p>635</p><p>636</p><p>38 36 637Appendix 4 Figure Headings</p><p>638</p><p>639Figure A4-1. Variation in model sensitivity through the simulation period when the </p><p>640Tempthreshold (for reproduction) was set at +20% of baseline, expressed on a quarterly basis for </p><p>641simulation years 2001-2005. Solid black line represents the average quarterly deviation in </p><p>642snail abundance from the baseline abundance after Tempthreshold was set at +20%. Dashed-gray</p><p>643horizontal line represents 20.4 °C (+20% of the baseline 17 °C) in order to reference when </p><p>644input data fall below this temperature threshold, which yielded the greatest percent deviation </p><p>645in snail abundance. Temperature data used as input for the model (solid gray line) are shown </p><p>646for Site 18 (sensitivity results were very similar for Site 13, not shown).</p><p>647</p><p>648Figure A4-2. Variation in model sensitivity to Depthmax (for reproduction) expressed on a </p><p>649quarterly basis for simulation years 2001-2005. Solid black lines represent the average </p><p>650quarterly deviation in snail abundance from the baseline abundance after Depthmax was set at </p><p>651minus 20%. Dashed-gray horizontal lines represent 72 cm (-20% of the baseline 90 cm) in </p><p>652order to reference when input data fall below this depth threshold. EDEN-estimated depths </p><p>653(solid gray line) shown for Site 18 and Site 13 (the latter having a lower ground elevation).</p><p>654</p><p>655Figure A4-3. Landscape level output of the simulated Everglades total snail population </p><p>656associated with sensitivity analyses that lowered Depth Max for reproduction from the </p><p>657baseline setting of 90 cm (panel a) down to 72 cm (-20% of baseline) (panel b). The output is</p><p>658for February 1st of 2004, which reflects a snail population exposed to relatively high water </p><p>659levels (Appendix 3) in the 2003 breeding season.</p><p>660</p><p>39 37 661Fig A4-1. </p><p>-60.0 30.0</p><p>-50.0 25.0 e c</p><p> n -40.0 a</p><p> d 20.0 ) n u C ( b</p><p> e A -30.0</p><p> r l i u t a a</p><p> n 15.0 r S e</p><p> p n</p><p> i -20.0</p><p> m e e g T</p><p> n 10.0 a</p><p> h -10.0 C</p><p>% 5.0 0.0 Q1 Q2 Q3 Q4 Q1 Q2 Q3 Q4 Q1 Q2 Q3 Q4 Q1 Q2 Q3 Q4 Q1 Q2 Q3 Q4 2001 2002 2003 2004 2005 10.0 0.0 662</p><p>663</p><p>664</p><p>665</p><p>40 38 666 fig A4-2.</p><p>-100.0 100</p><p>Site 18 90 -80.0</p><p> e 80 c n a</p><p> d 70 n -60.0 u b A</p><p>60 ) l i m a c n (</p><p>S</p><p>-40.0 50 h t n i p e e g D</p><p> n 40 a h -20.0 C 30 %</p><p>20 0.0 Q1 Q2 Q3 Q4 Q1 Q2 Q3 Q4 Q1 Q2 Q3 Q4 Q1 Q2 Q3 Q4 Q1 Q2 Q3 Q4 10 2001 2002 2003 2004 2005 20.0 0 667</p><p>668 -100.0 100</p><p>Site 13 90 -80.0 80</p><p> e c</p><p> n 70 a</p><p> d -60.0 n u )</p><p> b 60 A m</p><p> l c i (</p><p> a</p><p>-40.0 50 h n t S p</p><p> e n i</p><p>40 D e g</p><p> n -20.0 a</p><p> h 30 c</p><p>% Q1 Q2 Q3 Q4 Q1 Q2 Q3 Q4 Q1 Q2 Q3 Q4 Q1 Q2 Q3 Q4 Q1 Q2 Q3 Q4 20 0.0 2001 2002 2003 2004 2005 10</p><p>20.0 0 669</p><p>41 39 670FigA4-3</p><p>671 Panel a. (depthmax=90) Panel b. (depthmax=72)</p><p>672</p><p>42 40</p>
Details
-
File Typepdf
-
Upload Time-
-
Content LanguagesEnglish
-
Upload UserAnonymous/Not logged-in
-
File Pages40 Page
-
File Size-