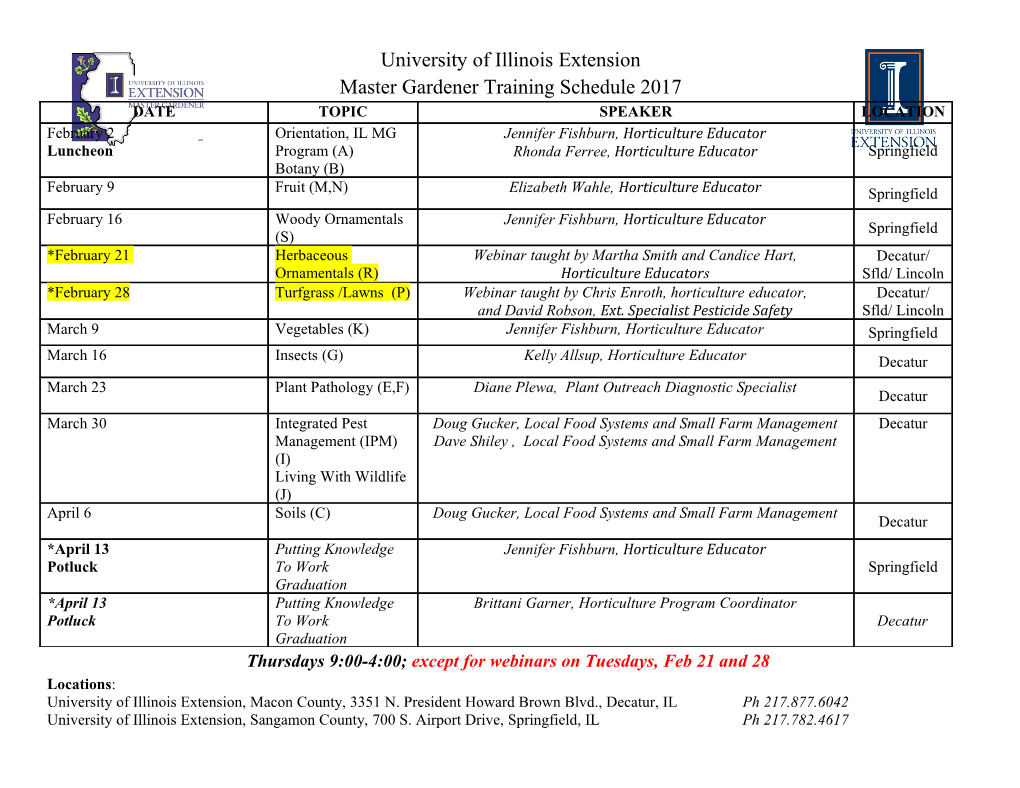
<p>Chapter 4 – atomic structure Atom – smallest particle w/all the properties of an element - electron, proton, neutron - empty space - electrically neutral in normal state Ionization – removal of an orbital electron from an atom No outer shell can contain more than 8 electrons Max electrons per shell = 2n2, n = shell number Centripetal force keeps an electron in its orbit Atomic mass number does not equal the precise mass of an atom Isotopes Same atomic number but different atomic mass Isobar Atomic nuclei with same atomic mass but different atomic numbers Isotone Atomic nuclei with same number of neutrons but different numbers of protons Isomer Atomic nuclei with same atomic number and same atomic mass number Molecule Combination of various elements Compound Any quantity of one type of molecule Radioactivity Emission of particles and energy in order to become stable Radioactive Decay Results in emission of alpha particles, beta particles, and gamma rays Half-Life Time required for a quantity of radioactivity to be reduced to ½ of its original value Radioactive Decay Activity Remaining = Original Activity (0.5)n, n = number of half lives Alpha Particle Helium nucleus containing 2 protons and 2 neutrons Beta Particle Electron emitted from the nucleus of a radioactive atom Chapter 5 – electromagnetic spectrum, radiologically important photons X-ray photon is a quantum of electromagnetic radiation EMR velocity is 3x108 m/s Amplitude ½ the range from crest to valley over which the sine wave varies Frequency Number of wavelengths passing a point of observation per second At a given velocity, wavelength and frequency are inversely proportional Wave Equation Wavelength = Velocity/Frequency λ = c/f Electromagnetic Wave Equation Velocity = Frequency x Wavelength c = f λ Electromagnetic Wave Equation f = c/ λ and λ = c/f The electromagnetic spectrum includes the entire range of electromagnetic radiation Diagnostic ultrasound is not part of the electromagnetic spectrum The energy of a photon is directly proportional to its frequency The only difference between x-ray and gamma ray is origin Visible light is identified by wavelength, RF is identified by frequency, and x-rays are identified by energy Photons interact with matter most easily when the matter is approximately the same size as the photon wavelength X-rays behave like particles Visible light behaves like a wave Radiation attenuation is the reduction in intensity resulting from scatter and absorption Inverse Square Law 1 2 2 2 I /I = d2 /d1 Radiation intensity is inversely related to the square of the distance from the source The inverse square law is applicable to 7 times the longest dimension of the source The x-ray photon is a discrete bundle of energy Planck’s Quantum Equation E = hf Where E = photon energy, h = Planck’s constant, f = photon frequency in hertz The energy of a photon is directly proportional to its frequency Equivalent Planck Equation E = hf, f = E/h, E = hc/ λ Relativity E = mc2 Where E = energy in joules, m = mass in kg, c = light in m/s Chapter 8 – x-ray machine Autotransformer Has a single winding and is designed to supply a precise voltage to the filament circuit and to the high voltage circuit of the e-ray imaging system Autotransformer Law Vs/Vp = Ns/Np Where Vp = primary voltage, Vs = secondary voltage, Np = number of primary windings, Ns = number of secondary windings kVp determines the quality of the x-ray beam Thermionic Emission Release of electrons from a heated element Product of x-ray tube current (mA) and exposure (s) is mAs, which is also electrostatic charge © mAs times are used on falling-load and capacitor discharge imaging systems High Voltage Generator Contains 3 primary parts: high-voltage transformer, filament transformer, and rectifiers Rectification is the process of converting AC to DC Voltage rectification is required to ensure that electrons flow from cathode to anode only With 3-phase power, the voltage impressed across the x-ray tube is nearly constant, never dropping to zero during exposure Full-wave rectification or high-frequency voltage generation is used in almost all stationary x-ray systems During capacitor discharge, the voltage falls approximately 1kV/mAs Less voltage ripple results in higher radiation quality an dquantity Power = Current x Potential Watts = Amperes x Volts High voltage generator power (kW) = maximum x-ray tube current (mA) at 100 kVp and 100 ms Power Rating (kW) = mA x kVp for high frequency and 3-phase 1000 Power Rating (kW) = 0.7 x mA x kVp for single phase 1000 Chapter 9 – x-ray machine The protective housing guards against excessive radiation exposure and electrical shock X-ray tubes are designed w/either a glass or metal enclosure The cathode is the negative side of the x-ray tube and has two primary parts: a filament and a focusing cup Tungsten vaporization with deposition on the inside of the glass enclosure is the most common cause of tube failure The x-ray tube current is adjusted by controlling the filament current Thermionic emission at low kVp and high mA can be space-charge limited The anode is the positive side of the x-ray tube; it conducts electricity and radiates heat and contains the target Higher tube currents and shorter exposure times are possible with rotating diode The rotating anode is powered by an electromagnet induction motor The focal spot is the actual x-ray source The line focus principle results in an effective focal spot size much less than the actual focal spot size The smaller the anode angle, the larger is the heel effect The hell effect results in smaller effective focal spot and less radiation intensity on the anode side of the x-ray beam Excessive heat results in reduced x-ray tube life Maximum radiographic techniques should never be applied to a cold anode The most frequent cause of abrupt tube failure is electron arcing from filament to enclosure due to vaporized tungsten Single Phase Heat Units HU = kVp x mA x s 3 Phase/High Frequency Heat Units HU = 1.4 x kVp x mA x s Chapter 10 – x-ray production Kinetic energy is energy of motion Kinetic Energy KE = ½ mv2 Where m = mass in kg, v = velocity in m/s, KE in joules Approximately 99% of the KE of projectile electrons is converted to heat Characteristic x-rays are emitted when an outer shell electron fills an inner shell void Only the K-characteristic x-rays of tungsten are useful for imaging This type of radiation is called characteristic because it is characteristic of the target element Bremsstrahlung x-rays are produced when a projectile electron is slowed by the electric field of a target atom nucleus In the diagnostic range, most x-rays are bremsstrahlung x-rays A discrete spectrum contains only specific values A continuous spectrum contains all possible values Characteristic x-rays have precisely fixed (discrete) energies and form a discrete emission spectrum Bremsstrahlung x-rays have a range of energies and form a continuous emission spectrum Maximum x-ray energy is associated with the minimum x-ray wavelength (λmin) A change in mA or mAs results in a proportional change in the amplitude of the x-ray emission spectrum at all energies A change in voltage peak affects both the amplitude and the position of the x-ray emission spectrum A change in kVp has no effect on the position of the discrete x-ray emission spectrum In the diagnostic range, a 15% increase in kVp is equivalent to doubling the mAs The overall result of added filtration is an increase in the average energy of the x—ray beam (higher quality, spectrum shift to the right) with an accompanying reduction in x- ray quantity (reduced spectrum amplitude) Increasing target atomic number increases the efficiency of x-ray production and the energy of characteristic and bremsstrahlung x-rays Because of reduced ripple, operation with 3-phase power or high frequency is equivalent to an approximately 12% increase in kVp or almost a doubling of mAs over single phase power Chapter 11 – x-ray emission, sectional (compensating) filtration X-ray quantity is the number of x-rays in the useful beam X-ray quantity and amperage I1/I2 = mAs1/mAs2 Where I1 and I2 are the x-ray intensities at the mAs1 and mAs2, respectively X-ray quantity is proportional to mAs Remember that mAs is just a measure of the total number of electrons that travel from cathode to anode to produce x-rays mAs = mA x s = mC/s x s = mC Where C (coulomb) is a measure of electrostatic charges and 1 C = 6.25x1018 electrons X-ray quantity and kVp 2 2 I1/I2 = (kVp1) /(kVp2) Where I1 and I2 are the x-ray intensities at kVp1 and kVp2, respectively X-ray quantity is proportional to the kVp2 X-ray Quantity and Distance 2 I1/I2 = (d2/d1) Where I1 and I2 are the x-ray intensities at distances d1 and d2 respectively X-ray quantity is inversely proportional to the square of the distance from the source When SID (source-to-image receptor distance) is increased, mAs must be increased by SID2 to maintain to maintain constant OD (average optical density The Square Law 2 2 mAs1/mAs2 = SID1 / SID2 Where mAs1 = the technique at SID1 and mAs2 = the technique at SID2 Adding filtration to useful x-ray beam reduces patient dose Penetrability is one description of the ability of an x-ray beam to pass through tissue Attenuation is the reduction in x-ray intensity resulting from absorption and scattering The HVL of an x-ray beam is the thickness of absorbing material necessary to reduce the x-ray intensity to half of its original value HVL is the best method for specifying x-ray quality Steps to Determine the HVL (with figure 11-3) 1. Determine the x-ray beam intensity with no absorbing material in the beam and then with different known thicknesses of absorber 2. Plot the ordered pairs of data 3. Determine the x-ray quantity equal to half the original quantity and locate this value on the y-axis of the graph (A). 4. Draw a horizontal line parallel with the x-ray from the point A in step 3 until it intersects the curve (B). 5. From point B, drop a vertical line to the x-axis 6. On the x-axis, read the thickness of absorber required to reduce the x-ray intensity to half of its original value point (C). This is the HVL. Increasing the kVp peak increases the quality of an x-ray beam Increasing filtration increases the quality of an x-ray beam Added filtration results in increased HVL Chapter 12 – x-ray interactions with matter, differential absorption Coherent scattering is of little importance to diagnostic radiology Compton Effect Ei = Es + (Eb + EKE) Where Ei = energy of the incident x-ray, Es = energy of the scattered x-ray, Eb = electron binding energy, and EKE = kinetic energy of the electron The probability of the Compton effect is inversely proportional to the energy (1/E) and independent of atomic number Compton scattering reduces contrast in an x-ray image Photoelectric Effect Ei = Eb + EKE Where Ei = the energy of the incident x-ray, Eb = electron-binding energy, and EKE = kinetic energy of the electron The photoelectric effect is total x-ray absorption interaction The probability of the photoelectric effect is inversely proportional to the third power of the x-ray energy (1/E)3 The probability of photoelectric effect is directly proportional to the third power of the atomic number of the absorbing material (Z3) Pair production does not occur during x-ray imaging Photodisintegration does not occur in diagnostic radiology Differential absorption occurs because of Compton scattering, photoelectric effect, and x- rays transmitted through the patient Differential absorption increases as the kVp is reduced To image small differences in soft tissue, one must use low kVp to get maximum differential absorption The interaction between x-rays and tissue is proportional to the mass density of the tissue regardless of the type of interaction Attenuation is the product of absorption and scattering Chapter 13 – film construction, Kodak film Image-forming x-rays are those that exit the patient and interact with the image receptor The base of radiographic film is 150-300 micrometers thick, semi-rigid, lucent, and made of polyester. The latent image is the invisible change induced in the silver halide crystal An ion is an atom that has either too many or too few electrons and therefore is electrically charged The result is the same whether the interaction involves visible light from an intensifying screen or direct exposure by x-rays Large-grain emulsions are more sensitive than small-grain emulsions Crossover is the exposure of an emulsion by light from the opposite side of the radiographic intensifying screen Rare earth screens are made with rare earth elements, atomic numbers 57-71 Reciprocity Law Exposure = Intensity x Time = Constant Optical Density The fog level for unprocessed film is approximately 0.2 mR (2 micro Gya) Chapter 14 – radiographic processing Developing is the stage of processing during which the latent image is converted to a visible image Fixing the silver halide that was not exposed to radiation is the process of clearing it from the emulsion and hardening the emulsion to preserve the image Wetting – (M = 15 sec, A = n/a) Swells the emulsion to permit subsequent chemical penetration Developing – (M = 5 min, A = 22 sec) Produces a visible image from the latent image Stop Bath – (M = 30 sec, A = n/a) Terminates development and removes excess chemical from emulsion Fixing – (M = 15 min, A = 22 sec) Removes remaining silver halide from emulsion and hardens gelatin Washing – (M = 20 min, A = 20 sec) Removes excess chemicals Drying – (M = 30 min, A = 26 sec) Removes water and prepares radiograph for viewing Synergism occurs when the action of two agents working together is greater than the sum of the action of each agent working independently Lack of sufficient glutaraldehyde may be the biggest cause of problems with automatic processing Archival quality refers to the permanence of the radiograph; the image thus does not deteriorate with age but remains in its original state Silver sulfide stain is the most common cause of poor archival quality The shorter dimension of the film should always be against the side rail in order to maintain the proper replenishment rate Film transport time should not vary by more than plus or minus 2% of the time specified by the manufacturer Cleaning the tanks and the transport system should be a part of the routine maintenance of any processor Most processing faults leading to damp film are due to a depletion of glutaraldehyde, the hardener in the developer A finished radiograph that is damp easily picks up dust particles that could result in artifacts Chapter 15 – film screens, film, screens, spinning top test The radiographic intensifying screen amplifies the image-forming x-rays that reach the screen-film cassette The phosphor converts the x-ray beam into light Isotropic emission means radiation with equal intensity in all directions Luminescence Fluorescence – no lag, < 10-8 s Phosphorescence – afterglow, >10-8 s Intensification Factor IF = Exposure required without screen Exposure required with screen Detective Quantum Efficiency DQE = number of x-rays absorbed x 100 number of incident x-rays Conversion Efficiency CE = emitted light x 100 x-rays absorbed Higher conversion efficiency results in increased noise Generally, those conditions that increase the IF reduce special resolution In mammography, the screen is positioned in contact with the emulsion on the side of the film away from the x-ray source to reduce screen blur and improve spatial resolution Screen-film compatibility is essential: use only those films for which the screens are designed Rare earth radiographic intensifying screens have the principal advantage of speed The combination of improved CE and higher DQE results in the increased speed of rare earth radiographic intensifying screens Chapter 16 – beam restricting devices Collimation reduces patient dose and improves contrast resolution Approximately 1% of incident x-rays reach the image receptor Scatter radiation increases as the field size of the x-ray beam increases Compression of anatomy improves spatial and contrast resolution and lowers patient dose Collimation reduces patient dose and improves contrast resolution Under no circumstances should the x-ray beam exceed the size of the image receptor Total Filtration Total Filtration = Inherent Filtration + Added Filtration Chapter 17 – grids, construction, performance Reduced image contrast results from scattered x-rays Grid Surface X-ray Absorption % x-ray absorption = width of grip strip x 100 width of grid strip + width of interspace Grid Ratio Grid Ratio = h/D High-ratio grids increase patient radiation dose The use of high-frequency grids requires high radiographic technique and results in higher patient radiation dose Grid Frequency Grid frequency = 10,000 micrometers/cm (T + D) micrometers/line pair The principal function of a grid is to improve image contrast Contrast Improvement Factor K = Radiographic Contrast with grid Radiographic contrast without grid The contrast improvement factor is higher for high-ratio grids Bucky Factor B = incident remnant radiation = patient dose with grid Transmitted image – forming radiation patient dose without grid As the bucky factor increases, radiographic technique and patient dose increase proportionately Selectivity Sigma = primary radiation transmitted through grid Scatter radiation transmitted through grid Grid Characteristics 1. High-ratio grids have high contrast improvement factors 2. High-frequency grids have low contrast improvement factors 3. Heavy grids have high selectivity and high contrast improvement factors Grid Cutoff Distance to cutoff = SID . Grid Ratio The main disadvantage of parallel and crossed grid is grid cutoff High-ratio grids have less positioning latitude than low-ratio grids In general, grid ratios up to 8:1 are satisfactory at tube potentials below 90 kVp. Grid ratios above 8:1 are used with kVp exceeds 90 kVp. Grid Selection Factors 1. Patient dose increases with increasing grid ratio 2. High-ratio grids are usually used for high-kVp examinations 3. Patient dose at high kVp is less than that at low kVp One disadvantage of the air-gap technique is image magnification with associated focal spot blur Chapter 18 – radiographic exposure and technique KVp controls radiographic contrast Ampere 1A = 1 C/s = 6.3 x 1018 electrons per second With a constant exposure time, mA controls x-ray quantity and therefore patient dose X-ray quality remains fixed with a change in mA Short exposure time reduces motion blur mAs Milliamperes (mA) x exposure time (s) mAs controls OD Equivalent Exposures of equal mAs mAs = mA x time mA (1st exposure) x time (2nd exposure) = mA (1st exposure) x time (2nd exposure) mA (1 st exposure) = time (2 nd exposure) mA (2nd exposure) time (first exposure) Total Projectile Electrons mA = mC/s, therefore mAs = mC/s x s = mC mAs is one measure of electrostatic charge Only the x-ray quantity is affected by changes in mAs Distance has no effect on radiation quality The Direct Square Law mAs versus SID mAs (2 nd exposure) = (SID) 2 (2 nd exposure) mAs (1st exposure) = (SID)2 (1st exposure) Distance (SID) affects OD Changing the focal spot for a given kVp/mAs setting does not change x-ray quantity or quality Half-wave rectification results in the same radiation quality as that for full-wave rectification, but the radiation quantity is halved The radiation quality does not change when going from half-wave to full-wave rectification; however, the radiation quantity doubles Three-phase power results in higher x-ray quantity and quality High-frequency generation results in even higher x-ray quantity and quality Chapter 19 – radiographic quality Spatial resolution improves as screen blur decreases, motion blur decreases, and geometric blur decreases Radiographic noise is the random fluctuations in the OD of the image The use of high mAs, low kVp settings, and slower image receptors reduces quantum mottle Radiographic Quality Rules 1. Fast image receptors have high noise and low spatial resolution and contrast resolution 2. High spatial resolution and contrast resolution require low noise and slow image receptors 3. Low noise accompanies slow image receptors with high spatial resolution and contrast resolution An increase in LRE of 0.3 results from doubling the radiation exposure Optical Density OD = log10 Io It Higher fog density reduces the contrast of the radiographic image Base plus fog OD has a range of approximately 0.1 to 0.3 The reciprocity law states that the OD on a radiograph is proportional only to the total energy imparted to the radiographic film Film contrast is related to the slope of the straight-line portion of the characteristic curve Image Receptor Contrast Average Gradient = OD2 – OD1 LRE2 – LRE1 Where OD2 is the optical density of 2.0 plus base and fog densities, OD1 is the optical density of 0.25 plus base and fog densities, and LRE2 and LRE1 are the LREs associated with OD2 and OD1 respectively Image Receptor Speed Speed = 1 . Exposure in roentgens to produce an OD of 1.0 plus base + fog Speed vs mAs New image receptor speed = old mAs Old image receptor speed new mAs Latitude and contrast are inversely proportional Factors affecting the finished radiograph The concentration of processing chemicals The degree of chemistry agitation during development The development time The development temperature Geometric Factors Magnification Distortion Focal-spot blur Magnification Factor MF = Image Size/object size = SID/ SOD Object Size = Image Size x (SOD/SID) Minimizing Magnification Large SID Small OID Unequal magnification of different portions of the same object is called shape distortion Distortion depends on Object thickness, position, and shape Thick objects are more distorted than thin objects If the object plane and image plane are not parallel, distortion occurs Focal spot blur occurs because the focal spot is not a point Focal spot blur is the most important factor in determining spatial resolution Focal Spot Blur SOD/OID = Effective focal spot/Focal spot blur The focal spot blur is small on the anode side and large on the cathode side Radiographic Contrast RC = film contrast x subject contrast kVp is the most important influence on subject contrast Patient motion is usually the cause of motion blur Principles to be considered when planning a particular examination 1. Use of intensifying screens decreases patient dose by a factor of at least 20 2. As the speed of the image receptor increases, radiographic noise increases and spatial resolution is decreased 3. Low-contrast imaging procedures have a wider latitude, margin of error, in producing an acceptable radiograph Keep exposure time as short as possible The primary control of radiographic contrast is kVp The primary control of OD is mAs</p>
Details
-
File Typepdf
-
Upload Time-
-
Content LanguagesEnglish
-
Upload UserAnonymous/Not logged-in
-
File Pages15 Page
-
File Size-