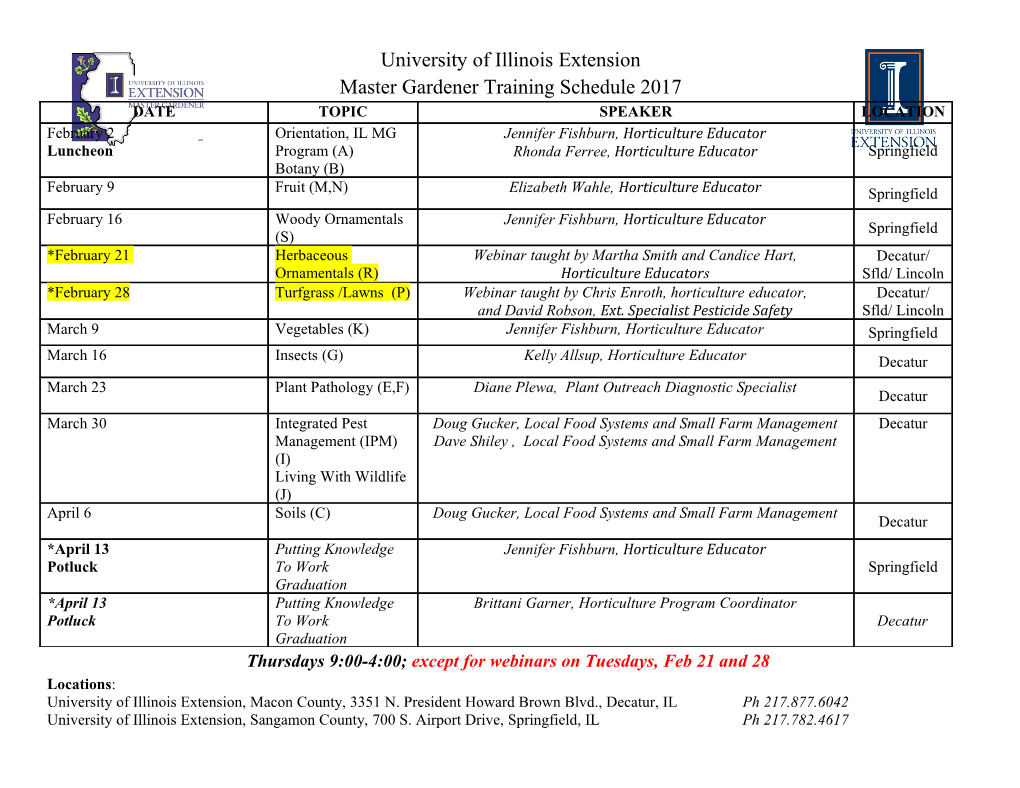
<p> ME2109 Developing a Trial Monitoring Strategy for pH in UK Marine Waters</p><p>A Report to Defra</p><p>Plymouth Marine Laboratory Prospect Place West Hoe Plymouth PL1 3DH</p><p>2 ME2109 Developing a Trial Monitoring Strategy for pH in UK Marine Waters</p><p>Contents page</p><p>List of contributors and project management……………………………………….3 Executive summary…………………………………………………………………4 List of abbreviations………………………………………………………………...6 Proposal for a Trial Monitoring Strategy for pH in UK Marine Waters………7 Introduction to reviews and proposal development…………………………………12 Section 1 A review of data sets in which pH has been measured directly or can be calculated from other carbonate system measurements...14 Section 2 A formal validation of existing model performance against data mined for this project………………………………………………22 Section 3 A review ranking the biogeochemical and physical processes likely to determine the acidity of seawater in different shelf regions……28 Section 4 A review of where our shelf seas are most vulnerable to pH changes…...33 Section 5 Development of an observational programme……………………………43 5a A critical review of existing and developing technologies for measuring the variability and change of pH………………………...43 5b Development of an observing plan……………………………………….65 5c Quality control……………………………………………………………70</p><p>Appendices</p><p>1. Original Proposal for ME2109……………………………………………………76 2. Strawman monitoring proposal for consultation with MARG……………………82 3. Outline summary of feedback from MARG………………………………………87 4. Written feedback from MARG……………………………………………………89 5. Factors limiting additional UK sampling capability for pH………………………93 6. Costings for a UK pH monitoring scheme as proposed…………………..……....95</p><p>3 ME2109 Contributing authors and project management</p><p>Plymouth Marine Laboratory: Prospect Place West Hoe Dr Carol Turley* Plymouth, PL1 3DH Dr Anthony Bale Mr Jerry Blackford telephone 01752 633100 Dr Nicholas Hardman-Mountford * [email protected] Ms Nancy Jones </p><p>National Oceanography Centre: </p><p>Dr David Hydes* University of Southampton Dr Boris Kelly-Gerreyn Waterfront Campus Dr Eric Achterberg European Way Dr Cedric Floquet Southampton, SO14 3ZH Dr Toby Tyrrell Dr Matt Mowlem telephone 023 8059 6547 switchboard 023 8059 6666 *[email protected] </p><p>University of East Anglia: School of Environmental Sciences Prof. Andrew Watson Norwich, NR4 7TJ Dr. Dorothea Bakker* Dr. Ute Schuster telephone 01603 592648 Switchboard 01603 592542 * [email protected] ______</p><p>ME2109 Defra Project Management</p><p>Project Officer </p><p>Dr Beth Greenaway</p><p>Management Group</p><p>Dr. Andrea Leedale Defra Dr. Paul Leonard Defra Dr. Andy Greaves Defra Dr. Steve Malcolm CEFAS Dr. Christoph Heinze CARBOOCEAN</p><p>Stakeholder Consultation </p><p>MARG</p><p>4 ME2109 Developing a Trial Monitoring Strategy for pH in UK Marine Waters</p><p>Executive Summary</p><p>Background</p><p>9 Concentrations of CO2 are increasing in the atmosphere. About 7x10 tonnes of CO2 enter the oceans each year. Calculations based upon the IPCC "Business as Usual" scenario show that by the mid-21st century the additional CO2 will cause the acid content of the ocean's surface to double (equivalent to a decrease* of 0.3 in the measured pH). The probability of this occurring is high. (* NB the pH scale decreases with increasing acidity) Baseline measurements of pH against which changes in UK waters can be judged are not available.</p><p>Existing Data There are almost no high quality, directly-measured data on pH for UK marine waters. pH can be calculated from other measurements. Good quality time series data is becoming available in some areas. Data for the Atlantic waters that are the source waters for UK shelf seas are available from 2002 onwards but funding for these time series is uncertain after 2007/8. </p><p>Modelling of pH (ERSEM-POLCOM-HALTAFALL model) It is unlikely that we can gain a comprehensive view of the spatial distribution of vulnerabilities from observational data alone. An alternative approach is to use existing data to validate deterministic simulation models as developed under ME2107 and use these to perform the desired analysis. Little data exists against which to validate models. The model captures the essential bulk properties of the carbonate system when compared with available data and can be considered as fit for purpose. Improved treatment of coastal processes: river loads, optical properties and parameterisation of alkalinity, are the key model refinements required. The present variability of pH in marine water due to seasonal temperature and biological activity, away from estuary plume effects, is about 0.4. The model simulations suggest that North Sea pH will be 0.2 pH units lower than pre- industrial by 2050.</p><p>Processes influencing the acidity of seawater in different shelf regions. The acidity of UK waters is determined by the biogeochemical cycle of growth and decay, exchange of waters with the Atlantic and of CO2 with the atmosphere.</p><p>5 Processes are intensified in estuary plumes (annual ranges greater than 1 pH unit are predicted).</p><p>Which areas of UK shelf seas are most vulnerable to pH changes? All UK waters will experience declining pH. The current state of knowledge on the impacts of ocean acidification is not yet sufficient to distinguish areas more or less vulnerable to changing shelf sea pH.</p><p>Existing capability and developing technology Detection of change of pH at the predicted annual rate of 0.003 (pH units) requires measurements at the limit of what is now possible. Presently pH must be calculated from measurements of other carbonate system parameters.</p><p> The UK has excellent capacity for measuring the partial pressure of CO2, (pCO2) in seawater on voluntary observing ships (VOS) and research vessels.</p><p> To calculate pH from pCO2, additional measurements of alkalinity and total CO2 have to be made on water samples in the laboratory using time consuming and therefore expensive methods. Analytical equipment using pH electrodes could be developed relatively quickly (6 months) to deliver measurements of the required precision, exploiting previous work for the EA. Development of a system based on colorimetry offers the possibility of systems which are robust enough for autonomous use (promising resolution of current undersampling in space and time), with levels of precision better than can be achieved by other means (~0.001pH). The cost of such development would be (~£200k) which must be balanced against savings in recurrent measurement cost. </p><p>Proposed work Requirements (i) best technologies and methods be used to get a precision and accuracy (±0.003 pH) equal to projected annual change (ii) annual range of pH in key hydrographic and biogeochemical process areas be defined (iii) regular data be collected appropriate for development and validation of prognostic models (iv) data be made rapidly available for use in models and indicators of eco-system change. TASK 1 Collection of data documenting the change in pH through two years in waters representing the range of conditions found in UK waters from eutrophic to waters of recent ocean origin. The data sets would include the necessary hydrodynamic and biogeochemical data needed for the better development of numerical models. This will be possible using existing observational efforts, which collect both data sufficiently frequently during the year for the annual changes to be precisely defined and where the ancillary data are already collected. To be based on POL, PML and, given sufficient resources, FRS Aberdeen Observatories and NOC (FerryBox) high frequency Portsmouth-Bilbao sampling.</p><p>6 TASK 2 Sustained monitoring is required of ocean waters as these are the source waters to the UK shelf. Rates of change being observed in the North Atlantic disagree with those currently predicted by numerical models. TASK 3 Data will need to be quality controlled and archived along with meta data that fully describes how the data were collected and how pH values were obtained and calibrated. Data will be available through BODC, MDIP and MERMAN. TASK 4 Investment should be put into instrument development so that measurement of pH can be done autonomously at high accuracy and precision. Both the pH electrode and the colorimetric approach should be followed. TASK 5 Modelling and statistical methods should be used to evaluate the baseline data collected in Tasks 1 & 2 so that an efficient long term monitoring programme can be established as part of the UK’s Clean Safe Seas Environmental Monitoring Programme (CSEMP).</p><p>List abbreviations that are not defined in the text</p><p>BODC British Oceanographic Data Centre FRS Fisheries Research Services MDIP Marine Data and Information Partnership NOC National Oceanography Centre PML Plymouth Marine Laboratory POL Proudman Oceanographic Laboratory TA Total Alkalinity UEA University of East Anglia VOS Voluntary Observing Ship</p><p>7 ME2109: Proposal for a trial Monitoring Strategy for pH in UK Marine Waters</p><p>The “baseline study” Purpose of study Requirements Resources constraints Resources Present Status Measurement summary & Standardisation Definition of tasks</p><p>The “baseline study” Before a long term monitoring programme can be established, high-resolution “baseline” data on the current levels of acidity (pH) are needed. The work would precisely determine both pH and its variability across the range of marine waters around the UK coast. The baseline study and the parallel refinement of protocols for sampling and analyses would enable a monitoring programme to be established which would provide statistically robust data for UK waters and adjacent ocean waters. Definition:“Baseline” measurements are carried out using the most appropriate available technology and recording both the data and methods used. Consequently any measurements made at a future time can be referenced back to these measurements and changed, or reassessed, with a known degree of certainty.</p><p>Purpose of study To define the range and variability in pH over a two year period in key water types that represent the range of conditions in UK waters and the ocean waters which are the source waters to UK shelf seas. To provide reference points for the detection of long term changes against a background of natural variation. To provide validation of the procedures that will be adopted in a future programme that provides long term monitoring of pH. To provide a data set which will assist the development and validation of numerical models that will enable better understanding of ocean acidification and likely future rates of change. Undertake statistical analysis of the data and review model data that will: o aid the design of a long term monitoring programme o develop appropriate indicators for describing change of pH in UK waters</p><p>Requirements Measurements of pH made to a precision and accuracy of ±0.003 pH units which is equal to the projected annual rate of change. Measurements made with sufficient frequency that the annual range of pH in UK waters is defined. Model results suggest this range may be as high as 1.0 pH unit in eutrophic waters. Measurements made with sufficient geographical spread that dynamic changes resulting from both hydrographic and biogeochemical processes can be observed.</p><p>8 Measurements made using the best available technologies and methodologies. Measurements documented in such away that derived values of pH can be recalculated if and when required by future improvements in the accuracy of our knowledge of chemical equilibria of the carbonate system in sea water. Measurements made of sufficient carbonate system parameters that the system is “over defined” and the results of directly measured and calculated pH can be compared to assess the accuracy and precision of current methodologies. Measurements made at defined times and regular locations so that they are appropriate for use in the development and validation of prognostic models. Measurements made of carbonate system parameters along side those of hydrodynamic and biogeochemical state so that both direct understanding and prognostic modelling expertise is improved by the availability of the new data sets. The methodologies and calculation used by different laboratories must be traceable between the laboratories. Best practice should be applied following the internationally agreed recommendations (currently CDIAC (DOE (1994) Handbook of methods for the analysis of the various parameters of the carbon dioxide system in sea water. (Version 2), A. G. Dickson & C. Goyet, eds. ORNL/CDIAC-74. which is under revision ).</p><p>Resource constraints We propose a baseline study which will be based on four existing UK sustained observing activities with an option of a fifth scheme at Aberdeen. These schemes provide the ability to collect samples at the required density without extending existing activities. The required complimentary data on hydrodynamics and biogeochemistry are already being made in these programmes. In each sampling scheme, except Aberdeen, some of the carbonate system measurements are already being made.</p><p>The programmes are in order of distance from shore: POL Coastal Observatory, Liverpool Bay (RV Prince Madog) FRS Stonehaven long-term sampling station, Aberdeen* Western Channel Observatory (L4, E1, RV Plymouth Quest transects) NOC Pride of Bilbao (VOS) data collection UEA Transatlantic (VOS) data collection</p><p>*Inclusion of this sampling scheme is geographically and scientifically attractive but at present has resource implications in that the samples generated would exceed the analytical capacity at NOC.</p><p>Resources POL Coastal Observatory, Liverpool Bay:- provides detailed coverage of a region of strong fresh water influence in a eutrophic system. A key question in this region is:- is the pH determined more by the respiration of organic matter supplied by the river or by the production of organic matter which is enhanced by the nutrient input in the rivers ? FRS Stonehaven long-term sampling station:- regular weekly sampling for nutrients, hydrographic information and phyto- and zooplankton data. Extra sampling is practical to obtain discrete sample for analysis of TA & TCO2 at NOC (given </p><p>9 additional capacity). There are no additional carbonate measurements undertaken at present. PML Western Channel Observatory:- the RV Plymouth Quest visits the L4 Station weekly and E1 monthly and is instrumented for pCO2 with the autonomous PML/Dartcom system - providing detailed information between Plymouth and the seasonally stratified waters at the E1 site, 22 miles offshore. The waters at the 2 sampling sites are stratified in summer enabling the influence of benthic processes on pH to be determined. NOC Pride of Bilbao (VOS) data collection:- provides high time resolution data allowing detailed recording of changes in pH in relation to biogeochemical activity in a range of environments from eutrophic harbours to the temperate Atlantic Ocean on the route between Portsmouth and Bilbao. It provides key information on the differences in behaviour between shelf seawaters and the ocean waters that are the source for those shelf sea waters. UEA Transatlantic (VOS) data collection:- provides data from the longest running UK data set of marine carbon data. It is critical that we can assess the variability in the ocean uptake of CO2 and the extent of the consequent year to year fluctuations in pH. </p><p>Present Status:</p><p>Carbonate system pCO2 is currently measured automatically and at high resolution at four of the five sites. Systems are presently operational on the MV Santa Maria, MV Pride of Bilbao, RV Prince Madog and RV Plymouth Quest. pH can now be measured to almost the degree of precision required for this work using a flowing liquid-junction, electrode-cell system (developed at PML for the EA) in an underway system to provide high resolution data . This system has a precision of typically ~0.005 pH and a quoted accuracy of 0.01 pH. However experience with this system in terms of maintaining that precision is lacking. This is currently being evaluated by NOC and a similar system will be tested at PML. TA and TCO2 can only be measured to the required degree of accuracy and precision on discrete samples. We propose to over-determine the system by collection and measurement of samples in each of the survey schemes except on the MV Santa Maria (i.e. POL, PML and PoB, and at Aberdeen, if funds allow). These are key measurements and an internationally-accepted, certified reference material will be used to control the accuracy of these analyses.</p><p>Other biogeochemical system determinands. By basing the work on 4 existing monitoring programmes we can support this work within an existing frame work that is collecting high quality data. Salinity data is available from all areas and calibration is based on well-established procedures and traced back to use of IAPSO standard sea water. Temperature data are commonly available but for carbonate system work data must be accurate and precise to better than 0.1oK. A protocol for the required traceability of temperature is being developed at NOC by Charlene Bargeron in consultation with Andrew Dickson as part of a current revision of DoE CDIAC (DOE (1994) Handbook of methods for the analysis of the various parameters of the carbon dioxide system in sea water. (Version 2), A. G. Dickson & C. Goyet, eds. ORNL/CDIAC-74.) Nutrients are currently measured throughout the year at POL, PML and PoB measured by NOC and PML and at Stonehaven measured by FRS at Aberdeen.</p><p>10 Measurement summary & standardisation All the systems will provide records of hydrographic conditions and changes in concentrations of nutrients tracking the inputs of land based influences, over turn of the water and the intensity of the annual production/respiration cycle in the different areas. All areas, except Aberdeen, will provide data from autonomous pCO2 systems and water samples will be collected for the determination of total alkalinity and total CO2 so that the carbonate system can be over-determined in each area.</p><p>POL Coastal Observatory, Liverpool Bay: </p><p> 8 times per year using the RV Prince Madog. Autonomous continuous pCO2 will be made on these survey cruises. </p><p> Surface waters samples for the determination of TCO2 and TA will be collected on each survey at each site (36) and sent to NOC for analysis along with nutrient samples collected at each station. (288 samples/year).</p><p>FRS, Aberdeen Stonehaven Station. Weekly sampling at 4 depths, 3km offshore and uninfluenced by river runoff. Parallel sampling for hydrographic variables, nutrients and biological data Samples will be collected at 4 depths (4 * 50 = 200 samples/year).</p><p>Western Channel Observatory (L4, E1, RV Plymouth Quest transects) Weekly section to L4 and monthly section to E1</p><p> Continuous pCO2 and potentially pH in due course (EA system) surface records Samples will be collected at 4 depths: (4*50 + 4*12 = 250 samples/year).</p><p>NOC Pride of Bilbao (VOS) data collection</p><p> Continuous observation of pCO2 330 days per year </p><p> 36 samples for TA and TCO2 collected on each of 8 manned crossings per year = 288 samples measured at NOC pH electrode system measurements made with flowing liquid junction capable of resolving pH to 0.002 will be implemented to run continuously along side the pCO2 system.</p><p>UEA Transatlantic (VOS) data collection</p><p> Continuous observation of pCO2 >120 days per year </p><p>Equipment All the analytical and sampling equipment used in this work will be traceable through the meta data provided alongside the reported data.</p><p>Methods The analytical methods used will follow current best practice as described in the DoE CDIAC Handbook.</p><p>11 Calculations When reporting data derived from calculations, data will be accompanied by statements of which carbonate system parameterisation have been used.</p><p>Definition of tasks:</p><p>TASK 1: Two year programme of sample collection to establish “baseline” condition in UK water types. (Lead NOC with PML) </p><p>Carryout a programme of sampling and measurement along side existing UK sampling programmes: POL Coastal Observatory, Liverpool Bay Western Channel Observatory (L4, E1) NOC Pride of Bilbao (VOS) data collection Optionally Stonehaven at FRS, Aberdeen</p><p>TASK 2: North Atlantic Data Base (UEA only)</p><p>Provide a data set that details the variability of the pH in the North Atlantic waters that are the source waters for UK shelf seas since the start of observations in 2002, and continue time series of UEA Transatlantic (VOS) data collection.</p><p>TASK 3: UK acid seas monitoring data archive (Lead BODC with partners)</p><p>Carryout the necessary formatting and quality control and assembly of meta data (detailing all the sampling and chemical analysis procedures used and subsequent QC and calculations applied to the data). Transfer this data to an archive available from BODC and accessible through MDIP.</p><p>TASK 4: Direct measurements of the pH of seawater (Lead PML with CEFAS) </p><p>Develop and validate a high precision electrode-based pH measuring device that will reduce the potential costs of “permanent” monitoring programme and enable autonomous use on Smart Buoys and in Ferry Boxes.</p><p>TASK 5: Design of a statistically robust long term programme for monitoring change in acidity of marine waters (Lead PML with CEFAS)</p><p>Contribute to developing protocols for observational and model data archiving. Conduct a formal model validation process on a yearly basis incorporating data gathered under the proposed programme. The resulting error statistics would be used to drive improvements in the model system (funded under other programs). The validated model output would be provided to partners to contribute to the fine tuning of the observational program in subsequent phases.</p><p>12 ME2109 Introduction to reviews and proposal development</p><p>Rationale: Atmospheric carbon dioxide concentrations have increased substantially over the last two hundred years through human activities, in particular due to the burning of fossil fuels. Projections are that CO2 emissions may increase substantially in the future. It is now widely accepted these increased CO2 emissions have driven and will drive future changes in the earth’s weather patterns and climate. Nearly half of this anthropogenic CO2 has been absorbed through the surface of our oceans and more will be absorbed in the future. Presently ocean uptake of CO2 is reducing the rate of increase of CO2 in the atmosphere and so slowing the rate of climate change. However, as CO2 dissolves in seawater the chemical equilibrium of the carbonate system in the sea is shifted. This is causing the oceans to become more acidic with the potential to adversely effect the functioning of marine ecosystems. The potential impacts of acidification in our oceans have been recognised but we have little evidence of what changes have occurred and are occurring in waters around the UK. The UK Government and other stakeholders need this knowledge to determine the implications of acidification of the UK waters.</p><p>Approach: To do, this Defra wishes to undertake a programme of measurements which will:- (i) establish the current status of UK waters with respect to their carbonate chemistry, and (ii) in combination with experiment work on organism, biodiversity and process response to higher CO2 and ecosystem modelling, has the potential to detect future changes and their impact on marine eco-systems, if and when they occur. The work described here will provide a framework for a future long term monitoring programme.</p><p>Main objectives: The initial phase of the work is an assessment of the available data sets and ecosystems vulnerable to change, together with a critical review of current and developing technology for both observations and the throughput of this data into the marine management cycle. The key outcome of this work will be a set of recommendations and a plan for a two year trial programme of monitoring. The design of this programme will be based on the extension of existing marine field work and, its integration into the developing UK Marine Monitoring and Assessment Strategy. </p><p>Benefits: This effective and costed programme of work will be integrated with currently operating and developing monitoring programmes. Best practice will be determined through a review of available measuring technologies, sampling procedures, data processing and data analysis alongside numerical modelling. This, in addition to experimental and modelling approaches, will reduce the uncertainty in assessing the vulnerability to UK marine ecosystems of acidification</p><p>Background information: NOC, PML and UEA have measurement systems that are now providing regular information on the exchange of CO2 between the atmosphere and the sea in UK waters. These are based on continuous observations using instruments installed on commercial and research ships and instrumented buoys which can make measurements autonomously and report the data ashore in real time. These and other organisation round the UK regularly collect water samples for chemical analysis, these programmes can be extended to collect samples for the measurement </p><p>13 of carbonate chemicals. The numerical ecosystem model ERSEM has reached a level of maturity where it can contribute to the extrapolation of information into the future and to areas where in-situ data has not been collected.</p><p>Marine Management</p><p>Information Needs Information Use</p><p>Monitoring Strategy Reporting</p><p>Network Design Data Analysis</p><p>Sample Collection Data Processing</p><p>Sample Measurement</p><p>Schematic outlining the proposed strategy</p><p>14 Section 1. A review of existing data sets in which pH has been measured directly or can be calculated from other carbonate system measurements. </p><p>Lead author: Ute Schuster, UEA</p><p>This review includes:- 1. A review of data available in the published literature and elsewhere and data that may become available at some time in the future from other sources such as the EU-FP6-project CARBOOCEAN. 2. Data sets under the ownership of project participants (and available to project partners) to validate existing model systems. The largest is that of underway pCO2 collected on the UEA trans-Atlantic (Portsmouth - Caribbean) Ship of Opportunity. This has been running continuously since April 2002 with earlier measurements in 1994 and 1995. Additionally there are data from the PML monitoring stations E1 and L4 in the English Channel, data collected by the CASIX systems on NERC research ships, and from NOC on the Portsmouth – Bilbao Ferry box line since September 2005. The best approach for estimating pH from data sets with a single inorganic carbon parameter (eg. pCO2) will be investigated. 3. A preliminary MCCIP report card will be produced on the basis of the existing data.</p><p>Data available from project partners</p><p>MV Santa Maria data, UEA, 2002 to 2006: SM_Defra_070326.dat</p><p>Relevant data (including one carbon parameter and salinity) are available from the July 2002 onwards, collected during CAVASSOO (Carbon Variability Studies by Ships of Opportunity, http://lgmacweb.env.uea.ac.uk/e072/welcome.htm, contract number EVK2-CT-2000-00088) and CarboOcean (Marine carbon sources and sinks assessment, http://www.carboocean.org/, contract number 511176-2), on board MV Santa Maria trading between Le Havre, France, or Portsmouth, UK, and the Caribbean.</p><p>PI: Ute Schuster and Andrew Watson, UEA</p><p>15 The data file has the following columns Column Parameter Unit Measured/calculated 1 Year yyyy GPS recording 2 Date Day since zero GPS recording 3 Time Decimal GPS recording 4 Decimal day of calculated year 5 Latitude [oN] GPS recording 6 Longitude [oE] GPS recording 7 SST [oC] Measured 8 SSS [psu] Measured</p><p>9 Sea surface pCO2 [μatm] Measured 10 Alkalinity [μeq/kg] Calculated Note A 11 pH Calculated Note B Note B 12 TCO2 [μmol/kg] Calculated Note B 13 fCO2 [μatm] Calculated </p><p>Note A: Alkalinity was calculated using SST and SSS, south of 30oN according to the equation given in [Lee, et al., 2006] for the (sub)tropics, and north of 30oN according to the equation given in [Lee, et al., 2006] for North Atlantic. Note B : pH, TCO2, and fCO2 were calculated using CO2sys_macro_PC.xls [Lewis and Wallace, 1998], using SST, SSS, pCO2, on a seawater pH scale with the dissociation constants by [Mehrbach, et al., 1973], refitted by [Dickson and Millero, 1987].</p><p>Individual data files of each voyage are available under the following file IDs (“SM” stands for “Santa Maria”, followed by start date of voyage as “yyyymmdd”).</p><p>P r i d e o f B i l b a o d a t a 2 0 0 5 a n d 2 0 0 6</p><p>P&O Pride of Bilbao, NOCS, 2005 and 2006, PoB_Defra_070326.dat</p><p>Data are available from September 2005 to July 2006, collected during the FerryBox project (http://www.ferrybox.org/, European Commission contract no: EVK2-CT-2002-00144), on board Pride of Bilbao sailing Portsmouth, UK, and Bilbao, Spain.</p><p>PI: David Hydes, NOCS</p><p>The data file has the following columns Column Parameter Unit Measured/calculated 1 Year 2 Date Day since zero 3 Time decimal 4 Decimal day of year 5 Latitude [oN] 6 Longitude [oE]</p><p>16 7 SST [oC] Measured 8 Salinity [psu] Measured Note C 9 pCO2 [μatm] Calculated 10 Alkalinity [μequ/kg] Measured 11 pH Calculated Note C</p><p>12 TCO2 [μmol/kg] Measured Note C 13 fCO2 [μatm] Calculated 14 Phosphate [μmol/kg] Measured 15 Silicate [μmol/kg] Measured</p><p>Note C : pH, pCO2, and fCO2 were calculated using CO2sys_macro_PC.xls [Lewis and Wallace, 1998], using SST, SSS, Total alkalinity, TCO2, phosphate, and silicate, on a seawater pH scale with the dissociation constants by [Mehrbach, et al., 1973], refitted by [Dickson and Millero, 1987].</p><p>MV Prince of Seas, 1994 to 1995, PoS_defra_070326.dat</p><p>Data are available from 1994 and 1995, collected during UK DoE contract PECD/7/ 12/143, sailing between the UK and the Caribbean.</p><p>PI: Nathalie Lefevre and Andrew Watson</p><p>The data file has the following columns Column Parameter Unit Measured/calculated 1 Year yyyy GPS recording 2 Date Day since zero GPS recording 3 Time Decimal GPS recording 4 Decimal day of Decimal calculated year 5 Latitude [oN] GPS recording 6 Longitude [oE] GPS recording 7 SST [oC] Measured</p><p>9 Sea surface pCO2 [μatm] Measured</p><p>17 PML/Dartcom systems (Carbon-Ops project)</p><p>PI: Nick Hardman-Mountford</p><p>Ship Cruise Dates Location Discovery AMT-17 Oct-Nov 2005 N & S Atlantic D313 (SOLAS DOGEE) Nov-Dec 2006 NE Atlantic D317 Mar-Apr 2007 N Atlantic D318 Apr-May 2007 N Atlantic Prince Madog MATSIS Apr 2006 Irish Sea Hollyhead-Dublin transect x2 Jul 2006 Irish Sea Autosub Jul 2006 Irish Sea MATSIS Sep 2006 Irish Sea POL Coastal Observatory (5 to date) Sep 2006-Apr 2007 Irish Sea James Clark Ross Immingham-Stanley Sep 2006 N & S Atlantic JR152/159 (Stanley, South Georgia) Oct 2006 Southern Ocean JR161 (Stanley, Signy, South Georgia) Nov 2006 Southern Ocean JR163 (Stanley, Rothera, Signy, South Georgia) Dec 2006-Jan 2007 Southern Ocean Stanley-Montevideo Jan 2007 SW Atlantic JR157 (Stanley, Rothera, Bellingshausen Sea) Jan/Feb 2007 Southern Ocean JR165/170 (Rothera, Bellinghausen Sea) Feb/Apr 2007 Southern Ocean JR168 (Scotia Sea, Weddell Sea) Apr/May 2007 Southern Ocean Plymouth Quest L4/E1 seasonal cycle Mar 2005-Sep 2006 W. English Channel L4/E1 weekly/monthly transect Apr 2007-Date W. English Channel James Cook None yet (awaiting TSG installation) Scheduled Apr 2007 N. Atlantic</p><p>18 Tamar estuary data</p><p>File number File name/date Number of stations Number of alkalinity 1 TA-14 SEP 83 62 42 2 TA - 16 NOV 83 60 44 3 TA- 26 JAN 84 44 29 4 TA - 30 MAR 84 70 37 5 TA- 15 MAY 84 55 30 6 TA - 13 JUN 84 37 18 7 TA – 20 JUL 84 53 29 8 TA - 23 JUL 84 48 23 9 TA - 25 JUL 84 51 21 10 TA - 27 JULY 84 50 20 11 TA - 29 JULY 84 43 19 12 TA- 31 JUL 84 48 20 13 TA - 03 AUG 84 40 20 14 TA - 05 AUG 84 28 16 15 TA - 07 SEP 84 45 18 Tamar estuary alkalinity data files:</p><p>Data collected between Plymouth Sound and the fresh water of the R. Tamar . PI: Tony Bale</p><p>Column headings within files:</p><p>Column Header Units 1 Distance Km from Gunnislake weir 2 Time local 3 Temperature Celsius 4 Salinity PSU 5 Oxygen saturation % 6 Suspended solids mg per litre 7 pH 8 alkalinity equivalents</p><p>These data were all collected between Plymouth Sound and the fresh water of the R. Tamar.</p><p>19 Additional data</p><p>Reference Time Parameters Location [Frankignoulle, 1985 pH Measured North Sea, Shetland Islands 1988] pCO2 measured alk measured [Kempe and 1985 and 1986 pH: Measured North Pegler, 1991] Alk: measured Sea, 3 TCO2: measured water pCO2: calculated masses: N. Atlantic (enters N Sea from the NW), Skagerrak (outflow of Baltic), German Bight (influenced by European rivers). </p><p>[Hoppema, 1991] 1987 TCO2: measured Eastern Alk: measured part of the pH: measured Southern SSS: measured North Sea pCO2: calculated Bight</p><p>[Frankignoulle, 1992 and 1993 pH: Measured et al., 1996] Alk: measured pCO2: calculated</p><p>English Channel and Southern Bight of the North Sea [Borges and 1995 and 1996 pH: Measured Belgian Frankignoulle, pCO2: measured/ and 1999] calculated southern Alk measured Dutch Oxygen: coasts measured</p><p>[Frankignoulle, 1992 to 1997 pH measured European estuaries et al., 1998] pCO2 calculated </p><p>20 before 1995, then measured [Frankignoulle 1993 to 1999 pH measured and Borges, pCO2 calculated 2001] before 1995, then measured</p><p>European continental shelf – Galician Sea, Bay of Biscay, Armorican Sea, Celtic Sea, English Channel, North Sea</p><p>[Bozec, et al., 2001 TCO2: measured 2005] Oxygen: measured Nutrients: measured Chl a: measured</p><p>North Sea </p><p>21 References</p><p>Borges, A. V., and M. Frankignoulle (1999), Daily and seasonal variations of the partial pressure of CO2 in surface seawater along Belgian and southern Dutch coastal areas, J. Mar. Syst., 19, 251-266. Bozec, Y., et al. (2005), The continental shelf pump for CO2 in the North Sea - evidence from summer observation, Marine Chemistry, 93, 131-147. Dickson, A. G., and F. J. Millero (1987), A comparison of the equilibrium constant for the dissolution of carbonic acid in seawater media, Deep-Sea Res., 34, 1733- 1743. Frankignoulle, M. (1988), Field measurements of air-sea CO2 exchange, Limnol Oceanogr., 33, 313-322. Frankignoulle, M., et al. (1998), Carbon dioxide emission from European estuaries, Science, 282, 434-436. Frankignoulle, M., and A. V. Borges (2001), European continental shelf as a significant sink for atmospheric carbon dioxide, Global Biogeochem. Cycl., 15, 569-576. Frankignoulle, M., et al. (1996), Distribution of surface seawater partial CO2 pressure in the English Channel and in the Southern Bight of the North Sea, Continental Shelf Research, 16, 381-395. Hoppema, J. M. J. (1991), The seasonal behavior of carbon dioxide and oxygen in the coastal North Sea along the Netherlands, Neth. J. Sea Res., 28, 167-179. Kempe, S., and K. Pegler (1991), Sinks and Sources of CO2 in Coastal Seas - the North Sea, Tellus, B43, 224-235. Lee, K., et al. (2006), Global relationships of total alkalinity with salinity and temperature in surface waters of the world's oceans, Geophysical Research Letters, 33, L19605, doi:19610.11029/12006GL027207. Lewis, E., and D. W. R. Wallace (1998), Program Development for CO2 System Calculations, 26 pp., Carbon Dioxide Information Analysis Center, Oak Ridge National Laboratory, US Department of Energy, Oak Ridge, Tennessee. Mehrbach, C., et al. (1973), Measurement of the apparent dissociation constants of carbonic acid in seawater at atmospheric pressure., Limnol Oceanogr., 18, 897- 907. Thomas H. et al. Rapid decline of the CO2 buffering capacity in the North Sea and implications for the North Atlantic Ocean, Global Biogeochem. Cycles, accepted (2007).</p><p>22 Section 2. A formal validation of existing model performance against data mined for this project </p><p>Authors: Jerry Blackford & Nancy Jones, PML</p><p>The numerical ecosystem model ERSEM-POLCOMS has reached a level of maturity where it can potentially contribute successfully to the extrapolation of information into the future and estimation of conditions in areas where in-situ data has not been collected. However the appropriate use of models depends on their accuracy and an understanding of why and when models and data deviate. Under ME2107 effort is underway to validate models; however this project will deliver some new data sets not currently available. This section reports a formal model validation exercise incorporating newly available data and quantifies model accuracy and error, as an input to Section 3.</p><p>1. Introduction</p><p>Because of the operational limitations of monitoring pH or related variables and the lack of scientific focus on pH prior to our current concerns about high CO2, it is unlikely that we can gain a comprehensive or even synoptic view of the spatial distribution of vulnerabilities from observational data alone. An alternative approach is to use existing data to validate deterministic simulation models as developed under ME2107 and use these to perform the desired analysis. </p><p>Under ME2107 we have created a UK modelling capacity for exploring the effects of high </p><p>CO2 (including lowered pH) on the marine ecosystem of UK shelf waters and made an initial exploration of marine ecosystem response to elevated CO2. Blackford & Gilbert, (2007) assesses the annual pH range, its drivers and future predictions for the southern part of the North Sea (below 56°N). Work has now extended the model domain to the whole of the UK shelf waters. </p><p>The numerical ecosystem model used, (ERSEM-POLCOMS) has reached a level of maturity where it can potentially contribute successfully to the extrapolation of information into the future and estimation of conditions in areas where in-situ data has not been collected. However the appropriate use of models depends on their accuracy and an understanding of why and when models and data deviate.</p><p>2. Model System Synopsis</p><p>The model system is a coupling involving three well established model codes, covering the carbonate system (HALTAFALL; Ingri et al, 1967), the marine ecosystem (ERSEM; Baretta, 1995; Blackford, 2004) and POLCOMS (Holt and James, 2001) giving a 3D hydrodynamic system. All of these model codes have been used previously in combination (HALTAFALL and ERSEM (Blackford and Burkill, 2002); ERSEM and POLCOMS (for example Allen et al, 2001; Holt et al 2005). </p><p>HALTAFALL provides an iterative method to determine chemical speciation, parameterised by two of total inorganic carbon (a state variable in the ERSEM ecosystem model), total alkalinity (TA), pCO2 or pH allowing calculation of the other two variables. We use two </p><p>23 regime dependant relationships to derive TA from salinity. For salinity > 34.65 we use the relationship reported by Bellerby et al (2005) for North Atlantic waters, (TA = 66.96∙S – 36.803); for salinity < 34.65 we approximate from Borges & Frankignoulle (1999) giving TA = 3887.0 – 46.25∙S. We use the sea water pH scale, with coefficients according to Weiss (1974), Dickson and Millero (1987), Hansson (1973) and Millero (1979). Air-Sea exchange of CO2 is calculated using the parameterisation of Nightingale et al (2000) acting on the derived partial pressure of CO2 in the water and the parameterised atmospheric concentration.</p><p>The European Regional Seas Ecosystem Model (ERSEM) is a complex plankton functional type (PFT) model developed in the context of the North Sea but now finding wider application (Baretta et al, 1995; Blackford et al, 2004). The POLCOMS hydrodynamic model is a three dimensional baroclinic circulation model in this case set up for the UK shelf Seas, taking boundary conditions from wider area versions of the same model. It is described in detail in Holt and James (2001). Dissolved inorganic carbon (DIC) concentrations for the main regional rivers are taken from Pätsch & Lenhart (2004). For rivers with no specific data we use the budget calculations in Thomas et al (2005) to derive a DIC load. For the future scenarios we assume that riverine DIC is in equilibrium with the prescribed atmospheric conditions and scaled accordingly. Riverine nutrient and flow rates are taken from various sources and are assumed not to change in the future scenarios. We prescribe atmospheric CO2 levels according to IPCC (2001) estimates, omitting the small seasonal signal for simplicity The ERSEM-POLCOMS model demonstrates some skill in reproducing regional observations (Holt et al., 2005, Allen et al in press, Lewis et al., in press). The carbonate-ERSEM model is initially validated in Blackford & Gilbert (2007).</p><p>3. Data Availability</p><p>For this project the model output has been compared against three distinct data sets:</p><p>1. Publicly available pH data from the Dutch ‘Waterbase’ database, maintained by Dutch National Institute for Coastal and Marine Management (RIKZ) and the Dutch Institute for Inland Water Management and Waste Water treatment (RIZA) http://www.waterbase.nl/, covering the southern North Sea 2. pCO2 data from the CAVASOO program supplied courtesy of Prof Andy Watson (UEA), relating to the English Channel. 3. Alkalinity and dissolved organic carbon data supplied by Dr David Hydes from the NOC Ferrybox program, also relating to the English Channel.</p><p>4. Results</p><p>4.1 A note on general model validation Detailed attempts to validate the ERSEM-POLCOMS North Sea simulations against a range of variables show a generally good representation of the physical environment, skill in resolving seasonal dynamics but a limited ability to resolve daily variability in harsh like-for- like comparisons with data (Holt et al., 2005; Allen et al., 2005). In stratified and off shore regions the model generally reproduces the correct partitioning of biomass between functional units but tends to under-estimate the thermocline depth during summer. In coastal environments the model does not produce completely the observed draw down in nutrients during the spring bloom, although chlorophyll levels are broadly correct. It is assumed that the complex optical properties of the strongly case II waters are not yet well represented in the model. Despite some specific drawbacks the ERSEM-POLCOMS North Sea models are generally considered fit-for-purpose and cited as the best validated of all regional modelling attempts (Moll & Radach, 2003)</p><p>24 Figure 2.1. Model (blue line) comparison with Waterbase data (points and error bars); the y- axis on each plot is pH. Each panel represents a distance from the Dutch coast towards the Dogger Bank (see Blackford and Gilbert, 2007).</p><p>25 4.2 Validation against waterbase data. Figure 2.1 presents a seasonal validation of modelled pH against data available from the Dutch Waterbase database (www.waterbase.nl) for a transect stretching from the Dutch coast to the Dogger bank. Because of the high variation, especially in peak timing, between years in the data and because ERSEM’s skill lies in the seasonal rather than short term prediction, we have calculated monthly mean, maximum and minimum pH values for the period 1997-2004. The model reproduces the mean of the data, the spatial trend and the range of the seasonal signal, although the timing of the main productivity signal in coastal regions is incorrect. This relates to the poor representation of case II water optical properties, rather than a misrepresentation of the physiological processes. It is worth noting that there is a considerable downward trend in pH as recorded in Waterbase, over the period 1997-2004 of about 0.02 pH -1 units.y , about 6 times greater than predicted from increases in atmospheric CO2 over this period. In our analysis we have de-trended the data around the year 2000 mean, consistent with our atmospheric CO2 parameterisation. </p><p>4.3 Validation against Cavasoo data</p><p>Figure 2.2 shows the comparison of model output with ship of opportunity pCO2 data mined under the CAVASOO project. In this region – the English Channel – the model is producing more seasonal variability than shown in the data but well represents the mean condition. </p><p>Essentially the model over estimates winter levels of pCO2, this could be due to excessive respiration in the modelled biology, although a range of factors could be contributing. Without additional data describing concurrent biochemistry it is difficult to be conclusive. A longitudinally resolved analysis confirms the generally high variability in the model and in particular identifies a tendency to excessive pCO2 at around 6° west. This probably derives from over estimation of frontal structures in the region causing excessive production. The model does reproduce the correct mean and longitudinal trend.</p><p>Figure 2.2a. Comparison of model output with CAVASOO data, seasonally resolved. Blue line represents the model mean, the grey area, model variability.</p><p>26 600 observations model 500 w 2 400 O C p 300</p><p>200 -12 -10 -8 -6 -4 -2 0 longitude Figure 2.2b. Comparison of model output with CAVASOO data longitudinally resolved </p><p>4.4 Validation against Ferrybox data</p><p>Although the data is sparse (Fig 2.3) the model is in general agreement with the observations.</p><p>Figure 2.3. Model comparison (shaded) with Ferrybox DIC data (circles and range bars).</p><p>4.5 Summary These initial studies identify the improved treatment of coastal processes: river loads, optical properties and TA parameterisation, as the key model refinements required. However it is clear that the model captures the essential bulk properties of the carbonate system when compared with available data and can be considered as fit for purpose. </p><p>27 References</p><p>Allen, J.I., Blackford, J.C., Ashworth, M.I., Proctor, R., Holt, J.T., Siddorn, J.R., 2001. A highly spatially resolved ecosystem model for the north-west European continental shelf. Sarsia. 86, 423-440. Baretta, J.W., Ebenhöh W. and Ruardij P., 1995. The European regional Seas Ecosystem Model, a complex marine ecosystem model. Netherlands Journal of Sea Research 33, 233- 246 Bellerby R.G.J., Olsen A., Furevik T. and Anderson L.A., 2005, Response of the surface ocean CO2 system in the Nordic Seas and North Atlantic to climate change. In: Climate Variability in the Nordic Seas, H. Drange, T.M. Dokken, T. Furevik, R. Gerdes, and W. Berger, Eds., Geophysical Monograph Series, AGU, 189-198 Blackford, J.C., Burkill, P.H., 2002. Planktonic community structure and carbon cycling in the Arabian Sea as a result of monsoonal forcing: The application of a generic model. Journal of Marine Systems, 36/3-4, 239-267. Blackford, J.C., Allen, J.I., Gilbert, F.G., 2004. Ecosystem dynamics at six contrasting sites: a generic modelling study. Journal of Marine Systems. 52, 191-215. </p><p>Blackford, J.C., Gilbert, F.J., 2007. pH variability and CO2 induced acidification in the North Sea. Journal of Marine Systems. 64, 229-241 Borges, A. V., Frankignoulle, M., 1999. Daily and seasonal variations of the partial pressure of CO2 in surface seawater along Belgian and southern Dutch coastal areas. Journal of Marine Systems, 19, 251-266. Dickson, A. G., Millero, F. J., 1987. A comparison of the equilibrium constants for the dissociation of carbonic acid in seawater media. Deep Sea Research 34, 1733-1743. Hansson, I. 1973. A new set of acidity constants for carbonic acid and boric acid in sea water. Deep Sea Research 20, 461-478. Holt, J.T., James, I.D., 2001. An s-coordinate model of the North West European Continental Shelf. Part 1 Model description and density structure. J. Geophys. Res. 106(C7): 14015- 14034. Holt, J.T., Allen, J.I., Proctor, R., Gilbert, F.G., 2005. Error quantification of a high resolution coupled hydrodynamic-ecosystem coastal ocean model: part 1 Model overview and hydrodynamics. J. Mar. Sys. 57,167-188. Ingri, N., Kakolowicz, W., Sillén, L.G., Warnqvist, B., 1967. High-speed computers as a supplement to graphical methods-V. HALTAFALL, a general program for calculating the composition of equilibrium mixtures. Talanta, 14, 1261. IPCC, 2001: Climate Change 2001: The Scientific Basis. Contribution of Working Group I to the Third Assessment Report of the Intergovernmental Panel on Climate Change [Houghton, J.T.,Y. Ding, D.J. Griggs, M. Noguer, P.J. van der Linden, X. Dai, K. Maskell, and C.A. Johnson (eds.)]. Cambridge University Press, Cambridge, United Kingdom and New York, NY, USA, 881pp. Millero, F. J. 1979. The thermodynamics of the carbonate system in seawater. Geochim. Cosmochim. Acta 43, 1651-1661. Nightingale, P. D., Malin, G., Law, C. S., Watson, A. J., Liss, P. S., Liddicoat, M. I., Boutin, J., Upstill-Goddard, R. C., 2000. In situ evaluation of air-sea gas exchange parameterizations using novel conservative and volatile tracers. Global Biogeochemical Cycles 14, 373-388. Pätsch, J., Lenhart, H. J., 2004. Daily loads of nutrients, total alkalinity, dissolved inorganic carbon and dissolved organic carbon of the European continental rivers for the years 1977- 2002. Berichte aus dem Zentrum für Meeres- und Klimaforschung, Reihe B: Ozeanographie. Thomas, H., Bozec, Y., de Baar, H. J. W., Elkalay, K., Frankignoulle, M., Schiettecatte, L.- S., Kattner, G., Borges, A. V., 2005. The carbon budget of the North Sea. Biogeosciences 2, 87-96. Weiss, R. F., 1974. Carbon dioxide in water and seawater: The solubility of a non-ideal gas. Marine Chemistry 2, 203-215, 1974.</p><p>28 Section 3. A review ranking the biogeochemical and physical processes likely to determine the acidity of seawater in different shelf regions.</p><p>Lead author: Carol Turley, PML</p><p>At a particular location the acidity of seawater will vary through the year because:- (i) seasonal changes in sea surface temperature and mixing affect surface water pH, (ii) processes of biological growth and decay influence CO2 in the water, (iii) of external inputs such as rain and rivers, and (iv) the acidity of the sea water is also determined by reaction with mineral phases. Based on the existing literature, a ranking has been made of the processes that are most likely to determine the acidity in different regions of the UK marine environment at different times of year. These will be compared with results from the existing ERSEM model systems.</p><p>1. Alkalinity (TA) and Dissolved Inorganic Carbon (DIC): Ocean pH is controlled by alkalinity and DIC and can be calculated from these two parameters. Internal variations in DIC and alkalinity are controlled by the ratio between photosynthesis: respiration (P:R) and between CaCO3 dissolution: CaCO3 precipitation. </p><p>2. Atmospheric CO2 concentration: Increasing CO2 emissions through the burning of fossil fuels and cement manufacturing have increased atmospheric CO2 concentrations from 280 ppm in 1800 to 380 ppm at the present day. This is set to continue to rise if we continue our fossil fuel usage in the same way (business as usual) with end of century atm CO2 of up to 1000 ppm predicted (IPCC 2007). However, there are calls for stabilization at 550 ppm (Stern, 2007) and lower. Atm CO2 is the major driver for surface ocean CO2 and TCO2 enrichment and therefore surface ocean pH decline.</p><p>3. Ocean uptake: The medium term (decades and centuries) sink for the atm CO2 is the surface of the worlds oceans. Already c. 50% of the CO2 produced (500 Gtons CO2) over the last 200yrs has been taken up by the oceans (Sabine et al. 2004) through air-sea gas exchange and this has resulted in a decline in pH of 0.1 unit. Predictions indicate that the global ocean pH will decline by a further 0.3-0.4 by 2100 and by 0.6 by 2300 in the business as usual scenario (Calderia & Wickett, 2003). Should changes to air-sea gas exchange (e.g. due to changes to wind and the balance between photosynthesis and respiration (P:R)) occur then there could be important small temporal scale variations (hours to weeks). However, this is unlikely to be important on larger annual time scales.</p><p>4. Primary production and respiration: There is a huge natural annual flux of -1 CO2 between the ocean and the atmosphere of almost 90 Gt C yr that, pre- 1800, was believed to be almost in balance. There was probably a net flux from ocean to atmosphere of about 0.6 Gt C yr-1 that balanced the supply of dissolved inorganic carbon to the oceans via rivers (Sarmiento and Sundquist, 1992). This huge influx and efflux is due to a combination of marine productivity (the biological pump) and ocean circulation (the solubility pump). Phytoplankton growth reduces dissolved inorganic carbon (DIC) levels in </p><p>29 surface seawater causing an undersaturation of dissolved CO2 and hence CO2 is transferred from the air to the ocean. The re-equilibration time for CO2 is slow due to the buffering of CO2 in seawater. Respiration processes release CO2, although some organic and inorganic carbon is transferred to the deeper oceans via particle sinking. This process is known as the biological pump. Primary production using photosynthesis results in the removal of CO2 from the seawater and an increase in pH. Respiration, on the other hand, results in the production of CO2 and a decrease in pH. The greater the productivity of the waters the greater the variation in pH due to P:R. so this ratio is important in UK shelf waters. Both of these are very important controls of the seasonal variability in pH but are not likely to impact longer time scales unless one of them changes. Almost all of the anthropogenic CO2 is thought to be taken up by the solubility pump (IPCC, 2001), as CO2 availability does not normally limit biological productivity in the world’s oceans. Indeed, a recent modelling study has indicated that including present biology in oceanic global circulation models increases the oceanic sink of anthropogenic CO2 by only 4.9% (Orr et al., 2001). </p><p>5. CaCO3 dissolution and CaCO3 precipitation: Calcium carbonate is usually found in the environment either as calcite or aragonite both of which are abundant in organisms. However, aragonite is more soluble than calcite. Calcium carbonate dissolves in seawater as shown below:</p><p>2+ 2- CaCO3 = Ca + CO3 1</p><p>Carbonate ions react with carbon dioxide as follows:</p><p>2- - CO2 + CO3 + H20 = 2HCO3 2</p><p>Indeed this reaction represents a useful summary of what happens when anthropogenic carbon dioxide dissolves in seawater. The net effect is removal of carbonate ions and production of bicarbonate ions and a lowering in pH. This in turn will encourage the dissolution of more calcium carbonate. </p><p>The medium to long-term sink for anthropogenic CO2 is dilution in the oceans and reaction with carbonate sediments with the long-term (1000-10,000 yrs) controls on alkalinity and DIC being the weathering (sources) and burial (sinks) of silicate and carbonate rocks. By 2300 about 70-80% of the anthropogenic CO2 will be taken up by the oceans, reducing pH by c. 0.7units, as the oceans turnover the CO2 will react with the deep ocean sediments and this will buffer ocean pH. This process will take several thousand years (Archer et al., 1997). Over tens of thousands of years a further buffering will take place with igneous rocks so that ocean pH will return to around 8.1 and the effect of the anthropogenic CO2 effectively removed. </p><p>Combining equations 1 and 2 gives:</p><p>2+ - CaCO3 + CO2 + H2O = Ca + 2HCO3 3</p><p>30 The contribution and impact of such buffering in shallow shelf seas is unknown as are the impacts of erosion and resuspension due to storms, bottom currents, ice disturbance and anthropogenic activities such as bottom trawling and aggregate extraction. However, these are only likely to have significant effects on pH at local and short term time scales. </p><p>A common misconception in biogeochemistry is that formation of calcite removes carbon dioxide. As can clearly be seen above, formation of calcite (the reverse of equation 3) actually produces CO2. Calcification produces CO2 but CaCO3 shells and liths can act as ballast for the long term removal of C from the surface oceans (biological pump). </p><p>6. Seawater temperature and warming seas: Colder water has greater gas carrying capacity and can therefore hold more CO2 than warmer water. So this can have an impact on a seasonal basis. The surface oceans are warming as well as becoming more acidic (IPCC, 2007). However, a recent paper indicated that future changes in ocean acidification caused by atmospheric CO2 emissions are largely independent of the amounts of climate change (Cao et al. 2007) with a climate sensitivity of 2.5oC the reduction in projected global mean surface pH is about 0.48 unit rather than 0.47 unit with no consideration of climate change.</p><p>7. Riverine input: Freshwater input from rivers and estuaries is a direct input of DIC and Alk as well as carbonic acid and can have a significant impact on pH in estuaries and local coastal waters where there are significant river flows to the sea (e.g. the Southern North Sea, Eastern Irish Sea). These waters will experience additional pH variability.</p><p>8. Ocean circulation and upwelling of deep water: The controls of alkalinity and DIC of deep ocean waters are respiration of organic matter and dissolution of CaCO3. Thus deep water is rich in CO2 and when this upwells carries CO2 to surface waters and therefore reduces pH. Ocean circulation also results in air-sea exchange of CO2 as the solubility of CO2 is temperature dependent. </p><p>9. Nutrients: Nutrient variation can have a small direct impact on pH variation but this is likely to be seasonal and its significance restricted to areas of high nutrient input such as estuaries.</p><p>10. Other anthropogenic gas emissions to the atmosphere: Fossil-fuel use and agriculture are dramatically changing the global nitrogen and sulphur budgets, through the release of large fluxes of nitrogen oxides, ammonia and sulphur dioxide to the atmosphere. Because of the relatively short life-time (days to about a week) of reactive nitrogen and sulphur species in the atmosphere, the majority of the acid deposition occurs on land, in the coastal ocean and in the open-ocean downwind of the primary source regions. The alterations in surface water chemistry from anthropogenic nitrogen and sulphur deposition are a few percent of the acidification and DIC increases due to the oceanic uptake of anthropogenic CO2. However, the impacts are more substantial in coastal waters, where the ecosystem responses to ocean acidification could have the most severe implications for mankind (Doney et al., submitted).</p><p>31 11. Rainfall: Rainfall direct to water will have very short term effects on the pH of the sea that directly interfaces with the air but this will be rapidly diluted through mixing and is likely to be insignificant on temporal and spatial scales.</p><p>12. Vents and seeps: CO2 vents and seeps can impact ocean pH in local waters where seepage is significant (e.g. Aegean). Although methane seeps occur in UK shelf waters there are no known CO2 seeps so this is thought to be insignificant in UK waters.</p><p>13. Hypoxic/anoxic waters: waters with low oxygen content experience extreme fluctuations in pH. Hypoxic or “dead zones” are increasing globally especially in environments with restricted exchange and high nutrient or organic input such as enclosed seas (e.g. Baltic Sea, Gulf of Mexico). Increased thermal stratification due to increased SST may result in their further extension. Some areas of the southern North Sea are prone to reduced O2 (Wadden Sea). </p><p>14. Methane hydrate releases: Increasing global temperatures may result in the release of methane from melting tundra and sediment bound methane hydrates. As well as being a strong greenhouse gas, methane is oxidised in the atmosphere resulting in further increases to atmospheric CO2 concentrations. It is thought that the rapid release of methane hydrates 55 My ago may have resulted in the ocean acidification event that caused the extinction of a large proportion of benthic calcifiers (Zachos, 2005).</p><p>15. Sediments: Organic-rich sediments experience extreme fluctuations in pH of an order of 2 pH units – this is due to respiration of microbes as they decompose organic matter. This has a significant influence on the distribution of organisms and processes that occur at different depths within the sediment profile. </p><p>References:</p><p>Archer, D., H. Kheshgi, and E. Maier-Reimer (1997), Multiple timescales for neutralization of fossil fuel CO2, Geophys. Res. Lett. 24, 405– 408. Archer, D., H. Kheshgi, and E. Maier-Reimer (1998), Dynamics of fossil fuel neutralization by marine CaCO3, Global Biogeochem. Cycles 12, 259– 276. Caldeira, K. and Wickett, M.E., 2003. Anthropogenic carbon and ocean pH. Nature 425, 365-365. Cao, L., K. Caldeira, and A. K. Jain (2007), Effects of carbon dioxide and climate change on ocean acidification and carbonate mineral saturation, Geophys. Res. Lett. 34, L05607, doi:10.1029/2006GL028605 Doney, S.C., N. Mahowald, I. Lima, R.A. Feely, F.T. Mackenzie, J.-F. Lamarque, and P.J. Rasch, The impact of anthropogenic atmospheric nitrogen and sulphur deposition on ocean acidification and the inorganic carbon system, Prod. Nat. Acad. Sci. USA, submitted. IPCC (2007) Fischlin A., Midgley G.F., Price J., Leemans R., Gopal B., Turley C., Rounsevell M., Dube P., Tarazona J., Velichko A. Intergovernmental Panel for </p><p>32 Climate Change Fourth Assessment Report, Working Group II, Chapter 4 – Ecosystems, Their Properties, Goods, and Services. Sabine, C.L. and 14 others (2004). The oceanic sink for anthropogenic CO2. Science 305, 367-371. Sarmiento, J.L. and Sundquist, E.T. (1992) Revised budget for the uptake of anthropogenic carbon-dioxide. Nature 356, 589-953. Stern, N.H. (2007). The economics of climate change: the Stern review. xix, 692p., Cambridge: Cambridge University Press, Zachos, J.C., Rohl, U., Schellenberg, S.A., Sluijs A, Hodell, D.A., Kelly, D.C., Thomas, E., Nicolo, M., Raffi, I., Lourens, L.J., McCarren, H. and Kroon, D. (2005) Rapid acidification of the ocean during the Paleocene-Eocene thermal maximum. Science 308, 1611-1615. </p><p>33 Section 4 . A review of where our shelf seas are most vulnerable to pH changes. </p><p>Lead Author: Carol Turley </p><p>We will use modelling and laboratory results from ME2107, current literature and the output from task 3 to estimate the vulnerability of UK Shelf Seas to acidification. In addition to the work planned under ME2107 we will:-</p><p>1. Compare, for each part of the model domain, the predicted in-situ pH range for various future atmospheric CO2 concentrations.</p><p>2. Quantify the distribution of predicted changes relative to natural seasonal variability in UK waters.</p><p>3. Given the known sensitivities of specific biogeochemical processes to pH changes we will briefly and qualitatively assess the potential disruption to the shelf ecosystem.</p><p>Introduction</p><p>Theoretically, identification of ‘hotspots’ of increased sensitivity to ocean acidification in UK waters could be beneficial in planning a monitoring programme for ocean pH. This is because the severity of consequences of ocean acidification may vary geographically around the UK and may depend on two key factors: (1) the inherent severity of the pH and associated carbon chemistry changes in each region and (2) the number of potentially susceptible organisms inhabiting each region. As an example of the second factor, if a particular area of sea were to be largely barren, then consequences of acidification are not likely to be important. We discuss here both the chemical differences and also the distributions of populations of potentially vulnerable organisms.</p><p>There is a distinct shortage of direct pH measurements in UK shelf Sea regions (see Section 1). In addition we have little knowledge of how pH and/or the CaCO3 saturation state (Ω) vary in near-coastal waters around the coastline of the UK. We therefore cannot say whether there are differences between the Severn Estuary, the Humber Estuary and the Moray Firth, for example, or any such detailed comparisons. Available data sets are summarised in WP1. </p><p>Modelled seasonal and depth variation of pH in the North Sea A more detailed examination of the biologically mediated CO2 fluxes over a seasonal cycle sheds light on the natural controls of pH variability in the context of a stratified water column (Figure 4.1). </p><p>34 8.18 8.16 -20 Primary Production 8.14 8.12 8.1 8.08 -40 8.06 8.04 8.02 -60 8 Modelled pH Benthic 7.98 Respiration 7.96 -80 7.94 0 30 61 91 122 152 183 213 243 274 304 335</p><p>160 140 -20 120 100 -40 80 60 40 -60 20 Pelagic Production - Respiration 0 -80 -20 0 30 61 91 122 152 183 213 243 274 304 335</p><p>150 Benthic Respiration 100</p><p>50</p><p>0 0 30 61 91 122 152 182 213 243 274 304 335 365</p><p>Figure 4.1. Modelled in-situ temporal and depth variability of pH and interactions with physical and biological processes over a calendar year (x-axis = days). Pelagic production units are productivity minus community respiration in mg C m-3 and -3 benthic respiration is outgassing of CO2 from the benthos also in mg C m . </p><p>Two broad features, in terms of pH, are apparent. Firstly a pH maximum (up to 0.15 units above background levels), tracks the production maxima from the surface spring bloom and continuing along the deep chlorophyll layer throughout summer. This coincides with the distribution of net positive pelagic community production and CO2 uptake. Secondly there is a pronounced pH minima (as much as 0.15 pH units below background levels) associated with the deeper waters under the thermocline that build up during the stratified period. Here benthic respiration and the subsequent diffusion of CO2 into the pelagic are causing elevated CO2 levels that are trapped in the stratified system. The flux of CO2 across the air-sea interface (not shown) is a net uptake during April and May, coincident with high primary production. During the summer months the out-gassing is driven by the net community respiration in the surface stratified layer. This reduces with the weakening of the thermocline and resultant increase in surface production during the autumn. A strong out-gassing signal is seen late in the year as the system finally overturns and the benthic CO2 is exposed to the atmosphere.</p><p>Model predictions of present and future pH variability Predictive model studies (Blackford & Gilbert, 2007) indicate that in-situ pH ranges approaching and sometimes exceeding 1 pH unit are associated only with the major </p><p>35 river plumes (Figure 4.2), consistent with observations in the region (from the Dutch Waterbase </p><p>Figure 4.2. Modelled current in-situ pH range across the annual cycle. Note that boundary errors are causing an unrealistic result for the Baltic outflow region.</p><p>375ppm</p><p>Diff = 0.10 Mean = 8.03</p><p>1000ppm</p><p>Diff = 0.48 Mean = 7.65</p><p>Figure 4.3. Modelled surface pH fields and means for contemporary and one future atmospheric CO2 scenarios applied to the whole UK shelf. The mean surface value and the difference from pre-industrial pH is indicated.</p><p>36 database) and observations of similar systems (Hinga, 2002). It is suspected that, in the immediate vicinity of some of the major riverine inputs, the accuracy of the modelled pH derivation is affected by lack of accurate parameterisation of the riverine chemistry, particularly the simplification that total alkalinity is solely derived from salinity. Evidence (Borges & Frankignoulle, 1999; Pätsch & Lenhart, 2004) suggests that, apart from between river variation, river plumes exhibit significant seasonal trends. Off shore, away from riverine influence, the annual pH range is much reduced, generally < 0.4 pH units.</p><p>Results from IMCO2 European Region Sea Ecosystem Model (ERSEM) simulations suggest that North Sea pH will be 0.2 pH units lower than pre-industrial by 2050 and may decrease by a further 0.13 – 0.28 pH units by 2100, depending on emissions (Blackford and Gilbert, 2007). All scenarios of atmospheric CO2 result in a consistent decline in pH across UK shelf waters (Figure 4.3). These estimates are consistent with modelled global oceanic acidification rates (e.g. Caldeira and Wickett, 2003). The simulations suggest that by 2050 some areas of the North Sea will be experiencing a pH range completely distinct from current levels, although the majority of the region retains some degree of range overlap. By 2100 much of the region will have a distinct range. Exceptions are restricted to near shore environments which are predominantly forced by riverine inputs and experience ranges of ~1.0 pH unit. The degree of overlap in these near river zones is probably exaggerated for two reasons; the riverine biogeochemistry, particularly the TA loadings are not well represented and the river loads have been kept constant between each scenario. There is also a tendency for excessive diffusion and transport of river plumes by the 3D hydrodynamics, arising from the 7km horizontal resolution.</p><p>Acidification and Calcifying Organisms</p><p>Ocean acidification is only just emerging as a substantial future threat to marine organisms and ecosystems – on a par to climate change impacts (Raven et al., 2005; IPCC, 2007). For this reason, at the present time we have only have a limited understanding as to which organisms may be impacted by these pH changes, and how exactly they will be impacted. There is an increasing body of evidence to suggest that calcifying organisms (those using calcium carbonate to build shells or skeletons) will be affected but non-calcifiers may be impacted too (see below). The reason that acidification is observed to affect calcifiers is probably related to the link between 2- 2+ CaCO3 saturation state (Ω, = [CO3 ][Ca ]/Ksp) and pH. The value of Ω has been found to control inorganic calcification and dissolution in laboratory experiments. Values of Ω above 1 correspond to the water being more supersaturated with respect to calcium carbonate. Spontaneous inorganic precipitation (crystallisation) is increasingly favoured. As Ω decreases to values progressively lower than 1, dissolution of calcium carbonate becomes more and more likely, or proceeds more and more rapidly. A value of Ω=1 represents the boundary between supersaturation and undersaturation.</p><p>Given the paucity of data, this assessment can only be extremely rudimentary in nature, and we are only able to sketch out general principles. </p><p>37 From the GLODAP open-ocean dataset it is clear that Ω declines with latitude. This derives from the effect of temperature on CO2 solubility. Colder waters can hold a greater concentration of CO2 molecules while still being at equilibrium with the same overlying atmospheric partial pressure of CO2. For this reason, waters at higher latitudes tend, on the whole, to have higher TCO2 concentrations, which in turn leads to lower values of Ω. In line with this general understanding, computer models predict that the first waters to go undersaturated (Ω<1) will be polar waters (Orr et al., 2005). In the context of the UK, it is therefore possible that, as pH declines into the future, more northerly parts of UK waters will experience undersaturation before more southerly parts.</p><p>A second general principle is that deeper waters (below the thermocline) tend to be more acidic (and as a consequence have lower values of Ω) than surface waters, due to decomposition of organic matter. Ω also declines with depth because of a pressure effect, such that waters below several kilometres depth are usually undersaturated; any calcium carbonate falling onto the seafloor at such greater depths is dissolved. Waters at depths of only a few hundred metres have intermediate saturation states but are likely to become undersaturated before surface waters, as long as anthropogenic carbon is able to reach them. The invasion of anthropogenic CO2 into surface waters is translated into an effect on deeper waters in the vicinity of the UK, due to deep water formation taking place in the Greenland-Iceland-Norwegian Seas, and due to deep winter mixing in these seas and in the northeastern North Atlantic. Deep water coral communities, also known as cold water corals, have recently been found along most of the northwest European shelf break, including the shelf break to the west and northwest of Scotland at sites such as Mingulay Reef. Models of future aragonite saturation horizons imply that penetration of anthropogenic CO2 will result in 70% of such reef sites experiencing undersaturated waters by the end of this century (Guinotte et al., 2006).</p><p>A third general principle is that wintertime values of surface water pH and Ω tend to be lower than summer values, in temperate locations experiencing seasonal stratification and strong spring blooms, such as is the case in most waters around the UK (Figure 4, Merico et al., 2006). Seasonal stratification (and therefore large annual variations in pH and Ω) occurs in the northern North Sea but not the southern North Sea. Much of the English Channel is typically well mixed by tides throughout the year, whereas the Irish Sea has a mixture of well mixed, stratified and transitional waters (Pingree and Griffiths, 1978). The consequent probable lack of a sustained post-spring elevation of Ω in the regions that never fully stratify, such as the southern North Sea, makes it likely that undersaturation in summer will be reached first in these areas. How the latitudinal and stratification effects will combine is not well understood. </p><p>38</p><p>Fig 4.4. Model-calculated relationship between phytoplankton seasonal dynamics and the carbonate system, in the eastern Bering Sea (Merico et al., 2006).</p><p>Calcifying phytoplankton (coccolithophores) include bloom-forming species, most notably Emiliania huxleyi are particularly common in certain parts of the northeastern North Atlantic and the northwest European Shelf. Due to their high visibility in satellite images we have a good understanding of where these blooms do and do not occur around the UK. Blooms occur frequently in the northern North Sea, but not the southern North Sea. They also occur in the vicinity of the Scilly Isles, in the Celtic Sea, Normandy Shelf, Bay of Biscay and along the shelf break including to the west of Ireland. They have not been seen to occur at all, however, in the Irish Sea. Large blooms also occur in the western English Channel, to the south of Devon and Cornwall, but blooms have not been observed in the eastern Channel. Bloom concentrations occur out towards deeper water to the northwest of Scotland.</p><p>Less is known about where calcifying zooplankton (foraminifera and pteropods, for instance) are abundant in the waters around the UK, although the data collected by the Continuous Plankton Recorder (CPR) should be ideal for this purpose.</p><p>Surface-water corals are restricted to lower latitudes and do not occur around the UK. Recently-discovered deep-water corals occur along the shelf break to the west and northwest of Scotland at extensive sites, such as Mingulay Reef, but their full distribution is unknown. </p><p>Shellfish also use calcium carbonate as the building material from which to construct their shells, including mussels, oysters, barnacles, whelks, clams, winkles, cockles and scallops. Other commercially important calcifiers include lobsters and crabs (Kleypas et al., 2006). There is accumulating evidence that many of these organisms could be affected by declining pH and this is of concern to shellfish industries around the UK. </p><p>39 Many other benthic animals also produce shells from calcium carbonate, including the sea urchin and starfish groups. Impacts on egg production and development, pelagic larvae and juveniles and their settlement and recruitment have been raised as a particular concern (Turley et al., 2006) and research in this area is just beginning. The susceptibility of impacts of a future decline in pH and rise in CO2 to organisms and ecosystems is of concern and has been reviewed for the OSPAR area in Haugan et al. (2006). </p><p>Acidification and Non-Calcifying Organisms</p><p>Impacts on calcification have received more research attention than other processes so it is tempting to focus on calcification and calcifying organisms. However, reviews of the impact of ocean acidification (Raven et al., 2005; Haugen et al., 2006) conclude that most organisms and ecosystems are at potential risk either directly or indirectly from the chemical changes caused by increasing ocean CO2. Indeed, other key processes besides calcification, such as primary production, nitrification, nutrient regeneration may also be impacted. For example, the lower pH expected over the next 100 years can theoretically impact the speciation of many chemical elements (including oxidation states) (Zeebe and Wolf-Gladrow, 2001; Caldeira & Wickett, 2005). These include key nutrients (N, P, Si) and micronutrients (Fe, Co, Mn etc). For instance, a decrease in pH of 0.3 units could reduce the fraction of NH3 by around 50% (Raven, 1986). Clearly, unravelling the combined impacts of declining pH on nutrient concentration and speciation, on nutrient uptake by natural phytoplankton assemblages, their primary production and composition or their nutritional value to the organisms that feed on them will be a challenge. There is evidence that rates of nitrification are sensitive to pH, with a reduction of ~ 50% at pH 7 (Huesemann et al., 2002). While pH is unlikely to fall to these levels, it does indicate the sensitivity of the process. This may result in a reduction of ammonia oxidation rates, the accumulation of ammonia instead of nitrate (that is, increasing the NH4:NO3 ratio). Depending on the nutrient requirements and uptake abilities of different species this has the potential to impact the growth and biodiversity of phytoplankton and bacteria. Indeed, animal physiology is also sensitive to rising CO2, investigations in mussels from the Mediterranean Sea suggest that the effects on calcification as well as metabolic depression and the shift in energy allocation occur in parallel (Michaelidis et al., 2005). These effects are drastic, i.e. a growth reduction by more than 50 % was found in mussels kept under CO2 at pH 7.3, a pH value expected for the year 2300. Other investigations show a significant reduction of growth and survival in echinoderms and gastropods from the Pacific at CO2 concentrations just 180 ppm (µatm) above today's values. In animals, high CO2 (hypercapnia) can cause changes to metabolic rate, growth rate, reduced reproductive capability and can result in mortality. Many of these experiments have been carried out at CO2 levels far higher than what will occur with ocean uptake of atmospheric CO2. However, little is known about the impact of the relatively low but long term increases in CO2 on animal and plant physiology. Juveniles of the few species studied are more sensitive than adults to the effects of increasing CO2 (Kurihara and Shirayama, 2004) if this occurs widely drastic impact on ecosystems may occur. Most marine phytoplankton are thought to have mechanisms to actively concentrate CO2, a carbon concentrating mechanism (CCM). Some investigations have shown that changes in seawater pH and CO2 have little (<10%) if any direct effect on their growth rate or their elemental composition (e.g. Beardall and Raven, 2004; Giordano et al., 2005). However, taxon specific </p><p>40 differences in CO2 sensitivity have been observed in laboratory culture (Rost et al., 2003) it is currently unknown whether a reduction of the advantage of possessing CCM will impact phytoplankton species diversity in the natural environment. This is a possibility and, should it occur, may impact the contribution of different functional groups, primary production, food web structure and marine biogeochemical cycles. </p><p>Other important changes to the oceans, besides ocean acidification, are predicted to occur this century which could have synergistic impacts on marine ecosystems (IPCC2007). Surface water temperature is already increasing and predicted to increase further, changes in rainfall and land run off will impact salinity and nutrient input to coastal seas. Increases in temperature will increase the degree of stratification of the water column (unless currents and wind mixing increase to balance this) and would reduce the influx of nutrients from below the thermocline that sustains primary production. The potential risk of hypoxia or anoxia in shelf sea ecosystems could increase. The combined impact of ocean acidification and other changes could well influence the relative composition, productivity, timing, location and predominance of the major functional groups of phytoplankton, zooplankton and benthic dwelling organisms and thereby impact the rest of the food web (IPCC 2007). Models that consider the key interactions of these functional groups and their response to a high CO2 world are key to making predictions of the overall impact on marine biogeochemical cycles and food webs. Experimentalists will also need to consider multi-factorial impacts to help provide information to drive or test the models. </p><p>Our conclusion from this brief summary of vulnerability to acidification is that all UK water will experience declining pH with different regions behaving in different ways that we do not fully understand. A wide variety of processes and functional groups are potentially vulnerable to reduced pH or increased seawater CO2 and we do not yet understand the impacts that this will have. For these reasons, including the scarcity of data, no spatial prioritisation can yet be made. Further work is needed on the impact of decreasing pH, increasing temperature etc on important functional groups and further effort is required on the development of predictive ecosystem models. A future pH monitoring strategy would sensibly be part of a programme monitoring other important changes to UK coastal waters.</p><p>41 References</p><p>Beardall J & Raven J A (2004). The potential effects of global climate change in microalgal photosynthesis, growth and ecology. Phycologia 43, 31–45. Blackford, J.C., Gilbert, F.J., 2007. pH variability and CO2 induced acidification in the North Sea. Journal of Marine Systems 64, 229-241 Borges, A. V., Frankignoulle, M., 1999. Daily and seasonal variations of the partial pressure of CO2 in surface seawater along Belgian and southern Dutch coastal areas. Journal of Marine Systems 19, 251-266. Caldeira, K. and Wickett, M.E., 2003. Anthropogenic carbon and ocean pH. Nature 425, 365-365. Caldeira, K. and Wickett, M.E., 2005. Ocean model predictions of chemistry changes from carbon dioxide emissions to the atmosphere and ocean. J. Geophys. Res.- Oceans 110, C09S04, doi:10.1029/2004JC002671. IPCC (2007) Fischlin A., Midgley G.F., Price J., Leemans R., Gopal B., Turley C., Rounsevell M., Dube P., Tarazona J., Velichko A. Intergovernmental Panel for Climate Change Fourth Assessment Report, Working Group II, Chapter 4 – Ecosystems, Their Properties, Goods, and Services. Giordano, M., Beardall J. & Raven J. A. (2005). CO2 concentrating mechanisms in algae: mechanism, environmental modulation, and evolution. Annual Review of Plant Biology 56, 99–131. Guinotte, JM et al. 2006. Will human-induced changes in seawater chemistry alter the distribution of deep-sea scleractinian corals? Frontiers in Ecology and the Environment 4, 141–146. Kleypas, J.A., R.A. Feely, V.J. Fabry, C. Langdon, C.L. Sabine, & L.L. Robbins (2006) Impacts of Ocean Acidification on Coral Reefs and Other Marine Calcifiers: A Guide for Future Research, report of a workshop held 18–20 April 2005, St. Petersburg, FL, sponsored by NSF, NOAA, and the U.S. Geological Survey, 88 pp. Kurihara, H. and Shirayama, Y. (2004) Effects of increased atmospheric CO2 on sea urchin early development. Mar. Ecol. Prog. Ser. 274, 161-169. Haugan, P.M; Turley,C; Poertner H.O. (2006) Effects on the marine environment of ocean acidification resulting from elevated levels of CO2 in the atmosphere, DN- utredning 2006-1, 1-36. Huesemann, M.H., Skilmann A.D. & Crecelius E.A (2002). The inhibition of marine nitrification by ocean disposal of carbon dioxide. Marine Pollution Bulletin 44, 142–148. Hinga, K.R. (2002). Effects of pH on coastal phytoplankton. Mar. Ecol. Progr. Ser. 238, 281–300. Merico, A, Tyrrell, T. and Cokacar, T. (2006) Is there any relationship between phytoplankton seasonal dynamics and the carbonate system? Journal of Marine Systems 59, 120-142. Michaelidis, B., C. Ouzounis, A. Paleras and HO. Pörtner (2005): Effects of long-term moderate hypercapnia on acid-base balance and growth rate in marine mussels (Mytilus galloprovincialis). Mar. Ecol. Progr. Ser. 293, 109-118. Orr, J.C. and co-authors, 2005. Anthropogenic ocean acidification over the twenty- first century and its impact on calcifying organisms. Nature 437, 681-686. Pätsch, J., Lenhart, H. J., 2004. Daily loads of nutrients, total alkalinity, dissolved inorganic carbon and dissolved organic carbon of the European continental rivers for the years 1977-2002. Berichte aus dem Zentrum für Meeres- und Klimaforschung, Reihe B: Ozeanographie.</p><p>42 Pingree R. D. and Griffiths, D.K. (1978) Tidal fronts on the shelf seas around the British Isles. Journal of Geophysical Research 83 (C9), 4615-4623. Raven, J.A. (1986) Physiological consequences of extremely small size for autotrophic organisms in the sea. In: Photosynthetic Picoplankton 214 (eds Platt, T. and Li, W.K.W.) 583. Canadian Bulletin of Fisheries and Aquatic Sciences: Ottawa, Canada. Raven, J., Caldeira, K., Elderfield, H., Hough-Guldberg, O., Liss, P., Riebesell, U., Shepherd, J., Turley, C.M. & Watson, A. (2005) Ocean acidification due to increasing atmospheric carbon dioxide. The Royal Society Policy document 12/05, pp. 68. Rost B, Riebesell U, Burkhardt S & Sültemeyer D (2003). Carbon acquisition by bloom-forming marine phytoplankton. Limnology and Oceanography 48, 55–67. Turley, C., Blackford, J., Widdicombe, S., Lowe, D., Nightingale, P.D. & Rees, A.P. (2006) Reviewing the impact of increased atmospheric CO2 on oceanic pH and the marine ecosystem. In: Avoiding Dangerous Climate Change, Schellnhuber, H J., Cramer,W., Nakicenovic, N., Wigley, T. and Yohe, G (Eds), Cambridge University Press, 8, 65-70. Zeebe RE & Wolf-Gladrow D (2001) CO2 in Seawater: Equilibrium, Kinetics, Isotopes. Elsevier Oceanography Series Vol.65, 360pp.</p><p>43</p>
Details
-
File Typepdf
-
Upload Time-
-
Content LanguagesEnglish
-
Upload UserAnonymous/Not logged-in
-
File Pages43 Page
-
File Size-