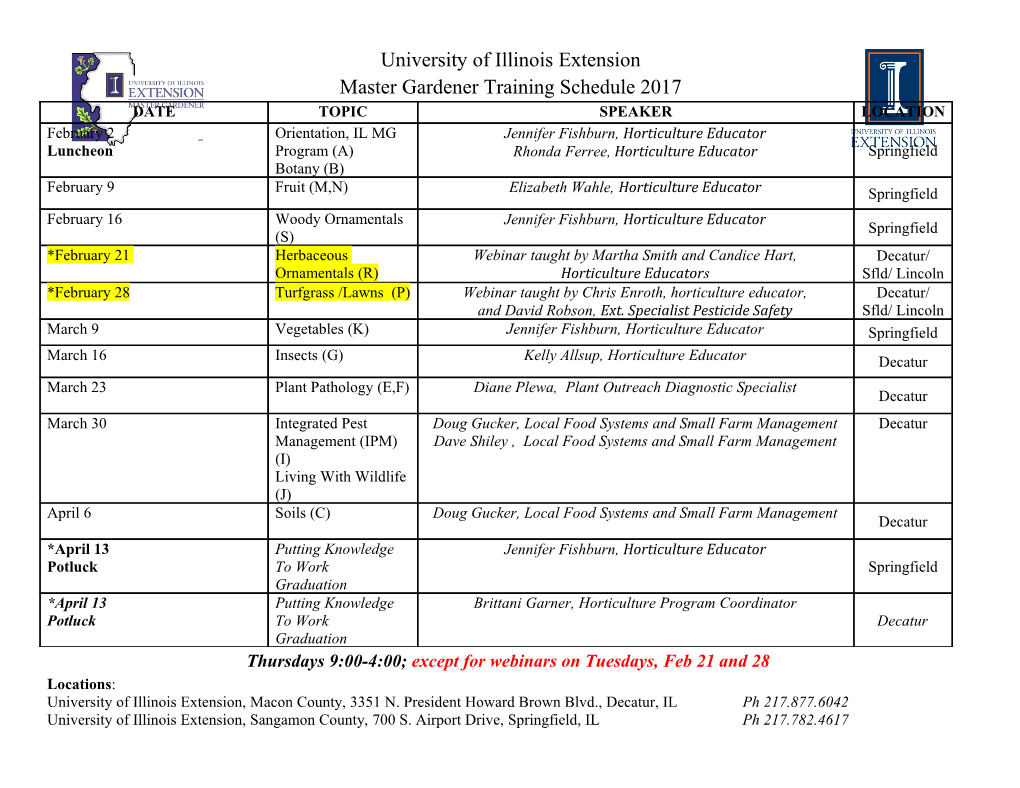
<p> 1SUPPORTING INFORMATIONS</p><p>2</p><p>3 fM to aM nucleic acid amplification for molecular diagnostics in a</p><p>4 silicone-coated metal microfluidic bioreactor</p><p>5</p><p>6 Guoliang Huang 1,2, Qin Huang 2, Li Ma2, Xianbo Luo2, Biao Pang2, Zhixin Zhang2, Yuliang Wang1, 7 Junqi Zhang1, Qi Li1, Rongxin Fu1, and Jiancheng Ye1</p><p>8</p><p>9 1Department of Biomedical Engineering, the School of Medicine, Tsinghua University, Beijing</p><p>10 100084, China.</p><p>11 2National Engineering Research Center for Beijing Biochip Technology, Beijing 102206, China.</p><p>12 Correspondence and requests for materials should be addressed to G.L.H. ([email protected])</p><p>1 1 1Supporting Information</p><p>2Results</p><p>3Comparison of isothermal DNA amplification in uncoated and non-stick-coated metal micro-</p><p>4nanoliter fluidic chips. Using the same DNA template concentration of 1.3 fM (10-15 M) and 7-μ L</p><p>5reaction mixtures in every bioreactor cell, isothermal DNA amplification in uncoated and non-stick</p><p>6coating metal micro-nanoliter fluidic chips was performed using our portable confocal detector. The</p><p>7contrast of DNA isothermal amplification curves is shown in Figure S1. Figure S1 (a) corresponds to</p><p>8isothermal DNA amplification in the uncoated metal micro-nanoliter fluidic chip, where the times at</p><p>9the second derivative inflexions of the exponential DNA amplification curves for the five bioreactor</p><p>10cells were 24.85, 27.29, 27.80, 28.18, and 29.53 min. The maximum of the time difference at these</p><p>11inflexions was ~4.68 min. At the top of the amplification curves, the fluorescence intensities of the</p><p>12five bioreactor cells ranged from 3600-6900; the percent deviation of the fluorescence intensity was</p><p>13~91.7%. Figure S1 (b) is isothermal DNA amplification in the non-stick-coated metal micro-</p><p>14nanoliter fluidic chip, where the times at the second derivative inflexions of the exponential DNA</p><p>15amplification curves for the five bioreactor cells corresponded to 19.42, 19.45, 19.59, 19.60, and</p><p>1619.92 min. The maximum of the time differences at these inflexions was 0.5 min. At the top of the</p><p>17amplification curves, the fluorescence intensities of the five bioreactor cells ranged from 4000-4500;</p><p>18the percent deviation of the fluorescence intensity was ~11%. Figure S1 (c) is the normalizing</p><p>19processing of the isothermal DNA amplification curves in Figure S1 (b), which is generally used in</p><p>20commercial RT-PCR setups.</p><p>21</p><p>1 2 (a)</p><p>1</p><p>(b)</p><p>(c)</p><p>1 3 1 2 Figure S1 3 4 Table S1</p><p>5 DNA template (fM) 1.3×102 1.3×101 1.3×100 1.3×10-1 1.3×10-2 1.3×10-3</p><p>Time at the inflexion (min) In 7-μL non-stick-coated metal micro-nanoliter fluidic bioreactors measured using our 14.8 17.4 19.6 21.5 24.5 28.7 Portable Confocal Detection System In 25-μL Eppendorf tubes non measured using an ABI 7700 16.3 18.4 20.8 23.7 28.9 amplifi Fast Real-Time PCR System cation 6</p><p>7DISCUSSION</p><p>8 The fluorescent detection response of nucleic acid amplification is related to many factors,</p><p>9including the number of amplified double-stranded DNAs (dsDNAs), the number of denatured</p><p>10dsDNAs at the amplification temperature, the surface adsorption of the bioreactor, photo-bleaching,</p><p>11and background noise. The continuously increasing number of amplified dsDNAs produces a</p><p>12positive or upper dynamical amplified signal. However, the denatured dsDNA at 65°C, the surface</p><p>13adsorption of DNA by the bioreactor, and photo-bleaching from the excitation light lead to a</p><p>14negative or downward dynamical change of the amplified signal, which usually causes the detection</p><p>15response of the nucleic acid amplification to be unstable and late1,2. Further, the background noise of</p><p>16the bioreactor material and surroundings produces a high original value for the dynamical amplified</p><p>17signal and low amplified efficiency or low ratio of end signal to start signal, which usually decreases</p><p>18sensitivity. Therefore, general nucleic acid amplification reactions in Eppendorf tubes and 96- or</p><p>19384-well microplates is usually performed in ≥25-μL volumes to increase the signal of the</p><p>1 4 1amplified dsDNA far more than the lost signal from the negative factors, as well as to obtain a</p><p>2relative steady exponential mode for the amplified signal and good consistency for repeated</p><p>3detection. Indeed, in these conditions, the differences from the denatured dsDNA at the high</p><p>4amplification temperature, the surface adsorption of the bioreactor, photo-bleaching, and background</p><p>5noise can be ignored. </p><p>6 When the reaction volume is reduced to <10 μL, the above negative effects are significant. If the</p><p>7lost fluorescence signal from the denatured dsDNA is Eu, the increasing fluorescence signal from the</p><p>8amplified dsDNA is Ea, the decreased fluorescence signal from surface adsorption is Es, the reduced</p><p>9fluorescence signal from photo-bleaching is Ep, and the background signal from the bioreactor</p><p>10material and surroundings is Eb, then the dynamic measured fluorescence signal of nucleic acid</p><p>11amplification E can be calculated with the following formula (1):</p><p>12 E = Eo + Ea + Eb - Es - Eu - Ep (1)</p><p>13where Eo is the original fluorescence signal of the mixtures, including the template DNA, sample,</p><p>14and reagents at 25°C. We report that the non-stick-coated metal micro-nanoliter fluidic chip and</p><p>15portable confocal detector decreased these possible negative effects, resulting in stable nucleic acid</p><p>16amplification, sensitive detection, and a rapid response in micro-nanoliter reaction volumes.</p><p>17 Figure S1 shows an obvious change in the nucleic acid amplification from the surface adsorption</p><p>18in uncoated and non-stick-coated metal micro-nanoliter fluidic bioreactors. In Figure S1 (a), the</p><p>19uncoated metal micro-nanoliter fluidic chip displays a larger difference both in the times at the</p><p>20second derivative inflexion of the exponential DNA amplification curves (~4.7 min) and the relative</p><p>21fluorescence intensity (~91.7%). In Figure S1 (b), when the metal micro-nanoliter fluidic bioreactor</p><p>22was coated with the silicone non-stick material, the surface of the bioreactor became smooth and</p><p>23inert to DNA molecules, effectively eliminating surface adsorption (Es), which makes the nucleic</p><p>24acid amplification stable with little difference in the times at the second derivative inflexion of the</p><p>25exponential DNA amplification curves (~0.5 min) and the relative fluorescence intensity (~11%). </p><p>1 5 1 Figure S2 displays our portable confocal detector, which can collect fluorescence near the optical</p><p>2diffraction limit and effectively limit the background noise (Fig. S5). The fluorescence from an</p><p>3object on the focal plane can be focused into a small spot and transmitted with >92% intensity to the</p><p>4detector behind a pinhole filter (PH) at the focal plane of the imaging lens set (L2). The fluorescence</p><p>5from other off-focus objects creates a large dispersive spot with a very low transmitted efficiency</p><p>6behind the PH (e.g., the transmitted efficiency at the off-focus position of 18 μm is only ~1.13%).</p><p>7The detection plane for the fluorescence of the bioreactor material and surrounding is farther than</p><p>8the off-focus position of 50 μm, and thus, the background noise (Eb) from the bioreactor material</p><p>9and surroundings is approximately 0 and can be ignored. </p><p>-t/T 10 The photo-bleaching from the excitation light is directly proportional to 1-e , where T is half of</p><p>11the attenuant period and t is the irradiation time with the excitation light3. In our portable confocal</p><p>12detector, the micro-nanoliter fluidic bioreactor was moved by a rotary scanning stage (RS), and</p><p>13every bioreactor cell in one measuring period of 30 s was irradiated for <10 ms. Therefore, the</p><p>14photo-bleaching from the excitation light (Ep) was approximately 0. High amplification efficiency</p><p>15and a negligible effect from denatured dsDNA can be obtained by optimizing the amplification</p><p>16conditions, including the temperature (65°C) and the addition of 0.5 mg/ml BSA and 6 mM MgS04,</p><p>17which renders the lost fluorescence signal from denatured dsDNA (Eu) stable as a constant. </p><p>18 After all of the effects from the surface adsorption of the bioreactor, the denatured dsDNA at the</p><p>19amplification temperature, the photo-bleaching from the excitation light, and the background from</p><p>20the bioreactor material and surroundings are limited, and equation (1) can is simplified as:</p><p>21 E = Ea + C (2)</p><p>22where C is a constant, C = Eo - Eu. Compared to equation (1), equation (2) indicates that the</p><p>23dynamic fluorescence signal E of the nucleic acid amplification is directly proportional to the</p><p>24increased fluorescence signal Ea of amplified dsDNAs and can immediately respond to amplified</p><p>25dsDNAs in real time. Thus, the sensitivity can be improved when the negative factors are eliminated.</p><p>1 6 1</p><p>2 3 4 Figure S2</p><p>5</p><p>6Methods </p><p>7Design of primers. Oligonucleotide primers were designed for the isothermal amplified assay according to the sequence of the</p><p>8Mycoplasma pneumoniae P1 gene from GenBank (Accession No. M18639). Six primers, including two loop primers (LF and</p><p>9LB), two outer primers (F3 and B3), and two inner primers (FIP and BIP), were designed to recognize eight distinct regions on</p><p>10the target sequence (Fig. S3). BIP consists of the complementary sequence of B1 and the sense sequence of B2, FIP is</p><p>11comprised of the complementary sequence of F1 and the sense sequence of F2. The primer sequences are listed in Figure S4.</p><p>12</p><p>1 7 1 2 3 Figure S3 4</p><p>5 6 7 Figure S4 8</p><p>9Portable real-time fluorescent confocal detector. To detect the fluorescent signal of DNA amplification in real time with</p><p>10high sensitivity and low background, a new portable confocal detector was developed as shown in Figure S5. In Figure S5, an</p><p>11objective set L1 with a focal length of 13 mm and numerical aperture of NA = 0.72 was designed, which consists of seven</p><p>12lenses, including two doublets, and uses only three glass materials, ZK7, ZF2, and ZK11. The excited light from a white LED</p><p>13was first collimated by an aspherical lens L1 and filtered by the band pass filter F1 with a central wavelength of 480 nm and</p><p>14bandwidth of 30 nm. Then, the excited light was focused by the objective set L2 to illuminate the bioreactor cells of the micro-</p><p>15nanoliter fluidic chip MC on the rotary scanning stage RS. The intensity of the excited light was adjusted to adapt to the</p><p>16different applications of the chip by the attenuator A1. A dichroic mirror D1 was used to reflect the fluorescence from the</p><p>17bioreactor cell and to penetrate the excited light from the LED. The fluorescence from Sybase Green inserting into the dsDNA</p><p>18in the bioreactor cell was excited by the LED and initially collected by the objective set L2, reflected by D1, and focused onto</p><p>1 8 1the detector PMT (HAMAMATSU, Japan) by the imaging lens set L3 with a focal length of 22 mm. A filter F2 with a 520-nm</p><p>2central wavelength and 40-nm bandwidth was used to penetrate the fluorescence and limit the excited light. The pinhole PH</p><p>3was used to filter the farraginous light from the environment and the off-focused fluorescence from the material of the micro-</p><p>4nanoliter fluidic chip. Finally, the fluorescence signal was transferred to a computer by an A/D processor. The temperature</p><p>5controller TCD was used to administer the heater HF with an accuracy of 0.1°C and speed of raising the temperature of 1°C/s</p><p>6by the temperature feedback of sensor S in the process of DNA isothermal amplification. A multiple-axis moving driver</p><p>7MAMD was used to supervise the rotary scanning stage RS with angle accuracy of 0.01°.</p><p>8When the micro-nanoliter fluidic chip was used, the inlet and outlet holes were first opened using an Eppendorf tip with light</p><p>9pressure. Then, the mixtures of the circular probes, reagents, and DNA samples were injected into the bioreactor cells of the</p><p>10micro-nanoliter fluidic chip from the inlet hole using the tip. Third, the inlet hole and the outlet hole were sealed with a thin PC</p><p>11film from ABI Corporation. Fourth, the thin PC film, exceeding the edge of the chip or covering the center fixed hole of the</p><p>12chip, was trimmed for detection. Fifth, the channel between any two adjacent bioreactor cells was obstructed by heating</p><p>13compression to keep all bioreactor cells independent of each other during the process of isothermal amplification. Finally, the</p><p>14micro-nanoliter fluidic chip was placed into the portable confocal detector (Fig. S5) for isothermal DNA amplification and</p><p>15gene-specific identification.</p><p>16</p><p>Figure S5 17</p><p>1 9 1References</p><p>21. Wang, M. et al. Accelerated Photobleaching of a Cyanine Dye in the Presence of a Ternary Target DNA, PNA</p><p>3 Probe, Dye Catalytic Complex: A Molecular Diagnostic. Anal. Chem. 81, 2043–2052 (2009).</p><p>42. Koek, M.M. et al. Metabolic Profiling of Ultrasmall Sample Volumes with GC/MS: From Microliter to Nanoliter</p><p>5 Samples. Anal. Chem. 82, 156–162 (2010).</p><p>63. Huang, G.L. et al. Fluorescence photobleaching properties for microarray chips. Chinese Journal of Luminescence.</p><p>7 Chinese Journal of Luminescence 27, 259-264 (2006). (in Chinese)</p><p>1 10 1Figure caption</p><p>2Figure S1 Comparison of DNA amplification in uncoated and non-stick-coated metal micro-</p><p>3nanoliter fluidic chips. (a) Isothermal DNA amplification in the uncoated metal micro-nanoliter</p><p>4fluidic chip, which displays obvious differences among the five parallel bioreactors. (b) Isothermal</p><p>5DNA amplification in the non-stick-coated metal micro-nanoliter fluidic chip, where the</p><p>6amplification in the five parallel bioreactors displays good consistency and the time difference at the</p><p>7second derivative inflexions of the exponential DNA amplification curves for the five bioreactors are</p><p>8within 0.5 min. (c) Normalizing processing of the isothermal DNA amplification curves in (b).</p><p>9</p><p>10Table S1 Comparison of the times at the inflexions of the second derivative of the exponential</p><p>11DNA amplification curves between our advanced metal micro-nanoliter fluidic bioreactors and</p><p>12in the general 25-μL Eppendorf tubes.</p><p>13</p><p>14Figure S2 Comparison of fluorescence collection on the focal plane to the off-focus position for</p><p>15the developed portable confocal detector. The horizontal axis corresponds to the radius of the</p><p>16optical spot from the center of the optical axis, and the vertical axis is the relative transmitted</p><p>17intensity. The fluorescent intensity on the focal plane can be collected with a high efficiency (92%)</p><p>18near the optical diffractive limit. There was a low transmitted efficiency of 3.46% at the off-focus</p><p>19position of 8 μm and lower transmitted efficiency of 1.13% at the off focus position of 18 μm.</p><p>20</p><p>21Figure S3 Diagram of the primers used. Constructions of the inner primers BIP and FIP are</p><p>22displayed. F1c and B1c are complementary sequences to F1 and B1, respectively.</p><p>1 11 1Figure S4 Partial nucleotide sequences of the P1 gene of M. pneumoniae and the primers used</p><p>2for amplification. Arrows indicate positions of specific primers. The left arrow indicates that a</p><p>3complementary sequence is used for the primer. The right arrow indicates that a sense sequence is</p><p>4used for the primer.</p><p>5Figure S5 Portable confocal detector. The white light LED had a power of 120 mW, aspherical</p><p>6lens L1 had a focal length of 20 mm, A1 is an attenuator, F1 and F2 are two band pass filters, D1 is a</p><p>7dichroic mirror, and L2 is an objective. MC is the microfluidic chip, S is the temperature sensor, RS</p><p>8is the rotary scanning stage, HF is the heater, L3 is an imaging lens set, PH is the pinhole, and PMT</p><p>9is a photo-electronic detector. A/D is a 16-bit analog-to-digital processor, TCD is the temperature</p><p>10controller, MAMD is a multiple-axis moving driver, and a computer was used to manage the process</p><p>11of isothermal DNA amplification and display the amplification curves in real time. </p><p>1 12</p>
Details
-
File Typepdf
-
Upload Time-
-
Content LanguagesEnglish
-
Upload UserAnonymous/Not logged-in
-
File Pages12 Page
-
File Size-