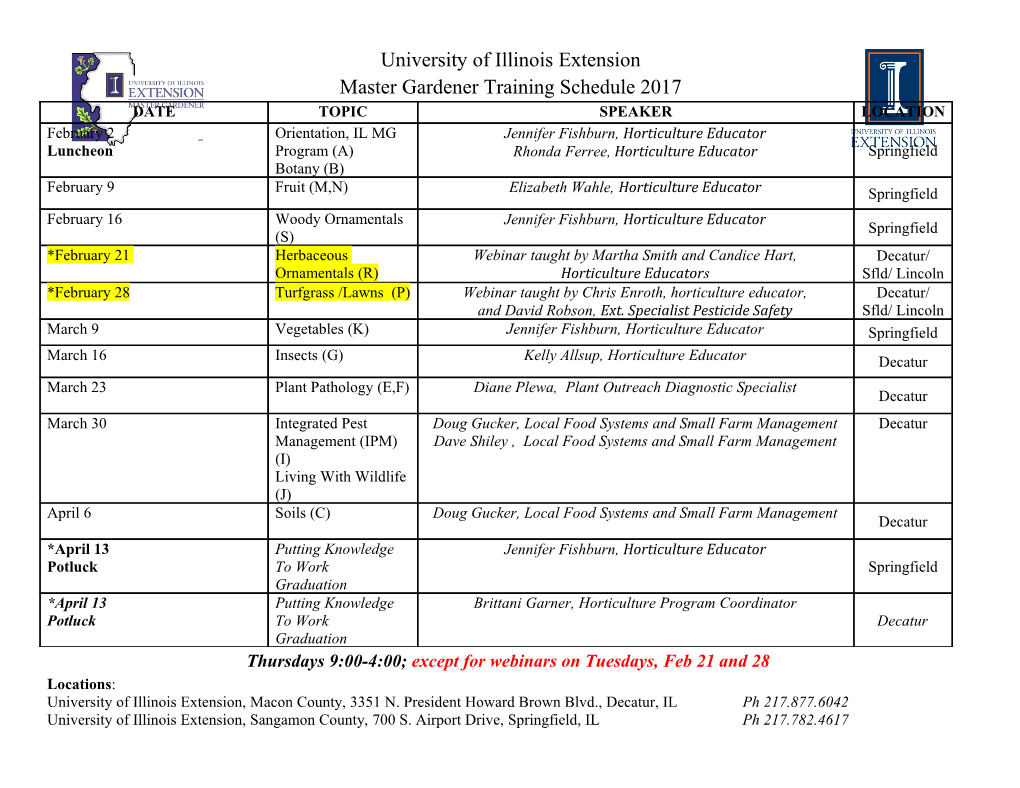
<p>DEPARTMENT for ENVIRONMENT, FOOD and RURAL AFFAIRS CSG 15 Research and Development Final Project Report (Not to be used for LINK projects)</p><p>Two hard copies of this form should be returned to: Research Policy and International Division, Final Reports Unit DEFRA, Area 301 Cromwell House, Dean Stanley Street, London, SW1P 3JH. An electronic version should be e-mailed to [email protected]</p><p>Project title Changes in Gene Expression Profiles under Nutrient Deficiency</p><p>DEFRA project code HH3502SFV</p><p>Contractor organisation Horticulture Research International, and location Wellesbourne, Warks CV35 9EF</p><p>Total DEFRA project costs £ 44,624</p><p>Project start date 10/01/01 Project end date 31/03/02</p><p>Executive summary (maximum 2 sides A4)</p><p> This project contributes to DEFRA's policy objectives: (a) to promote sustainable management and prudent use of natural resources, and (b) to protect the environment and conserve and enhance biodiversity.</p><p> It addresses the problem of excessive P fertilisation of crops, which is both costly and can lead to unnecessary pollution. Excessive P fertilisation occurs because chemical assays of both soil and plant P are unreliable. Novel biosensor technologies, based on knowledge of the changes in plant gene expression under P-starvation, could better inform P-fertiliser application.</p><p> Novel biosensor technologies might utilise transcripts from P-responsive genes to develop customised DNA-array and antibody-based bioassays of P-stress. Alternatively, the promoters of P-responsive genes could underpin the development of 'smart plants', in which P-responsive promoters control the expression of genes encoding visible products whose abundance reflects plant P status. </p><p> Our aim was to identify genes, expressed in shoot tissues of the model plant Arabidopsis thaliana, whose transcripts increased rapidly and specifically in response to P starvation. Expression profiling using Affymetrix GeneChip technology allowed us to assay the abundance of transcripts from approximately 8,000 genes (33% genome coverage) simultaneously.</p><p> Our initial objectives were to determine the effects of withdrawing the essential mineral nutrients P, N or K on shoot growth, mineral content and gene expression in Arabidopsis growing hydroponically. Withdrawing P reduced shoot P concentration after about 24 h, and slowed shoot growth after about 100 h. Thus, gene transcripts that increased in abundance between about 24 to 100 h following P withdrawal might be useful</p><p>CSG 15 (9/01) 1 Project Changes in Gene Expression Profiles under Nutrient DEFRA HH3502SFV title Deficiency project code</p><p> for biosensor development. Withdrawing P had no effect on shoot N or K concentration over this period. Similarly, withdrawing N or K did not affect shoot P concentration.</p><p> Our ultimate objective was to identify genes whose expression was increased specifically by P-starvation and before P-starvation affected plant growth. The expression of several hundred genes increased during P starvation. Of these, 37 genes were identified as being potentially useful for biosensor technologies. The expression of these 37 genes did not increase immediately (4 h) after P withdrawal, but increased at least threefold 28 to 100 h after P withdrawal. However, the expression of 19 of these genes was also increased by both N and K starvation, which suggests that they might be controlled by a common mineral-imbalance stress-response system. The expression of a further 5 genes was increased by both N and P starvation, and the expression of 8 genes was increased by both P and K starvation. The expression of the remaining four genes appeared to be increased specifically by P starvation. </p><p> The four (provisional) marker-genes specific for P starvation were identified as encoding LEA M17 (which is related to proteins that confer tolerance of stresses associated with a water-deficit), a nodulin-like protein (which is homologous to the MtN21 protein expressed during nodule organogenesis in Medicago truncatula), strictosidine synthase (which catalyses the condensation of tryptamine and secologanin to form strictosidine, a key intermediate in indole alkaloid production) and a protein with unknown function.</p><p> In conclusion: Transcripts and promoters from genes responding specifically to P-starvation can now be used to develop biosensor technologies to assay plant P status. These technologies will help reduce the application of P fertiliser. This will lower costs and reduce pollution, thereby delivering DEFRA's policy objectives for the sustainable use of natural resources, protection of the environment and enhanced biodiversity.</p><p>CSG 15 (9/01) 2 Project Changes in Gene Expression Profiles under Nutrient DEFRA HH3502SFV title Deficiency project code</p><p>Scientific report (maximum 20 sides A4) 1. INTRODUCTION</p><p>The UK horticultural and agricultural industries routinely apply large amounts of inorganic fertiliser-P to maintain crop yields and quality. It is estimated that up to £50/ha is spent annually on P-fertilisers for vegetables (equivalent to £7M nationally). More P is applied than is required because chemical assays of both soil and plant P are unreliable. Analyses of soil P are unreliable because critical concentrations of extractable soil P for maximum yield depend on soil type, and few farmers know the critical values for their fields. Analyses of plant P are difficult to interpret not only because little information is available for the critical tissue P concentration of vegetables, but also because these values depend on environmental, physiological and developmental parameters. Excessive P fertilisation is both costly and can lead to unnecessary pollution. Possible solutions to this problem include in situ assays of plant-P (Bollons & Barraclough, 1997, 1999), or the use of novel biosensor technologies based on knowledge of the changes in plant gene expression under P-starvation (White & Rahn, 1999), to inform fertiliser application. Several biosensor technologies can be envisaged. These include: (i) 'smart plants', in which P-responsive promoters control the expression of genes encoding visible products whose abundance reflects plant P status, (ii) custom-designed DNA microarrays, to detect the transcripts of genes whose expression is altered by P starvation, and (iii) traditional antibody-based assays that detect the presence of proteins accumulating when plants lack P. The aim of this project was to identify genes expressed in shoot tissues that are upregulated rapidly and specifically in response to P starvation. It utilises the model plant, Arabidopsis thaliana, since gene expression profiling can be performed readily in Arabidopsis using Affymetrix GeneChip technology, which allows the experimenter to assay the expression of approximately 8,000 genes (33% genome coverage) simultaneously. It is envisaged that transcripts of these genes will underpin the development of customised DNA-array and antibody-based bioassays of P-stress, and that promoters of these genes will underpin the development of 'smart plants'. In addition, knowledge of the changes in gene expression that occur when P supply is compromised will improve our general understanding of the physiology of P nutrition. Our approach was to combine knowledge of how the withdrawal of individual mineral nutrients (N, P, K) affects shoot mineral content, growth and gene expression to realise two overall scientific objectives: (A) to identify genes whose expression increases as the P concentration of the shoot declines, but before a shoot growth is compromised, and (B) to determine the subset of these genes that are upregulated specifically by P deficiency.</p><p>2. EXPERIMENTAL METHODS</p><p>Plant Material</p><p>Seeds of Arabidopsis thaliana (L.) Heynh. (Columbia Col–5, from Nottingham Arabidopsis Stock Centre, NASC, #N1688) were washed in 70% (v/v) ethanol/water and surface sterilised using NaOCl (1% active chlorine). Sterilised seeds were imbibed for 3-5 d in sterile distilled water at 4°C to break dormancy. Following imbibation, seeds were sown in unvented, polycarbonate culture boxes (Sigma-Aldrich Company Ltd., Dorset UK). Seedlings were grown for 21 d on perforated polycarbonate discs (diameter 91 mm) placed over 75 ml 0.8% (w/v) agar containing 1% (w/v) sucrose and a basal salt mix (Murashige & Skoog, 1962). Boxes were placed in a growth room set to 24°C, with 16 h light per day. Illumination was provided by a bank of 100W 84 fluorescent tubes (Philips, Eindhoven, Netherlands) giving an intensity of 45 mol photons m-2 s-1 at plant height. Roots grew into the agar, but shoots remained on the opposite side of the disc. After 21 d, seedlings were transferred, still on polycarbonate discs, to a hydroponics system in a Saxcil growth cabinet. Plants were illuminated for 16 h daily at a light intensity of approximately 75 µmol photons m-2 s-1 at plant height. Temperature was maintained at 24°C during the light period and 16°C during the dark. The</p><p>CSG 15 (9/01) 3 Project Changes in Gene Expression Profiles under Nutrient DEFRA HH3502SFV title Deficiency project code</p><p> relative humidity was approximately 80%. Each polycarbonate disc was placed in a light-proof 500 ml beaker over 450 ml aerated complete nutrient solution containing 0.75 mM K+, 4.025 mM Ca2+, 0.75 mM Mg2+, 0.01 mM 2+ 2+ 2+ + 2- - 2- Mn , 0.001 mM Zn , 0.003 mM Cu , 0.001 mM Na , 0.25 mM H2PO4 , 8.0 mM NO3 , 0.764 mM SO4 , 0.05 - - 2- mM Cl , 0.03 mM H2BO3 , 0.0005 mM MoO4 , 0.1 mM FeNaEDTA. Nutrient solution was recirculated using a peristaltic pump at a flow rate of 30 ml min-1 through four beakers and a central reservoir containing 6 l aerated nutrient solution. Four such hydroponic units could be operated simultaneously. Nutrient solutions were replaced twice a week. Plants were grown hydroponically for 7 d in complete nutrient solution prior to experimentation. Experiments began 28 d after sowing. To determine the effects of P, N or K starvation on shoot growth, mineral content and gene expression, the complete nutrient solution was replaced four hours into the light period with nutrient solutions lacking these elements. Sulphate replaced either phosphate or nitrate in solutions lacking P or N, respectively. Calcium replaced potassium in solutions lacking K.</p><p>Analysis of Plant Growth and Mineral Content</p><p>Plants were harvested at various intervals following P, N or K withdrawal. At each harvest the fresh weight of the shoot was determined for individual plants. Shoot material was bulked, dried at 80°C for 48 hours and the dry weight determined. Tissue P and K contents were determined following digestion of bulked and weighed material from batches of 25 plants by inductively coupled plasma optical emission spectrophotometry (JYHoriba Ultima 2 ICP-OES, Jobin Yvon Ltd, Middlesex, UK). Tissue N content was determined on a subsample of dried material loaded directly into a Leco CN 2000 combustion analyser (Leco UK Ltd, Cheshire, UK).</p><p>Gene Expression Studies </p><p>To avoid any complications resulting from light- or circadian-regulation of gene expression (Desprez et al. 1998; Kehoe et al. 1999; Harmer et al. 2000; Ma et al. 2001; Schaffer et al. 2001), all plants were harvested at the same point in the light cycle. Gene expression was determined on shoot material harvested -20, 4, 28 and 100 h after transfer to experimental conditions. To control for biological variation, shoot material from four to eight plants was bulked and snap frozen in liquid nitrogen. Thus, the gene expression recorded for each sample was the common (average) response of biological replicates. Samples were stored at –70°C prior to total RNA extraction. Total RNA was extracted from tissue samples following the addition of 1 ml TRIzol reagent, according to the manufactures instructions (Invitrogen Life Technologies, Rockville, Maryland). To test biological reproducibility, replicate experiments were performed (Table 1). To provide quality control of total RNA samples, OD260/OD280 was determined and RNA gel pictures were scrutinised. Total RNA was sent to AROS Applied Biotechnology (Aarhus, Denmark) for labelling and GeneChip™ analysis (Affymetrix, Santa Clara, Ca, USA). Following the precedent of Chen et al. (2002), any average difference (expression level) below 5 was floored to 5. The fold-change for each gene was calculated by dividing the average difference of an experimental sample by the average difference of an appropriate control sample. If, for any complete set of comparisons, the Affymetrix software declared the gene transcripts "absent", these data were eliminated from analyses. The database of Ghassemian et al. (2001) was used to map GeneChip™ ID to Arabidopsis Genome Initiative (AGI) identifiers.</p><p>Note: The work relating to genechip analysis carried out as part of this Project was subject to the agreement of certain licensing conditions with Affymetrix. The recipient(s) of this report therefore need(s) to be aware that any commercial exploitation of the results of this Project may require the further negotiation of licence terms with Affymetrix. </p><p>CSG 15 (9/01) 4 3. THE EFFECT OF NUTRIENT STARVATION ON SHOOT GROWTH AND MINERAL CONTENT</p><p>Twenty-eight days after sowing, P, N or K were withdrawn individually from Arabidopsis plants growing hydroponically and the timecourses of development of nutrient deficiencies were determined (Fig. 1). Shoot mass was unaffected for at least 100 hours after nutrient withdrawal, but P starvation significantly reduced shoot mass subsequently (Fig. 1A). For use as a diagnostic of P status, changes in gene expression upon P starvation must be observed within the initial 100 h following P withdrawal. No change in shoot P concentration was observed over the initial 24 h following P withdrawal, but shoot P concentration was reduced significantly between 24 and 72 h after P withdrawal (Fig. 1B). Thus, for use as a diagnostic of P status, it is likely that changes in gene expression upon P starvation need to be observed 24 to 72 h following P withdrawal, as the shoot P concentration declines. The withdrawal of neither N nor K affected P status over this period (Fig. 1B), and P starvation did not affect shoot N or K concentrations (data not shown). It would appear that a deficiency of one nutrient does not affect the tissue concentration of another. It is possible, therefore, to identify genes regulated specifically by P starvation.</p><p>35 12 )</p><p>30 W</p><p>D 10</p><p>) g / g</p><p>25 g</p><p> m 8 ( m</p><p>(</p><p> s t</p><p> s 20 n a</p><p> e 6 t m</p><p> n</p><p> t 15 o o c</p><p> o 4 h 10 P</p><p>S t o</p><p>5 o 2 h S 0 0 0 100 200 300 0 100 200 300 Time after nutrient withdrawal (h) Time after nutrient withdrawal (h)</p><p>Figure 1. The effect of P, N or K withdrawal from the nutrient solution bathing roots of Arabidopsis plants on shoot mass and shoot P concentration. Plants were grown in complete nutrient solution (●) and solutions lacking P (○), N (□) or K (Δ). Data are expressed as mean ± SEM.</p><p>4. THE EFFECT OF NUTRIENT STARVATION ON GENE EXPRESSION IN ARABIDOPSIS SHOOTS</p><p>Total RNA was extracted from shoots of Arabidopsis grown with a variety of nutritional treatments for various periods (Table 1). Timepoints were chosen at which (i) no nutrient deficiency was apparent (4 h), (ii) plants approached the critical shoot nutrient concentration for deficiency (28 h) and (iii) the effects of nutrient deficiency on shoot growth were imminent (100 h). About 5000 gene transcripts could be detected in each of these experiments.</p><p>Time of Harvest (h) Treatment -20 4 28 100</p><p>Full nutrient 1, 21 9 3, 13, 23 7, 17, 27 Minus N 1, 21 11 5, 15, 25 19 Minus P 1, 21 2, 10, 22 6, 14, 24 8, 18, 28 Minus K 1, 21 12 4, 16, 26 20</p><p>Table 1. HRI numbering of 28 Affymetrix GeneChips™ used for gene expression profiling. These are divided into individual experiments (colours) and include the experiments originally designated 'Genomic Arabidopsis Resource Network (GARNet) Experiment 1' (red), 'GARNet experiment 2' (blue) and 'Objective Experiment' (black).</p><p>This array of treatments yields several biological comparisons. First, it enables the changes in gene expression during ontogeny in plants grown in a complete nutrient solution to be determined. These are appreciable (Fig. 2; Ruan et al. 1998; Zhu et al. 2001). Thus, comparison of the changes in gene expression following nutrient withdrawal can only be determined relative to a control plant grown in complete nutrient solution harvested at a Project Changes in Gene Expression Profiles under Nutrient MAFF title Deficiency project code HH3502S FV comparable time. Second, by comparison with appropriate control plants, the temporal changes in the expression of genes following nutrient withdrawal can be determined (Fig. 3). By comparing the changes in expression profiles following P withdrawal, genes that are upregulated rapidly, but not transiently, by P depletion (ie genes upregulated at both 28 and 100 h) can be identified (Overall Scientific Objective A; Genomic Arabidopsis Resource Network, GARNet, Experiment 2). By comparing the gene expression profiles in shoots of plants in which either N, P or K are withdrawn with appropriate control plants, subsets of genes that are upregulated by lack of specific nutrients and those that are upregulated by a common mineral- imbalance stress-response system can be identified (Overall Scientific Objective B; GARNet Experiment 1).</p><p>100000 100000 h h </p><p>0</p><p>8 10000 10000 0 2 1</p><p> t t a a</p><p> n 1000 n 1000 o o i i s s s s e e r 100 r 100 p p x x e e</p><p> e e n 10 n 10 e e G G 1 1 1 10 100 1000 10000 1 10 100 1000 10000 Gene expression at -20 h Gene expression at -20 h</p><p>Figure 2. Changes in gene expression during the development of Arabidopsis. Arabidopsis were grown in a complete nutrient solution. GeneChip™ analysis was performed on RNA from shoots of plants harvested at three timepoints (-20, 28 and 100 h). Gene expression at 28 and 100 h was compared with gene expression at -20 h for all 5257 transcripts declared present by the Affymetrix software. Lines indicate a two-fold difference in gene expression between timepoints.</p><p>100000 100000 100000</p><p>10000 10000 10000 P K N - - -</p><p> n n n o o o i i i 1000 1000 1000 s s s s s s e e e r r r p p p x x x 100 100 100 e e e</p><p> e e e n n n e e e</p><p>G G 10</p><p>G 10 10</p><p>1 1 1 1 10 100 1000 10000 1 10 100 1000 10000 1 10 100 1000 10000 Gene express ion FNS Gene expression FNS Gene expression FNS</p><p>Figure 3. Changes in gene expression in shoots of Arabidopsis following the withdrawal of N, P or K from the nutrient solution. Gene expression in plants from which N, P or K had been withdrawn for 28 hours was compared with gene expression in plants grown in complete nutrient solution (FNS) for all 5500 transcripts declared present by the Affymetrix software. Lines indicate a two-fold difference in gene expression.</p><p>Validation of the Microarray Data: Nitrate-regulated Gene Expression</p><p>A comparison of the genes regulated by nitrate identified in our microarray experiments with those reported in the literature provided a simple validation of our data. We observed the downregulation of many genes when nitrate was withdrawn (Fig. 3), which is consistent with the upregulation of many genes when nitrate is supplied (Wang et al. 2000). Further, several genes (although not all genes) classically found to be upregulated in the presence of nitrate were duly downregulated upon nitrate starvation. Thus, the expression of ACH1, a gene encoding a high-affinity nitrate transporter, was downregulated fourfold by N starvation for 28 h, but the expression of genes for the nitrate transporters CHL1 and NTL1/NRT1 was unaffected. Similarly, among the</p><p>6 Project Changes in Gene Expression Profiles under Nutrient MAFF title Deficiency project code HH3502S FV</p><p>'novel' genes found to be upregulated in Arabidopsis in the presence of nitrate by Wang et al. (2000), we observed that the expression of the gene encoding a non-symbiotic hemoglobin (AHB1) was halved by withdrawing N for 28 h, but that the expression of neither the high affinity vacuolar calcium antiporter (CAX1) nor the late embryogenesis abundant (LEA) protein homolog SAG21 was affected after 28 h N starvation. Wang et al. (2000) also reported that several genes were repressed by nitrate in Arabidopsis. In our experiments, removing nitrate had no effect on the expression of genes encoding AMT1 (an ammonium transporter), ANR1 (a MADS box protein supposedly expressed specifically in roots), or an homeobox-leucine zipper protein (Athb-12). However, the gene encoding phosphoglycerate dehydrogenase (AT4g20380; BAA24440.1) was upregulated fivefold when nitrate was removed, which is consistent with the observations of Wang et al. (2000). Our inability to detect changes in the expression of some genes following N withdrawal may reflect the mildness of N-deficiency. In our experiments, a critical shoot N concentration had not been reached 28 h after withdrawing N.</p><p>Genes Upregulated during P Starvation</p><p>Time after P Downregulated Upregulated Upregulated withdrawal (h) > 2 > 2 > 3</p><p>4 261 435 152 28 413 466 139 100 452 649 266</p><p>Table 2. The number of genes whose expression in Arabidopsis shoots was downregulated by at least twofold, or upregulated by at least twofold or threefold at various times following the removal of P from the nutrient solution. In these experiments 5770 transcripts were declared present by the Affymetrix software.</p><p>The conventional criterion for differential gene expression is a twofold difference, at which the Affymetrix GeneChip™ has a rate of approximately 0.2% false-positives (Zhu & Wang, 2000). We observed that the expression of many genes was significantly affected by removing P from the nutrient solution (Fig. 3B; Table 2). The expression of several hundred genes was upregulated by P starvation. Genes whose expression is greatly increased upon P starvation will provide stronger molecular markers for physiological P deficiencies. Thus, only the genes whose expression more than tripled following P starvation were selected for subsequent analyses. The expression of 37 genes, which were not upregulated immediately (4 h) after P withdrawal, was increased both 28 and 100 h after P withdrawal (Fig. 4).</p><p>4 h 87</p><p>44 6 15</p><p>170 37 81 100 h 28 h</p><p>Figure 4. The number of genes whose expression was increased at least threefold upon the withdrawal of P from nutrient solutions for 4, 28 or 100 h. The identities of the 37 genes that were upregulated after 28 and 100 h, but not after 4 h, are given in Table 4.</p><p>7 Project Changes in Gene Expression Profiles under Nutrient MAFF title Deficiency project code HH3502S FV</p><p>Genes Upregulated Specifically by P Starvation</p><p>Nutrient Upregulated Upregulated Withdrawn > 2 > 3</p><p>P 463 135 N 1191 467 K 722 260</p><p>Table 3. The number of genes whose expression in Arabidopsis shoots was increased at least twofold or at least threefold following the removal of P, N or K from the nutrient solution for 28 h.</p><p>Many more genes were upregulated following the withdrawal of N or K from the nutrient medium than following the withdrawal of P (Fig. 3; Table 3). Nineteen of the 37 candidate marker genes whose expression was increased at least threefold as shoot P concentration declined, but before shoot growth was slowed, were also upregulated at least twofold by both N and K starvation. These genes may be upregulated by a common mineral-imbalance stress-response system. The expression of 5 candidate marker genes was upregulated by both N and P starvation, and the expression of 8 more genes was upregulated by both P and K starvation. Only four candidate marker genes were specifically upregulated by P starvation. These genes encoded LEA AtM17 (which is a member of the Late Embryogenesis Abundant proteins that are implicated in tolerance of several stresses associated with a water-deficit, Raynal et al. 1999, Bray et al. 2000), a nodulin-like protein (which is homologous to the MtN21 protein expressed during nodule organogenesis in Medicago truncatula, Gamas et al. 1996), strictosidine synthase (which catalyses the condensation of tryptamine and secologanin to form strictosidine, a key intermediate in indole alkaloid production) and a protein with unknown function. The expression of LEA AtM17 also appears to be induced by low temperature stress in young Arabidopsis seedlings (Raynal et al. 1999). However, this is unsurprising, since interrelationships between plant P status and the expression of genes impacting on low temperature acclimation have been reported previously (Hurry et al. 2000). It has also been suggested that the ABI3 transcription factor participates in regulating LEA AtM17 expression (Raynal et al. 1999). Because the expression of the four genes we have identified appears to be regulated nutritionally solely by P status, their transcripts could immediately be used to develop custom- designed DNA-arrays to assay plant P status, or, following heterologous expression, could be used to produce protein to develop antibody-based assays for plant P status. Promoters from these genes could be used in 'smart plants' to control the expression of genes encoding visible products (such as the Green Fluorescent Protein) whose abundance would then reflect plant P status. Indeed, it would be interesting to discover whether the promoters of these genes share common regulatory elements.</p><p>8 Project Changes in Gene Expression Profiles under Nutrient MAFF title Deficiency project code HH3502S FV</p><p>P starved P starved P starved N starved K starved For 4 h For 28 h For 100 h For 28 h For 28 h GeneChip™ Expression Increased Arabidopsis Genome Initiative (AGI) ID ID Level Expression relative to full nutrient solution Expression</p><p>16896_s_at M 1.00 4.98 5.66 1.00 1.00 P LEA M17 protein At2g41270 AAC78545.1 13967_at M 1.05 3.57 3.47 1.27 1.97 P Medicago nodulin MtN21-like AT4g28040 CAB36773.1 protein 15622_s_at H 2.84 3.23 5.73 1.87 1.35 P Strictosidine synthase (STS1) At1g74020 AAB40594.1 20558_at M 2.04 3.17 3.10 ND ND P Putative protein AT4g36850 CAB16817.1</p><p>12571_s_at M 1.18 7.91 4.11 15.66 0.21 NP Ferrochelatase-I (SW:P42043) At5g26030 AAD40138.1 20489_at M 1.42 4.64 11.72 3.57 1.95 NP Putative ethylene response At2g44840 AAC31840.1 element-binding protein (EREBP) 12002_at M 2.10 4.45 5.46 9.96 0.54 NP Hypothetical protein AT4g02940 AAC79112.1 13100_at M 2.66 3.42 3.11 3.29 1.20 NP Putative cytochrome P450 At2g45570 AAC06158.1 13219_at M 1.70 3.00 3.73 3.25 0.44 NP Class IV chitinase (CHIV) AT3g54420 CAA74930.1</p><p>20641_at M 0.04 11.76 4.08 1.00 9.28 PK LEA76 homologue type1 At1g52690 CAA63012.1 13949_at H 0.52 7.31 7.06 1.74 4.05 PK Thioesterase like protein AT4g17470 CAB10528.1 15141_s_at H 0.73 5.65 13.88 0.83 4.79 PK Vegetative storage protein AT5g24770 BAA22096.1 (D85191) 20096_at H 1.91 5.03 5.13 1.34 2.21 PK Putative berberine bridge At2g34810 AAC12821.1 enzyme 15389_at H 1.33 4.32 3.15 1.42 4.76 PK Unknown protein At2g22860 AAC32433.1 17187_at H 0.94 4.05 3.98 1.79 5.47 PK Similar to arginases AT4g08870 AAD17371.1 17843_s_at M 1.19 3.57 3.69 1.48 3.41 PK Putative cytochrome P450 At2g23220 AAB87109.1 14573_at M 0.52 3.34 3.24 0.72 2.61 PK T14P8.17 gene product AT4g02360 AAC19282.1</p><p>19672_at M 0.22 20.52 7.62 10.12 33.56 NPK RAP2.6 At1g43160 AAC36019.1 15531_i_at M 0.79 13.52 10.98 27.16 20.04 NPK Putative protein AT4g24340 CAB45069.1 18946_at M 0.96 12.88 4.85 16.46 18.58 NPK Peroxidase ATP24a AT5g39580 CAA72484.1 16073_f_at M 1.83 12.16 3.05 4.46 4.16 NPK Putative transcription factor At1g56650 AAC83630.1 (MYB75) 13009_i_at M 2.98 11.46 3.66 14.82 8.82 NPK Putative protein AT4g17950 CAA17138.1 15125_f_at H 0.34 9.77 24.71 3.14 10.04 NPK Vegetative storage protein AT5g24770 BAA22095.1 (D85190) 19150_at M 0.51 7.91 6.56 7.25 8.30 NPK PF|00657 type At1g54020 AAD25774.1 Lipase/Acylhydrolase with GDSL-motif 12746/8 M 0.47 5.84 7.65 2.84 4.73 NPK Drought-inducible cysteine AT4g11320 CAB51416.1 proteinase RD21A precursor- like protein 19186_s_at M 0.43 4.85 14.76 2.30 11.35 NPK Dehydrin (Xero 2) AT3g50970 AAB00374.1 16654_at M 1.15 4.44 3.59 5.97 4.50 NPK Hypothetical protein AT4g20010 CAA16609.1 18909_s_at M 2.80 3.83 4.95 3.39 4.56 NPK Subtilisin-like protease (AIR3) Not found AAC62611.1 15779_g_at L 2.44 3.62 3.40 4.14 10.12 NPK Zinc finger protein (Zat7) AT3g46090 CAA67234.1 16232_s_at M 0.79 3.46 9.74 6.12 6.76 NPK Putative protein At1g07160 CAB45796.1 20524_at M 0.62 3.34 3.94 7.24 4.89 NPK T3P18.12 gene product At1g62560 AAD43613.1 16510_at M 1.15 3.28 4.12 2.95 2.97 NPK Putative protein AT4g32480 CAA22575.1 15965_at M 1.05 3.28 3.91 6.28 4.61 NPK Jasmonate inducible protein At4g39090 AAB63631.1 isolog 14614_at H 2.13 3.17 3.02 3.25 3.68 NPK Putative glucosyltransferase At2g30140 AAC16958.1 19641_at M 2.19 3.03 5.49 3.40 3.13 NPK Putative glutathione S- At2g29490 AAC95189.1 transferase 15005_s_at L 1.08 3.02 3.62 3.26 7.18 NPK Putative protein kinase At2g30040 AAC31848.1</p><p>Table 4. Identities of the 37 genes upregulated at least threefold 28 and 100 h after P withdrawal, as shoot P concentration declined but before shoot growth was compromised, but not immediately following P withdrawal. Changes in gene expression following N, P or K starvation are expressed relative to controls grown in a complete nutrient solution. Qualitative estimates of absolute gene expression are classified as low (L), medium (M) and high (H), and the nutrient specificity of increased gene expression is summarised. The database of Ghassemian et al. (2001) was used to map Affymetrix GeneChip™ identifiers to Arabidopsis Genome Initiative (AGI) identifiers.</p><p>9 Project Changes in Gene Expression Profiles under Nutrient MAFF title Deficiency project code HH3502S FV</p><p>5. CONCLUSIONS</p><p>A. Thirty-seven genes were identified whose expression increased at least threefold as shoot P concentration declined, but before shoot growth was compromised, between 28 and 100 h after P was removed from the nutrient solution.</p><p>B. Four genes were identified whose expression was increased at least threefold as shoot P concentration declined, but was unaffected by N or K stress.</p><p>6. COMMUNICATION OF RESULTS</p><p>1. An abstract of the NASC transcriptome analyses will be published on the Genomic Arabidopsis Resource Network (GARNet) website at http://www.york.ac.uk/res/garnet/garnet.htm. 2. Results will be presented in papers at national and international scientific conferences, including: [1] J Hammond, PJ White, MJ Bennett, MR Broadley (2002) Abstract P7.18: Plants that make the most of phosphate. Society for Experimental Biology Annual Meeting, Swansea, April 2002. [2] J Hammond, M Bennett, M Broadley, P White (2002) Changes in gene expression upon nutrient removal and the development of novel sensor technologies. GARNet, York, September 2002. [3] J Hammond, MJ Bennett, MR Broadley, PJ White (2002) Gene expression and mineral nutrition. SEB Plant Transport Group, York, September 2002. 3. A high-impact refereed publication on "Changes in gene expression in Arabidopsis shoots under nutrient deficiency" is in preparation. 4. Illustrative data will be included in Dr White's lecture on "Mineral Nutrition of Higher Plants" in the HRI Applied Biotechnology Course, October 2002, and other HRI technology transfer events. 5. Results will be incorporated into the PhD thesis of John Hammond (University of Nottingham).</p><p>7. FUTURE PROSPECTS</p><p>In this short-term Project we have taken the first, but most important, step towards the development of novel biosensor technologies to help reduce P fertiliser application. Ultimately these will lower costs, reduce pollution and enhance biodiversity. The identification of specific nutrient-responsive genes will underpin the development of novel biosensor technologies for stress monitoring and crop improvement. These may include: (i) custom-designed DNA-arrays, to detect the transcripts of genes whose expression is altered by P starvation, (ii) traditional antibody-based assays that detect the presence of proteins accumulating when plants lack P, and (iii) 'smart plants', in which P-responsive promoters control the expression of genes encoding visible products whose abundance reflects plant P status. However, further research and development are required before these practical benefits can be realised.</p><p>DNA Arrays and Transcript Profiling</p><p>This Project has identified genes whose expression is altered following the withdrawal of the mineral nutrients P, N and K (Fig. 3). Knowledge of these nutrient-sensitive genes, whether their appearance or disappearance is specific or non-specific to the absence of a particular nutrient, can be used to design DNA-arrays for assaying mineral nutrient status of plants through transcript profiling. By comparing the relative expression of a small suite of genes that respond differently to plant mineral nutrient status, it is possible, theoretically, to diagnose the identity of a deficient mineral element. Thus, the mineral nutrient status of a plant could be assayed relatively rapidly in a small leaf sample. Such an assay is an exciting prospect, and immediately within our technological grasp.</p><p>10 Project Changes in Gene Expression Profiles under Nutrient MAFF title Deficiency project code HH3502S FV</p><p>It is important that transcript-profiling following the withdrawal of mineral nutrients is extended to crop plants. Now that P-responsive genes have been identified in Arabidopsis, it would be informative to ascertain whether homologous genes are also induced in Brassica upon P starvation. To effect this it may be possible to probe Arabidopsis microarrays with mRNA from Brassica (Girke et al. 2000). Alternatively a customised Brassica cDNA microarray could be produced to confirm the anticipated changes in gene expression in Brassica leaves following P withdrawal. These experiments would enable suitable DNA-arrays for assaying the mineral nutrient status of crop plants to be designed.</p><p>Immunoassays for Plant Mineral-Nutrient Status</p><p>In addition to their immediate utility in designing DNA-arrays for mRNA profiling, transcripts of any genes whose expression is upregulated by nutrient starvation could be expressed in heterologous systems to produce antigenic proteins. Antibodies to these proteins might form the basis of simple immunoassay kits for screening for plant mineral-nutrient status.</p><p>Smart Plants, and Transgenic Plants Tolerating Reduced P Inputs </p><p>In a 'proof of concept' study initiated by DEFRA (HH0915SFV), and taken forward through an ongoing HRI Browning Studentship to John Hammond (2000-2003), we have generated Arabidopsis lines expressing green fluorescent protein (GFP) and -glucuronidase (GUS) marker genes under the control of a specific P-responsive promoter (for the SQD1 gene). These plants show enhanced marker gene expression under P stress, and prove the concept of smart plants (Hammond et al. 2002). However, we identified a need to obtain alternative 'hyper- sensitive' P-specific promoters that were upregulated earlier than SQD1, and prior to 100 h following P withdrawal. The promoters of the four genes identified in this Project as being regulated specifically by P status would fulfil this requirement. Smart plants can be used as a basic research tool (e.g. in determining the role of root and/or soil structure in the acquisition of P) as well as for more applied ends (e.g. by providing a bioassay for P availability within soils). For example, smart plants could be used (i) in conjunction with a screen of mutants or ecotypes, to identify plants with enhanced nutrient use efficiency, which would be useful in breeding programs, (ii) following mutation of the transgenic lines to identify components of signaling cascades impacting on nutrient- specific gene regulation, (iii) as experimental material to assist and ratify computer predictions for the application of fertiliser and (iv) as a model bioassay for rapid assessment of plant available P in situations where existing chemical tests provide unclear results. Many downstream applications can be envisaged for such smart plant technology when transferred to horticultural crops. Marker plants that indicate plant nutrient status will allow efficient temporal and spatial fertiliser applications and further the development of decision-making systems for precision farming. In addition to enabling us to identify four new, and specific, P-responsive promoters, the data produced in this Project could presage the development of smart plants for both N and K nutrition, pending further analysis and experimentation. Transcription factors whose expression is regulated by plant nutrient status can be identified directly from the microarray data collected in this Project (Table 3). In addition, a comparison of the promoter sequences of genes expressed under specific conditions would enable the identification of potential cis-acting DNA elements regulating gene expression, as well as confirming the target genes for known transcription factors (Harmer et al. 2000; Maleck et al. 2000; Petersen et al. 2000; Ghassemian et al. 2001; Seki et al. 2001; Reymond, 2001; Zhu et al. 2001; Chen et al. 2002, Wu et al., 2002). In the future, regulating the expression of transcription factors and harnessing cis-acting DNA elements to particular beneficial genes could be used to control a suite of genes that effect tolerance of low fertiliser inputs.</p><p>11 Project Changes in Gene Expression Profiles under Nutrient MAFF title Deficiency project code HH3502S FV</p><p>8. REFERENCES</p><p>Bollons HM, Barraclough PB (1997) Inorganic orthophosphate for diagnosing the phosphorus status of wheat plants. Journal of Plant Nutrition 20, 641-655. Bollons HM, Barraclough PB (1999) Assessing the phosphorus status of winter wheat crops: inorganic orthophosphate in whole shoots. Journal of Agricultural Science 133, 285-295. Bray EA, Bailey-Serres J, Weretilnyk E (2000) Responses to abiotic stresses. In: Biochemistry & Molecular Biology of Plants, pp. 1158-1203. B Buchanan, W Gruissem, R Jones, eds. American Society of Plant Physiologists. Chen W et al. (2002) Expression profile matrix of Arabidopsis transcription factor genes suggests their putative functions in response to environmental stresses. Plant Cell 14, 559-574. Desprez T, Amselem J, Caboche M, Höfte H (1998) Differential gene expression in Arabidopsis monitored using cDNA arrays. Plant Journal 14, 643-652. Gamas P, de Carvalho Niebel F, Lescure N, Cullimore J (1996) Use of a subtractive hybridization approach to identify new Medicago truncatula genes induced during root nodule development. Molecular Plant Microbe Interactions 9, 233-242. Ghassemian M, Waner D, Tchieu J, Gribskov M, Schroeder JI (2001) An integrated Arabidopsis annotation database for Affymetrix Genechip® data analysis, and tools for regulatory motif searches. Trends in Plant Sciences 6, 448-449. Girke T, Todd J, Ruuska S, White J, Benning C, Ohlrogge J (2000) Microarray analysis of developing Arabidopsis seeds. Plant Physiology 124, 1570-1581. Hammond J, White PJ, Bennett MJ, Broadley MR (2002) Plants that make the most of phosphate. Society for Experimental Biology Annual Meeting, Swansea, April 2002. [Abstract P7.18] Harmer SL, Hogenesch JB, Straume M, Chang H-S, Han B, Zhu T, Wang X, Kreps JA, Kay SA (2000) Orchestrated transcription of key pathways in Arabidopsis by the circadian clock. Science 290, 2110- 2113. Hurry V, Strand Ǻ, Furbank R, Stitt M (2000) The role of inorganic phosphate in the development of freezing tolerance and the acclimatization of photosynthesis to low temperature is revealed by the pho mutants of Arabidopsis thaliana. Plant Journal 24, 383-396. Kehoe DM, Villand P, Somerville S (1999) DNA microarrays for studies of higher plants and other photosynthetic organisms. Trends in Plant Science 4, 38-41. Ma LG, Li JM, Qu LJ, Hager J, Chen ZL, Zhao HY, Deng XW (2001) Light control of Arabidopsis development entails coordinated regulation of genome expression and cellular pathways. Plant Cell 13, 2589-2607. Maleck K, Levine A, Eulgem T, Morgan A, Schmid J, Lawton KA, Dangl JL, Dietrich RA (2000) The transcriptome of Arabidopsis thaliana during systemic acquired resistance. Nature Genetics 26, 403- 410. Murashige T, Skoog F (1962) A revised medium for rapid growth and bioassays with tobacco tissue cultures. Physiologia Plantarum 15, 473-497. Petersen M, Brodersen P, Naested H, Andreasson E, Lindhart U, Johansen B, Nielsen HB, Lacy M, Austin MJ, Parker JE, Sharma SB, Klessig DF, Martienssen R, Mattsson O, Jensen AB, Mundy J (2000) Arabidopsis MAP kinase 4 negatively regulates systemic acquired resistance. Cell 103, 1111-1120. Raynal M, Guilleminot J, Gueguen C, Cooke R, Delseny M, Gruber V (1999) Structure, organization and expression of two closely related novel Lea (late-embryogenesis-abundant) genes in Arabidopsis thaliana. Plant Molecular Biology 40, 153-163. Reymond P (2001) DNA microarrays and plant defence. Plant Physiology & Biochemistry 39, 131-321. Ruan Y, Gilmore J, Conner T (1998) Towards Arabidopsis genome analysis: monitoring expression profiles of 1400 genes using cDNA microarrays. Plant Journal 15, 821-833. Schaffer R, Landgraf J, Accerbi M, Simon V, Larson M, Wisman E (2001) Microarray analysis of diurnal and circadian-regulated genes in Arabidopsis. Plant Cell 13, 113-123.</p><p>12 Project Changes in Gene Expression Profiles under Nutrient MAFF title Deficiency project code HH3502S FV</p><p>Seki M, Narusaka M, Abe H, Kasuga M, Yamaguchi-Shinozaki K, Carninci P, Hayashizaki Y, Shinozaki K (2001) Monitoring the expression pattern of 1300 Arabidopsis genes under drought and cold stresses by using a full-length cDNA microarray. Plant Cell 13, 61-72. Wang R, Guegler K, LaBrie ST, Crawford NM (2000) Genomic analysis of a nutrient response in Arabidopsis reveals diverse expression patterns and novel metabolic and potential regulatory genes induced by nitrate. Plant Cell 12, 1491-1509. Wang YH, Garvin DF, Kochian LV (2001) Nitrate-induced genes in tomato roots. Array analysis reveals novel genes that may play a role in nitrogen nutrition. Plant Physiology 127, 345-359. White PJ, Rahn CR (1999) A first step to ‘smart’ plants: isolating nutrient responsive promoters. Final Report on MAFF Project HH0915SFV, 6 pp. Wu K, Tian L, Hollingworth J, Brown DCW, Miki B (2002) Functional analysis of tomato Pti4 in Arabidopsis. Plant Physiology 128, 30-37. Zhu T, Budworth P, Han B, Brown D, Chang HS, Zou GZ, Wang X (2001) Toward elucidating the global gene expression patterns of developing Arabidopsis: Parallel analysis of 8 300 genes by a high-density oligonucleotide probe array. Plant Physiology and Biochemistry 39, 221-242. Zhu T, Wang X (2000) Large-scale profiling of the Arabidopsis transcriptome. Plant Physiology 124, 1472- 1476.</p><p>13 Project Changes in Gene Expression Profiles under Nutrient MAFF title Deficiency project code HH3502S FV</p><p>Please press enter</p><p>14</p>
Details
-
File Typepdf
-
Upload Time-
-
Content LanguagesEnglish
-
Upload UserAnonymous/Not logged-in
-
File Pages14 Page
-
File Size-