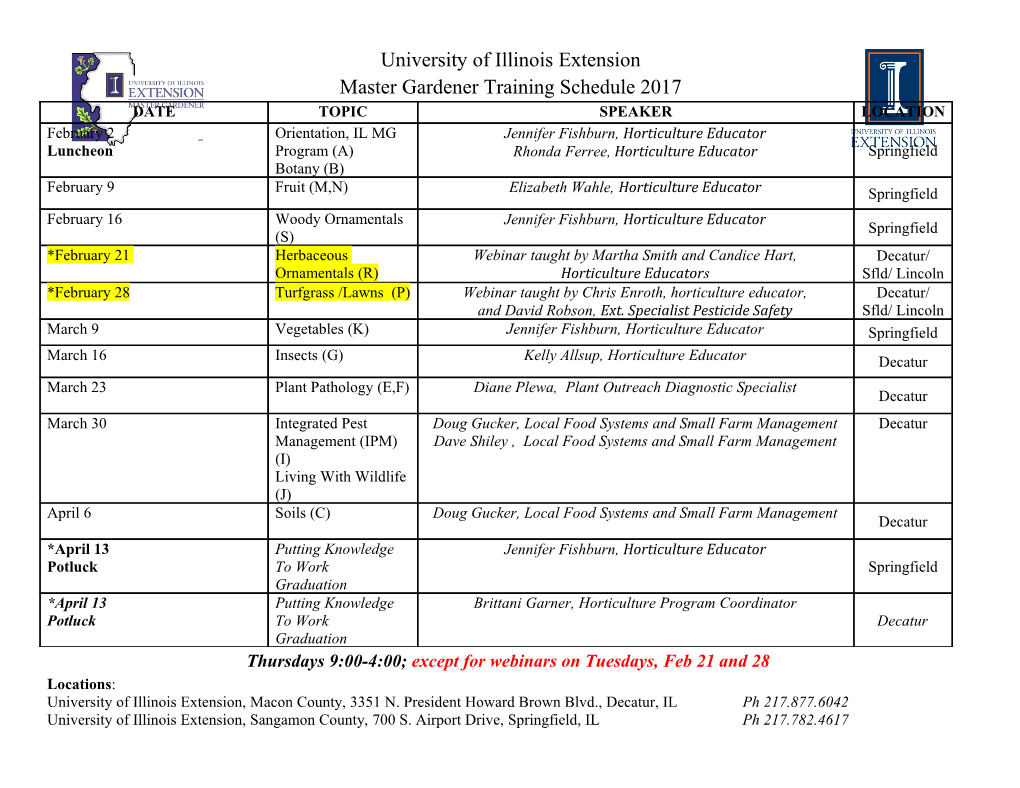
<p>Supplementary Information</p><p>METHODS Unless otherwise specified, all physico-chemical characterisations were carried out at room temperature. Sample homogeneity was ensured by thoroughly blending the batches of sample received before sub-sampling. For each measurement, samples were taken from the sub-samples randomly and measurements were performed at least in duplicate. In some cases, e.g. for surface area, TGA, TEM, DLS and zeta potential, tests were performed on the same sample batch at different laboratories and by different operators, yielding comparable results.</p><p>Crystalline phase. Samples were prepared by packing approximately 0.5 g of solid material in a plastic sample holder and flattening the surface with a glass slide. The crystalline phase was determined by analysis of the diffraction pattern produced upon exposure of the sample to x-rays generated by a Bruker ASX-D8 X-Ray </p><p>Diffractometer (XRD) using Cu K radiation and an operating current of 40 mA and voltage of 40 kV. The scan ranged from 20 o to 150 o with a step size of 0.005 o. The size of the aperture slit directing the X-ray source was 0.2 mm. Particle morphology. The morphology of each sample was assessed by analysis of Transmission Electron Microscopy (TEM) and Scanning Electron Microscopy (SEM) images. Samples for TEM were prepared by briefly sonicating a few milligrams of the solid material in approximately 20 µl ethanol to form a milky dispersion. Carbon- coated grids (copper, 300 mesh) were glow discharged in nitrogen for 30 s to render them hydrophilic. 5 µl of dispersion was applied to the freshly-prepared grids. After 2 minutes, excess dispersion was wicked off using filter paper (Whatman 541) and the grids were dried in air for 15 minutes. Grids were examined at eucentric height using an FEI Tecnai 12 TEM operating at 120 kV, and micrographs were recorded using an Olympus Megaview III CCD camera running AnalySiS imaging software (Olympus). The maximum and minimum “Feret” diameters were used as a measure of particle length and width and were determined from TEM images using a semi-automated particle size analysis program (ImageJ/Fiji) (Igathinathane et al. 2012). Approximately 100 particles across the TEM grid were measured for each sample which came from more than 10 images for NM-110 and NM-111, 5 for NM-112 and >20 for NM113. This ensured that a representative set of images were acquired. To minimise uncertainty </p><p>1 caused by overlapping particles, only particles that were separated or could be clearly defined were included in the analysis of maximum and minimum Feret diameter. In addition, two operators performed measurements using the same software. The final data reported here were the average values provided by the two operators. Samples for SEM were prepared by sprinkling ~5 mg of ZnO powder over adhesive conducting tape covering an SEM metal stub. The surface of the powder sample was flattened with a spatula, and excess powder was removed by gently tapping the stub on its side until a light coating of powder on the surface became apparent. The nanoparticles were coated with iridium using a Polaron SC570 sputter coater. Sputtering was conducted under vacuum while the passing gas was argon. The coating deposition time was 20 s at a plate current of 50 mA, giving a coating thickness of approximately 1 nm. Images were obtained with a Philips XL30 field emission SEM. The optimal spatial resolution of the microscope was 2–5 nm with varying accelerating voltage from 30 kV to 1 kV. Images of ZnO particles were acquired at an accelerating voltage of 5 kV, a working distance of approximately 10 mm, and a tilt angle of 0 °.</p><p>Specific surface area and porosity. Specific surface areas of the twelve ZnO samples were determined by the Brunauer-Emmett-Teller (BET) using a Micromeritics Tristar II 3020 instrument, which uses physical gas adsorption and capillary condensation principles to obtain information about the surface area. Prior to analysis, the powdered sample was transferred to a sample bulb which was de- gassed overnight at 300 C under high vacuum and subsequently weighed on an analytical balance in order to determine the sample mass after the degassing step. The sample tube containing the degassed sample was cooled by immersion in liquid nitrogen to 77 K and exposed to the adsorbate gas (nitrogen, ultra high purity 99.999 %) at 11 controlled pressures. Porosity was determined simultaneously with surface area by applying the Barrett-Joyner-Halenda (BJH) method for analysing gas adsorption and desorption isotherms to determine the total pore volume.</p><p>Elemental composition. To prepare samples for the determination of elemental composition, approximately 0.15 g of ZnO sample was dissolved in a 1:1 HNO3:H2O2 mixture with heating for 30 minutes. The solution was diluted to 100 mL, 10 µg ml-1 of Scandium Standard (Ultra Scientific Analytical Solutions) was added as an internal </p><p>2 standard, and the resultant solution was analysed by Inductively Coupled Plasma- Atomic Emission Spectroscopy (Varian 730 Axial ICP-AES). Certified multi-element solutions (QCD Analysts) were used to check accuracy. Elemental analysis of the surface. The elemental composition within 1–10 nm of the surface of the samples was quantitatively determined in duplicate by X-ray photoelectron spectroscopy (XPS). Powdered ZnO samples were placed in individual wells of a sample holder and irradiated with X-rays under ultra-high vacuum using a Kratos HS spectrometer, fitted with a monochromated Al Kα source, operating under standard conditions. The sampling area was approximately 0.3 mm by 0.7 mm. Wide scan survey spectra were recorded to identify and quantify all elements present on the surface. Thermal stability. Thermo-gravimetric analysis (TGA) of all ZnO batches was performed on a Perkin Elmer STA6000 instrument in duplicate. Roughly 50 mg of sample was heated in a ceramic crucible from 50 oC to 1000 oC at a rate of </p><p> o -1 -1 10 C min , under a 20 ml min flow of N2 gas. Volatile species evolved during heating were passed through a heated transfer line into the gas cell of a Fourier- transform infra-red spectroscopy system (FT-IR, Perkin Elmer Frontier) for analysis. Differential centrifugal sedimentation. To investigate the particle size distribution, differential centrifugal sedimentation (DCS) measurements were performed using a CPS Instruments 24000UHR disk centrifuge. Measurements were made at a fixed speed of 8900 rpm to access the distribution from 20–1000 nm, and, using the speed ramping mode, with speeds between 1000 and 12 000 rpm, to access the distribution from 0.02–10 µm. 17 ml of a gradient fluid comprising 8–24 % w/w sucrose (99 %, Sigma Aldrich) in ultrapure water (Milli-Q, 18.2 MΩ cm) with a 0.5 ml dodecane evaporation cap was used for all measurements. A 0.377 µm nominal mean diameter calibration standard (polyvinyl chloride, PS Instrument) was used. 0.1 ml of sample was injected for each run. The samples for fixed-speed measurements were 0.015 % w/w suspensions of ZnO in ultrapure water. 15 mg of test powder was added to a clean, 20 ml glass vial, and mixed with a spatula with ~1 ml of ultrapure water. More dispersant was added to create a final volume of 15 ml. This sample was then ultrasonicated using a high-power ultrasonic horn (Misonix 3000) for 20 s with a 2 s pulsed duty cycle with a 1.3 cm tip at an amplitude of 20%. Following ultrasonication, the sample was transferred to a 150 ml beaker and 85 ml of ultrapure water was added to produce a 100 ml dispersion. The </p><p>3 resultant suspension was stirred with an overhead stirrer for at least 15 minutes prior to measurement. The samples for speed ramping measurements were 1 % w/w suspensions prepared by directly adding 1 g of ZnO to 99 ml of ultrapure water and stirring with an overhead stirrer for 15 minutes. Probe sonication was then applied to the sample using a 1.3 cm ultrasonic horn tip at 100 % amplitude with a 2 s pulsed duty cycle. The sample was first sonicated for 15 s, measured, left to stir, sonicated for a further 15 s (total sonication time: 30 s), measured, left to stir, sonicated for a further 30 s (total sonication time: 60 s), and measured. Dynamic light scattering (DLS). Measurements of z-average hydrodynamic particle diameter in deionised water (Milli-Q, 18.2 MΩ cm) by DLS were obtained for all samples using a Brookhaven particle size analyzer 90Plus equipped with a 633 nm laser. The scattering angle in this instrument is 90 °. Reference standards (Duke polystyrene latex with a nominal diameter of 100 nm, and NIST RM8013 gold nanoparticles with a nominal diameter of 60 nm) were used to verify the performance of the instrument. 10 mg ZnO particles were added to a measuring cuvette containing 3 ml of deionised water. The cuvette was placed in an ultrasonic bath (Branson 3510, 100 W, 42 kHz) for 10 s, and further shaken by hand to check the particles were well dispersed before starting the DLS measurements. For the uncoated products, the uniform colloidal dispersion obtained after sonication was used directly for measurements. Due to the highly hydrophobic surface on particles of Z- COTE HP1, only some were successfully dispersed while most remained floating on top of the water (as shown in Figure 5). To avoid any influence from the undispersed particles, a pasteur pipette was used to transfer the dispersion to another cuvette for further measurement without additional sonication. Each measurement was based on 10 sub- runs which were averaged to produce the reported result. Duplicate experiments were performed on each sample. The temperature was maintained at 25 oC during measurement. The cuvette was thoroughly washed with deionised water before and after each measurement. Electrophoretic-mobility measurements of zeta potential (surface charge). A Malvern Zetasizer Nano Z system was used for zeta-potential measurements. 10 mg of each ZnO sample was placed in a plastic tube containing 3 ml dispersion medium. The dispersion medium was prepared in advance and is based on deionised water with the pH (pH=2, 4, 6, 8, 10) adjusted by adding 0.1 M HCl or 0.1 M NaOH. The tube was placed in an ultrasonic </p><p>4 bath (Branson 3510, 100 W, 42 kHz) for 10 s and then shaken manually to check dispersion. For uncoated ZnO products, the uniform colloidal dispersion after sonication was used directly for zeta potential measurements. Dispersed samples of Z-COTE HP1 were prepared as described above for DLS measurements. One ml of the dispersion was injected into a DTS 1060 cuvette. Five measurements were performed at each pH to determine the average and standard deviation. The temperature of all measurements was maintained at 25 oC. The cuvette was thoroughly washed with deionised water before and after each measurement. Photo catalytic activity. Two 62.5 ml mixtures of 1:1 Mineral Oil White Light (Aldrich):Caprylic Capric C8/C10 Triglyceride (MOTG) were prepared. ZnO (31 mg) was added to one mixture, and DPPH (5.2 mg) to the other. Each was magnetically stirred for 1.5 hours in a beaker covered on all sides with Al foil. Then the two mixtures were combined, poured into a crystallising dish (135 mm diameter x 23 mm height) covered on all sides with foil, and magnetically stirred for 5 minutes. Before exposure to UV (t = 0), 3 ml of the solution was withdrawn and its UV-Vis absorption spectrum was measured using a Cary 5G UV-Vis NIR spectrophotometer. The 3 ml sample was returned to the solution. The solution was then exposed to UV using a pre-warmed Spectroline UV lamp (BIB150 P/FA 365 nm, 150 W concentrated spot bulb, lamp diameter: 110 mm). The lamp was placed 12 cm above the ZnO/dye mixture, at a position where the 365 nm intensity was 45 mW cm2. Samples were taken at various times and absorbance at 520 nm was measured. The equipment was designed with a sliding shield separating the sample and UV-lamp so that between exposures the lamp remained on, thus avoiding variations in lamp intensity. Dissolution measurements. The dissolution of each ZnO sample was measured using the equilibrium dialysis method (Angel et al. 2013). Dialysis membranes had a molecular mass cut-off of 1 kDa (Cole Parmer Spectra/Por 7). The membranes were cut into 10 cm lengths, washed with deionised water (Milli-Q, 18.2 MΩ cm) filled with </p><p>20 ml of Milli-Q water and sealed with acid-washed (1 % v/v HNO3) plastic dialysis clips. The total volume of the dialysis cells was kept to below 5 % of the test solution in order to minimise dilution effects. At the start of the test, 50 mg l-1 of each ZnO sample was prepared in triplicate by dispersing in 3 L of 0.01 M Ca(NO3)2 buffered with 2 mM piperazine-N,N’-bisethanesulfonic acid (PIPES: Sigma-Aldrich) to pH 7.5 ± 0.1. The dialysis cells were immediately added and the solutions were </p><p>5 continuously stirred with PTFE magnetic stirrers for 72 hours at constant temperature (21 oC). At sampling times of 24, 48 and 72 hours, a dialysis cell from each treatment replicate was removed from the solution, rinsed with Milli-Q water and sub-sampled for the dialysed Zn concentration. At the same time, a filtered (0.1 µm) sample was also taken from the bulk solution and analysed for Zn concentration for comparison with the dialysed sample. An additional filtered (0.1 µm) sample from the bulk solution was taken at 8 hours. Samples from dialysis cells and from bulk solutions (0.1 µm filtered) showed no differences in levels of dissolved zinc at any time point, indicating that any agglomerated ZnO in the bulk solution was larger than 100 nm. The pHs of all solutions increased marginally after 72 hours to the range 7.56 -7.68. Dispersion of the NM-111 batch of Z-COTE HP1 in water. 20–33 mg of various samples of the NM-111 batch of Z-COTE HP1 were weighed into glass bottles, and 5 ml of Milli-Q water was added. The samples were bath sonicated for 3 minutes. After standing for a few minutes, the samples were illuminated with a white-LED torch from the side, and images were taken with a Nikon D5000 digital camera. Scattered light in the images indicated those samples with particles in suspension. Various samples of the same batch, NM-111, had experienced slightly different storage histories: Sample 1: Stored in original glass bottle, first opened in 2009 (only once or twice). Sample 2: Sub-sampled (~2 g) from Sample 1 in 2009, stored in plastic bottle. Sample 3: Sub-sampled from Sample 2 on the day of the described experiment, heated at 100 oC for 1.5 hours and cooled. Sample 4: Stored in original glass bottle, opened September 2012 and December 2012. Sample 5: Stored in original glass bottle, opened September 2013, otherwise unknown history. Sample 6: Stored in original glass bottle, opened December 2013 (once only). Sample 7: Stored in original glass bottle, opened on the day of the described experiment only.</p><p>6 Figure S1: X-ray diffraction spectra from the OECD batches, NM-110, NM-111, NM-112 and NM-113, of the four respective ZnO products, Z-COTE, Z-COTE HP1, Nanosun and bulk ZnO. The spectra reveal that the only detected phase is hexagonal wurtzite zincite. Other batches of the three nano-sized ZnO products display similar characteristics. The broader peak widths for NM-112 indicate a smaller crystallite size compared with the other OECD samples.</p><p>7</p><p>Figure S2: Typical SEM images of the OECD batches, NM-110, NM-111, NM-112 and NM- 113. The length of all scale bars is 500 nm.</p><p>8 (a) (b)</p><p>0.9 1.4 NM110 adsorption adsorption 0.8 NM111 desorption desorption ) ) 1.2 0.7 g g / / l l o o m m 0.6 1.0 m m ( (</p><p> d d 0.5 e</p><p> e 0.8 b b r r</p><p> o o 0.4 s s d</p><p> d 0.6 A A</p><p>0.3 y y t t i i t t 0.4 n n 0.2 a a u u Q Q 0.2 0.1</p><p>0.0 0.0 0.0 0.1 0.2 0.3 0.4 0.5 0.6 0.7 0.8 0.9 1.0 0.0 0.1 0.2 0.3 0.4 0.5 0.6 0.7 0.8 0.9 1.0 Relative Pressure (p/p0) Relative Pressure (p/p0)</p><p>(c) (d)</p><p>0.30 3.0 NM113 adsorption NM112 adsorption desorption 0.25 2.5 desorption ) ) g / l g / l o o</p><p> m 0.20</p><p> m 2.0 m ( m</p><p>(</p><p> d d e e</p><p> b 0.15 r b 1.5</p><p> r</p><p> o o s s d d A</p><p>A</p><p>0.10</p><p>1.0 y y t i t i t t n n a a u u 0.5 0.05 Q Q</p><p>0.0 0.00 0.0 0.1 0.2 0.3 0.4 0.5 0.6 0.7 0.8 0.9 1.0 0.0 0.1 0.2 0.3 0.4 0.5 0.6 0.7 0.8 0.9 1.0 Relative Pressure (p/p0) Relative Pressure (p/p0)</p><p>Figure S3: Typical isothermal plots of OECD batches of the ZnO products (a) NM-110 (Z- COTE), (b) NM-111 (Z-COTE HP1), (c) NM-112 (Nanosun) and (d) NM-113 (bulk ZnO), obtained from the BET method for gas adsorption and desorption. Other batches of these products show similar results. The limited amount of gas adsorbed/desorbed at lower pressures indicates that micropores contribute little to the total surface area. Black circles and red crosses represent data for adsorption and desorption, respectively</p><p>9 Figure S4 TGA curves of different batches of the three nano-sized ZnO products, as indicated by percentage mass changes when heated from 50 to 1000 oC at a rate of </p><p>10 10 oC min-1 under a nitrogen-gas flow rate of 20 ml min-1. (a) Z-COTE, (b) Z-COTE HP1, (c) Nanosun.</p><p>Table S1 Crystallite size with fitting error and lattice strain calculated from XRD spectra Product name Batch No. Crystallite size (nm) Strain Z-COTE OECD NM-110 69.3 ± 2.4 0.0009 CIAJ1004 70.1 ± 2.4 0.0010 EHHC1010 61.1 ± 2.1 0.0000 EHDA3001 65.0 ± 2.1 0.0003 Average ± SD 66.4 ± 4.2 0.0006 Z-COTE HP1 OECD NM-111 51.2 ± 1.5 0.0000 CNHH1902 58.0 ± 2.4 0.0010 CNHE0602 48.1 ± 1.2 0.0001 Average ± SD 52.4 ± 5.1 0.0004 Nanosun OECD NM-112 23.4 ± 1.2 0.0071 3817 23.4 ± 1.2 0.0088 5672 23.5 ± 1.2 0.0090 4051 24.2 ± 1.2 0.0085 Average ± SD 23.6 ± 0.4 0.0083 ZnO (bulk) OECD NM-113* 156.8 ± 18.9 0.0000 * Rough estimation, as the Williamson-Hall equation is for particles under 100 nm in size.</p><p>Table S2. Z-average hydrodynamic diameters and polydispersity indices (PDI) for various batches of Z-COTE, Z-COTE HP1, Nanosun and bulk ZnO dispersed in deionised water and measured by dynamic light scattering (DLS). Product Batch No. Z-average PDI name hydrodynamic diameter (nm) Z-COTE OECD NM-110 340 0.2 CIAJ1004 500 0.2 EHHC1010 360 0.2 EHDA3001 440 0.2</p><p>11 Z-COTE HP1 OECD NM-111 192 0.2 CNHH1902 201 0.2 CNHE0602 189 0.2 Nanosun OECD NM-112 440 0.3 3817 290 0.3 5672 370 0.3 4051 460 0.3 ZnO (bulk) OECD NM-113 470 0.2</p><p>12 Table S3. Dissolution of various batches of Z-COTE, Z-COTE HP1, Nanosun and bulk ZnO in aqueous 10 mM calcium nitrate, buffered at pH 7.5. Product Batch Number Equilibrium % dissolution* name solubility^ (µg l-1) ± SD Z-COTE OECD NM-110 5800 ± 240 14.4 EHHC1010 5770 ± 200 14.4 EHDA3001 5440 ± 140 13.5 CIAJ1004 5810 ± 160 14.5 Average 5705 14.2 Z-COTE H OECD NM-111 5260 ± 310 13.1 P1 CNHH1902 5240 ± 400 13.1 CNHE0602 5140 ± 180 12.8 Average 5213 13.0 Nanosun OECD NM-112 5330 ± 220 13.3 5672 4110 ± 190 10.2 4051 5470 ± 130 13.6 3817 5010 ± 80 12.5 Average 4980 12.4 ZnO OECD NM-113 5090 ± 180 12.7 (bulk) ^Mean equilibrium solubility (at 72 hours) from 3 replicate experiments. * % dissolution is the dissolved Zn relative to total Zn in each sample.</p><p>Calculations of bulk atomic ratios of Si/Zn in Z-COTE HP1</p><p>13 1) from ICP-AES data (Table 3) Atomic ratio Si/Zn = where Δm1 is the mass percentage of Si (for example 1685 ppm = 0.1685 %; 915 ppm = 0.0915 % and 660 ppm = 0.066 %), Δm2 is the mass percentage of Zn, M1 and M2 are the atomic masses of Si (28) and Zn (65), respectively. OECD NM-111: atomic ratio Si/Zn = = 0.00498 CNHH1902: atomic ratio Si/Zn = = 0.00267 CNHE0602: atomic ratio Si/Zn = = 0.00194</p><p>2) from TGA data (Table 5) Atomic ratio Si/Zn= where Δm1 is the mass of surface coating and was calculated by the percentage of total mass loss of Z-COTE HP1 sample minus the average mass loss of all Z- COTE samples (0.84 %), Δm2 is the weight remaining for ZnO (100 % - 0.84 % = 99.16 %). M1 and M2 are the molecular massess of surface coating (trithoxycaprylysilane, 276.49 g mol-1) and ZnO (81 g mol-1), respectively. OECD NM-111: atomic ratio Si/Zn==0.00473 CNHH1902: atomic ratio Si/Zn==0.00366 CNHE0602: atomic ratio Si/Zn==0.00387 Surface Si/Zn atomic ratios found from XPS are higher because the analysis depth of XPS is only several nanometres. Si/Zn atomic ratios calculated from TGA, ICP-AES and XPS are slightly different as a result of the very different techniques used to measure data and/or assumptions required. However, data from all three methods consistently indicate that the OECD batch of Z-COTE HP1, NM- 111, has a relatively greater amount of surface coating compared with the other two batches. </p><p>The theoretical Si/Zn atomic ratio of triethoxycaprylylsilane on a Wurtzite ZnO structure is estimated as 6.63 x 10-4 x specific surface area of ZnO nanoparticles. For Z-COTE HP1 products, the average surface area is 12.4 m2 g-1. So the theoretical Si/Zn atomic ratio is 0.0082. Therefore the percentage surface coverage of silane on Z-COTE HP1 was calculated as below. 1) from ICP-AES data OECD NM-111 = 100 x 0.00498/0.0082 = 60.7 % CNHH1902 = 100 x 0.00267/0.0082 = 32.6 % CNHE0602 = 100 x 0.00194/0.0082 = 23.7 % 2) from TGA data OECD NM-111 = 100 x 0.00473/0.0082 = 57.7 % CNHH1902 = 100 x 0.00366/0.0082 = 44.6 % CNHE0602 = 100 x 0.00387/0.0082 = 47.2 % In OECD NM-111, with the highest amount of surface coating, the coverage is around 60 %. These calculations correlate well with the differences in photoactivity (around 57 %) </p><p>14 between the uncoated NM-110 (decay time 7.7 min) and the coated NM-111 (decay time 12.1 min). The incomplete surface coverage on NM-111 mitigates, but does not eliminate, the photocatalytic activity of uncoated ZnO. </p><p>References</p><p>Angel BM, Batley GE, Jarolimek CV, Rogers NJ (2013) The impact of size on the fate and toxicity of nanoparticulate silver in aquatic systems. Chemosphere 93:359–365 Igathinathane C, Ulusoy U, Pordesimo LO (2012) Comparison of particle size distribution of celestite mineral by machine vision Sigma Volume approach and mechanical sieving. Powder Technol 215–16:137–146</p><p>15</p>
Details
-
File Typepdf
-
Upload Time-
-
Content LanguagesEnglish
-
Upload UserAnonymous/Not logged-in
-
File Pages15 Page
-
File Size-