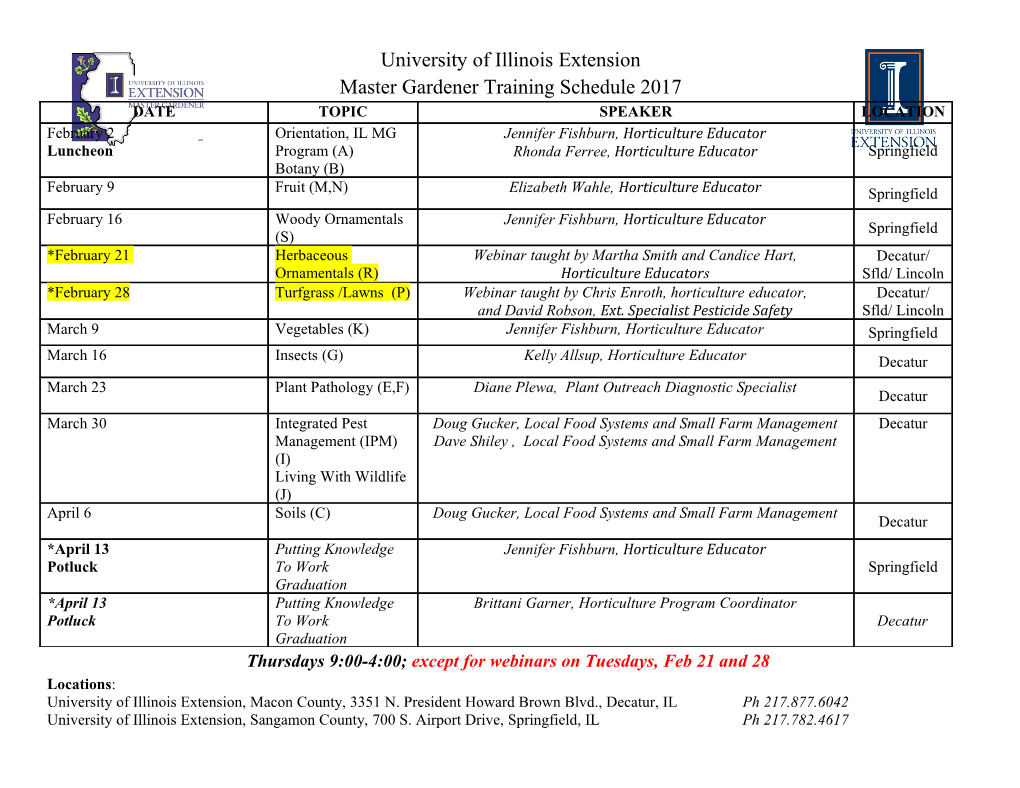
FLARM: Collision Threat Identification Influenced by Pilot’s Mental Model of the Cockpit Display Christoph G. Santel Uwe Klingauf [email protected] [email protected] Institute of Flight Systems and Automatic Control Technische Universitat¨ Darmstadt Darmstadt, Germany Abstract During the last decade, low-cost collision alerting systems have become available to assist glider pilots in their task of seeing and avoiding other traffic. These systems present pilots with traffic information on different displays. Previous work by the authors shows that many glider pilots make systematic errors in interpreting traffic information shown on one such display, the popular FLARM display. This is the case whenever the pitch angle, bank angle or drift angle deviate from zero. In this paper data from the aforementioned research are analyzed using analytical modeling of the pilots’ mental models in order to explain these systematic errors. Results show that the majority of glider pilots rely on a glider-fixed reference system when interpreting the traffic indications on the FLARM display. This is attributed to the available in-cockpit clues on the display. Since the data displayed on the FLARM display are actually derived from a FLARM-specific coordinate system — and is not glider-fixed — it is only natural that many pilots make systematic errors in predicting the position of traffic shown on the display. At the end of the paper possible courses of action to reduce these interpretation errors are discussed. Introduction Gliding is an air sports activity that is mostly carried out un- ρ der visual flight rules. Consequently, in most airspace classes glider pilots are responsible for visually detecting and avoiding other traffic [1, section 2.6]. In order to support glider pilots in their task of seeing and avoiding other traffic, low-cost collision alerting systems (CASs) have become available [2]. ε The FLARM collision alerting system By far the most popular CAS in gliding is the FLARM sys- tem. It represents a quasi-standard in the German gliding com- munity [3]. The system relies on participating aircraft being equipped with a cooperative FLARM transceiver unit. This transceiver unit detects the glider’s own position using a Global Fig. 1: FLARM display Positioning System (GPS) module and broadcasts this position to other aircraft using a proprietary radio protocol [4]. At the same time, the transceiver receives position reports from other displays the relative bearing ρ between the glider’s ground track aircraft within range. These position reports are then assessed and the traffic’s direction, as projected into the horizontal plane. for collision threats [5]. Directional information to the nearest The elevation ε of the traffic represents its angular distance or most threatening traffic known is displayed on a simple dis- above or below the horizon, as seen from the pilot’s point of play unit, as shown in Fig. 1. view in her or his own glider. This FLARM coordinate system This display shows the directional information in terms of po- can be also described with an analogy from the field of geogra- lar coordinates. A circular array of light-emitting diodes (LEDs) phy. The glider is located at the center of a globe in this analogy. VOL. 38, NO. 4 October–December 2014 43 TECHNICAL SOARING The globe’s equatorial plane (EP) is parallel to the horizontal ates. However, the previous work does not disclose how each plane and its zero-meridian passes through the glider’s ground individual pilot believes the FLARM CAS to work. It is only track. The traffic’s bearing ρ is represented by longitude and its logical to ask the question of how each glider pilot believes the elevation ε by latitude. Figure 2a illustrates this analogy. system to operate. However, this coordinate system is not self-evident from the graphical clues provided by the display. The FLARM display Mental models shows the top view of an airplane at the center of the circular The problem of how a pilot believes a certain aircraft system LED array. Using the concept of pictorial realism [6], a user to operate is a question of mental models (MMs). When inter- might assume that her or his own glider’s wingplane represents acting with any system, humans form a model of how this sys- the polar coordinate system’s EP. Similarly, the sketched air- tem works and what its purpose is. These MMs can take many plane points to the top of the LED circle, suggesting that the shapes. One extreme of a MM is a mere “black box,” where glider’s longitudinal axis passes through the zero-meridian. The simple rules of thumb are applied to relate input and output and polar coordinate system, which is suggested by the display’s in- where the user has no deeper understanding of a system’s inter- cockpit visual clues, is a glider-fixed polar coordinate system nal characteristics. The opposite extreme may be a user who has (Fig. 2b). When comparing Figs. 2a and 2b it is evident that a detailed and intricate understanding of the internal technical these coordinate systems do not necessarily coincide. workings of a system. The question of “why does FLARM rely on this non-intuitive According to Rouse and Morris “mental models are the mech- FLARM coordinate system?” might arise. A major advantage anisms whereby humans are able to generate descriptions of of this coordinate system is that all indications can be calcu- system purpose and form, explanations of system functioning lated from GPS measurements alone. No data about the glider’s and observed system states, and predictions of future system own attitude or the traffic’s attitude are required for these indica- states” [9, p. 7]. Consequently, pilots form MMs of systems tions. Therefore, the FLARM transceiver units do not need to be with which they interact, such as CASs. Wickens, Lee, Liu and equipped with attitude sensors and can be marketed at a lower Gordon Becker mention that often-times display design shapes price. Low costs, along with the benefit of receiving collision the MMs of a system developed by its users [10, chap. 8]. alerts, are considered to be driving factors in the rapid distribu- Empirical identification of MMs is a task prone with difficul- tion of low-cost CASs in parts of Europe [7, p. 103]. ties. Uncovering all details of how any given person models a This paper’s authors and their colleagues have found that technical system would be a task nearly impossible. Yet, several glider pilots make systematic errors in estimating traffic posi- methods are available to identify MMs. tions whenever the FLARM coordinate system and glider-fixed Direct inquiry requires interviewing the participant on how coordinate system do not coincide [8]. The data from their ex- she or he believes a system to operate. It can only periment suggest that at least some glider pilots use a glider- provide results which the participant is able to verbalize fixed coordinate system. So far this has been attributed to the [9, pp. 13–16]. concept of pictorial realism [6], which results in glider pilots gaining a false understanding of how the FLARM CAS oper- Empirical modeling indirectly infers characteristics of a MM (a) FLARM polar coordinate system (b) glider-fixed polar coordinate system Fig. 2: Orientation of polar coordinate systems while maneuvering. TECHNICAL SOARING 44 VOL. 38, NO. 4 October–December 2014 based on experimental results. For example, when compar- ing the performance of multiple groups while performing the same experimental task, one might infer that a supe- rior MM is developed by the group with the best perfor- mance. However, this does not provide direct insight into the MM [9, pp. 10–11]. Analytical modeling requires making multiple “educated guesses” about how a MM might look. These multiple guesses are then compared to the experimental data and the best-fitting guess is selected as being the most- likely MM. However, it cannot be said with certainty that the best-fitting MM reflects the actual MM of the participant [9, pp. 10–11]. Empirical modeling of pilots interacting with the FLARM display is used by Mehringskotter¨ [11]. Since the results of this study were unsatisfactory, he recommends that future work use Fig. 3: Flight simulator cockpit with gridded projection screen. analytical modeling [section 6.5.1.1]. Therefore, an analytical modeling approach is chosen in the paper at hand. Control at Technische Universitat¨ Darmstadt [12]. As the glider Hypothesis pilot participants did not have to perform a flight task, the sim- MMs are expected to vary between glider pilots. However, the ulator merely served to provide static visual immersion into dif- authors’ previous work suggests that MMs based on the glider- ferent flight scenarios. The simulator’s out-the-window (OTW) fixed coordinate system are most frequent [8]. This leads to the view was provided using the Diamond Global Canvas Visual following hypothesis. System image generation software [13]. The projection screen was gridded into 50 cells (see Fig. 3) and each cell was marked Hypothesis: Mental models based on the glider- with an alphanumeric identifier. fixed polar coordinate system are most frequently At the cockpit’s center, above the audio panel, an external found in glider pilots using the FLARM CAS’s dis- FLARM display V3 [14] was installed. It sat atop the audio play. panel and beneath the standby instruments. The participants were handed a touch screen monitor [15] to Method perform their experimental task. Participants loosely placed the In the presented approach an explorative analysis is con- monitor on their laps. On this monitor (Fig. 4), an answer grid ducted. The analyzed data set was previously gathered and ana- analogous to the grid in the OTW view, was shown.
Details
-
File Typepdf
-
Upload Time-
-
Content LanguagesEnglish
-
Upload UserAnonymous/Not logged-in
-
File Pages10 Page
-
File Size-