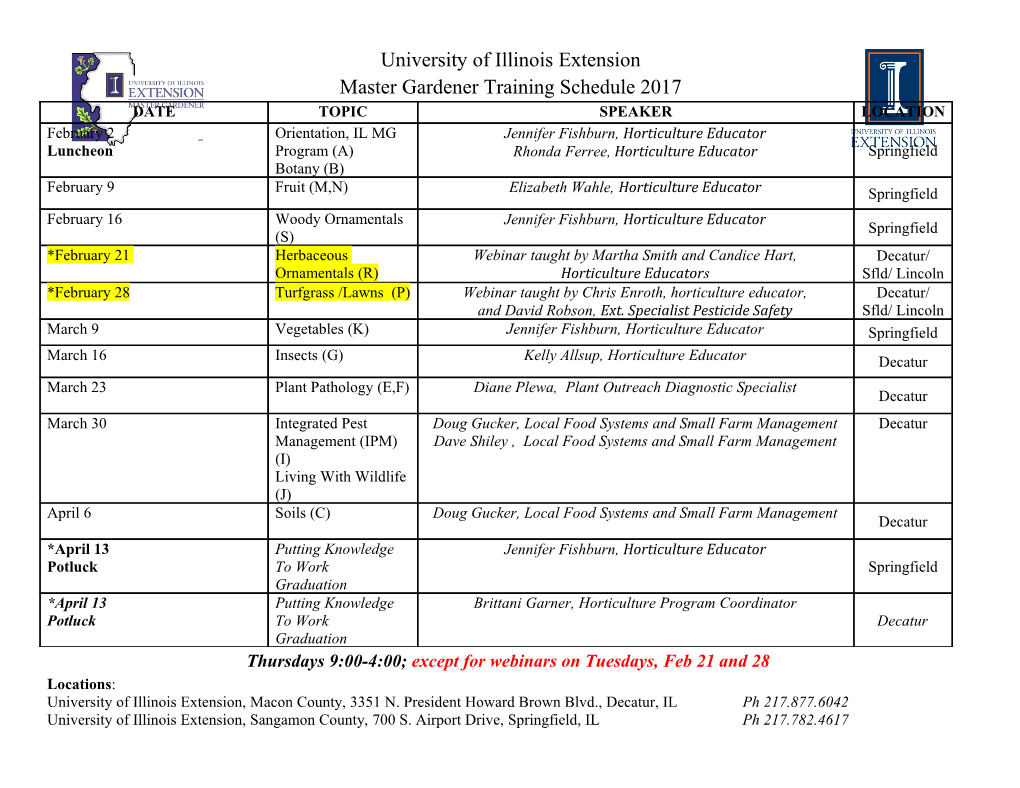
Introduction to Oxidation and Mass & Energy Balances Michael Mannuzza IT3/HWC OBG BaltimoreBaton Rouge,2014 LA 2016 Combustion • Combustion is an oxidation reaction: Fuel + O2 ---> Products of Combustion + Heat Energy • In addition to traditional burner fuels, incinerator fuel can include solid, gaseous and liquid waste. IT3/HWC • The Products of Combustion (POC) are the BaltimoreBaton primary concern from an Air Pollution Control Rouge,2014 LA 2016 (APC) perspective. Air Pollutants and Regulatory Drivers • The type of APC system required for an incinerator will be decided based on the system’s POCs and the regulatory limits mandated for specific pollutants. • Emissions of the following pollutants are typically regulated for most incinerator applications: • IT3/HWC • Dioxin/Furans NOx • Particulate Matter BaltimoreBaton • Mercury (Hg) Rouge,2014 LA • VOCs & Total Hydrocarbons 2016 • Semi-Volatile Metals (Cd, Pb) • Carbon Monoxide • Low-Volatility Metals (As, Be, Cr) • HCl & Cl2 • SOx • Others Identifying APC Requirements Three Steps: 1. Quantify anticipated POCs 2. Identify Regulatory Emission Constraints (establish abatement requirements) IT3/HWC 3. Quantify the discharge flow rate of the system. BaltimoreBaton Rouge,2014 LA 2016 Waste Feed Properties I. Begin by identifying the properties of the waste feed and quantifying the constituency of the waste: 1. Material Take-Offs 2. Proximate, Ultimate, and Ash Analysis 3. Sample & Analyze for Specific Compounds 4. Employ Other Empirical Methods PROXIMATE ANALYSIS ULTIMATE ANALYSIS ASH ANALYSIS Category wt % Category wt % Category wt % IT3/HWC IT3/HWC Moisture 3.3 Carbon (C) 61.1 Silicon Dioxide (SiO2) 74.1 BaltimoreBaton Ash 22.1 Hydrogen (H) 3.0 Aluminum Oxide (Al2O3) 20.0 Rouge,2014 LA 2016 Volatile Matter 27.3 Nitrogen (N) 1.35 Iron Oxide (Fe2O3) 3.25 Fixed Carbon 47.3 Sulfur (S) 0.4 Calcium Oxide (CaO) 0.68 Gross Calorific Value 24.73 Oxygen (O) 8.8 Magnesium Oxide (MgO) 0.48 Other 25.25 Other 1.49 *Ash Fusion Point = 1104 ⁰C Waste Feed Properties •If possible, identifying specific compounds in the waste is the best approach. GASEOUS WASTE STREAMS LIQUID WASTE STREAMS COMPOUND NAME % VOLUME COMPOUND NAME Wt. % Methane 56.33 - 66.40 Toluene 8.4 -11.0 Ethane 1.27 - 3.8 Xylene 1.2 – 3.1 Propane 0.75 -1.2 Propanol 1.9 -17.1 Butane 0.05 -1.0 Isopropylbenzene 0.08 – 9.3 IT3/HWC IT3/HWC Octane 0.02 -.08 Acetic Acid 0.5 – 0.9 BaltimoreBaton Pentane 0.13 – 1.1 1,2 Dichloroethane 1.1 – 13.1 Rouge,2014 LA Hexane 0.11 – 1.0 Methanol 0.9 – 5.0 2016 Carbon Monoxide 17.40 -21.0 Methyl Bromide 0.9 -1.5 Benzene 0.00 -0.21 Formaldehyde 1.1 -6.7 Toluene 0.00 – 0.46 Sodium Fluoride 1.0 – 1.5 Methylene Chloride 0.01 – 0.8 Hydrogen Chloride 4.5 – 9.5 Water (g) 2.95-23.93 Water (L) 21.3 – 78.42 Waste Feed Properties I. Waste feed rates and constituencies are rarely constant. It is important to establish a realistic waste feed design basis. The waste feed design basis must address the following: I. Worst case feed rate II. Highest heating value condition (kJ/kg of waste) III. Lowest heating value condition (kJ/kg of waste) IT3/HWC IV. Worst case sulfur condition, chlorine condition,NOx condition, metals condition, particulate condition, etc. BaltimoreBaton Rouge,2014 LA V. Any other critical regulatory or production related criteria associated 2016 with the waste. Stoichiometry of Combustion I. As a starting point, it is necessary to make some Initial assumptions. If adequate Oxygen will be made available for complete combustion, assume the following: I. All Carbon converts toCO2 II. All halogens convert to acids (e.g., Cl HCl, Br HBr) III. All alkali metals convert to hydroxides (e.g., Na NaOH) IV. All remaining hydrogen converts to H2O IT3/HWC V. All non-alkali metals convert to metal oxides in their most common BaltimoreBaton oxidation state (e.g., MgO, Fe2O3) Rouge,2014 LA 2016 VI. Bound Nitrogen in the waste converts to NO2 VII. All Sulfur converts to SO2 VIII. Non-combustible constituents pass through unchanged or thermally decompose to known compounds Stoichiometry of Combustion I. In reality, it is possible that additional products or congeners can be formed. I. SO3 II. Diatomic Halogens (Cl2, Br2 ) III. N2, NO, N2O IV. CO V. Dioxin/Furans VI. Others IT3/HWC BaltimoreBaton II. Formation of these tertiary compounds can be a function of: Rouge,2014 LA I. Temperature 2016 II. Contaminant Concentrations III. O2 and H2O Concentrations IV. Catalysts V. Other factors Stoichiometry of Combustion I. The initial assumptions outlined usually provide a reasonable estimate of the products of combustion that are generated. I. Allows the elemental contaminants to be quantified. II. Provides a mechanism for identifying combustion air requirements and exhaust flow rates II. The resulting POCs can then be refined if necessary by applying: IT3/HWC I. Empirical Data BaltimoreBaton Rouge,2014 LA II. Advanced Methods 2016 Stoichiometry of Combustion • Example: Dichlorobenzene, C6H4Cl2 C6H4Cl2 + ?O2 ?CO2 + ?H2O + ?HCl C6H4Cl2 + 6.5O2 6CO2 + 1H2O + 2HCl 1. Balance Carbon atoms first IT3/HWC BaltimoreBaton 2. Balance halogens, metals, Nitrogen & Sulfur next. Rouge,2014 LA 2016 3. Balance Hydrogen atoms 4. Balance Oxygen atoms last Stoichiometry of Combustion 1. Balance Carbon & CO2 first: C6H4Cl2 + ?O2 ?CO2 + ?H2O + ?HCl How many moles of CO2? Answer: 6 IT3/HWC BaltimoreBaton Rouge,2014 LA C6H4Cl2 + ?O2 6CO2 + ?H2O + ?HCl 2016 Stoichiometry of Combustion 2. Balance halogens, metals, Nitrogen & Sulfur next: C6H4Cl2 + ?O2 6CO2 + ?H2O + ?HCl How many moles of HCl? Answer: 2 IT3/HWC BaltimoreBaton Rouge,2014 LA C6H4Cl2 + ?O2 6CO2 + ?H2O + 2HCl 2016 Stoichiometry of Combustion 3. Balance Hydrogen atoms: C6H4Cl2 + ?O2 6CO2 + ?H2O + 2HCl How many moles of H2O? Answer: 1 IT3/HWC BaltimoreBaton Rouge,2014 LA C6H4Cl2 + ?O2 6CO2 + 1H2O + 2HCl 2016 Stoichiometry of Combustion 4. Balance Oxygen atoms last: C6H4Cl2 + ?O2 6CO2 + 1H2O + 2HCl How many moles of O2? Answer: 6.5 IT3/HWC BaltimoreBaton Rouge,2014 LA C6H4Cl2 + 6.5O2 6CO2 + 1H2O + 2HCl 2016 Stoichiometry of Combustion – Mass Balance C6H4Cl2 + 6.5O2 6CO2 + 1H2O + 2HCl Compound: C6H4Cl2 O2 CO2 H2O HCl Molecular Wt. (g/mol): 147.004 32.00 44.011 18.0158 36.461 No. of Moles: 1 6.5 6 1 2 1Total Wt. (g): 147.004 208.00 264.066 18.0158 72.9218 IT3/HWC 2Normalized Ratio: 1 1.415 1.796 0.123 0.496 BaltimoreBaton 1Total Wt. = (Molecular Wt.) * (No. of Moles) Rouge,2014 LA 2Normalized Ratio = Total Wt. divided by the molecular wt of C H Cl (147.004 g/mol). 2016 6 4 2 Thus: 1kg C6H4Cl2 + 1.415kg O2 1.796kg CO2 + 0.123kg H2O + 0.496 kg HCl Same applies for grams, pounds or any unit other unit of mass. Stoichiometry Calculations This approach can be applied to each constituent identified in a waste stream: STEP 1: Balance the moles IT3/HWC STEP 2: Balance the mass BaltimoreBaton Rouge,2014 LA 2016 Stoichiometry Calculations • Each constituent can be factored by its mass ratio and then summed to generate a representative compound that reflects the properties of the overall waste stream. • Similarly, a weighted calculation can be performed to determine the net heat of combustion of the waste stream. IT3/HWC BaltimoreBaton Rouge,2014 LA 2016 Heat of Combustion Definitions: • Heat of Combustion: The heat released by combustion of a unit quantity of fuel with its stoichiometrically correct amount of combustion air, measured either in calories or Btu. • Gross Heating Value:The heat released by combustion of a unit quantity of fuel with both the combustion air and fuel at a known reference temperature prior to combustion (e.g. 60 ⁰F) after the IT3/HWC products of combustion are allowed to cool to the initial BaltimoreBaton temperature. Also known as Higher Heating Value (HHV). Rouge,2014 LA 2016 • Net Heating Value: The heat release measured prior to the products of combustion being allowed to cool. Also known as Lower Heating Value (LHV). Heat of Combustion • For most incinerator applications, we are concerned only with the LHV of the fuel/waste. • Numerous data sources are available (reference books, internet, etc.). • Can be estimated or obtained through testing. • Heat of formation • Btu/lb = 14,544C+62,028(H2-0.125O2)+4,050S IT3/HWC Dulong’s Approximation ( ) • Empirical formula based on coal. BaltimoreBaton Rouge,2014 LA • Can be applied to other carbonaceous waste, but accuracy is questionable. 2016 • 410 Btu (103.3 kcal) per Mole of O2 Consumed • Good approximation for hydrocarbons. • Accuracy diminishes if Oxygen, Nitrogen, Halogens and other elements are present. • Mass of exhaust stream Mass & Energy Balance constituents (solid, gas) • Heat content of constituents Mass in = Mass out Energy in = Energy out Thermal losses Control volume • Mass of burner fuel and IT3/HWC combustion air BaltimoreBaton • Heat content of constituents Rouge,2014 LA • Net heat of combustion of 2016 burner fuel • Mass of waste stream constituents (solid, liquid, gas) • Heat content of constituents • Net heat of combustion of combustibles Mass & Energy Balance Conservation of Mass & Energy mstream1 + mstream2 + mstream3 =mstream4 Qstream1+Qstream2+Qstream3 =Qstream4 + Thermal Losses IT3/HWC BaltimoreBaton Rouge,2014 LA 2016 Mass & Energy Balance –Key Points I. Mass in must equal mass out (m in – mout = 0). II. Energy in must equal energy out (Q in – Qout = 0). III. Combustion air volume will generally be a direct function of the fuel input, however, additional air may be needed to maintain O2 levels or control temperature. IV. Adjust the mass of the fuel input until the system energy is IT3/HWC balanced. I. Cannot solve directly - must be an iteration. BaltimoreBaton Rouge,2014 LA II.
Details
-
File Typepdf
-
Upload Time-
-
Content LanguagesEnglish
-
Upload UserAnonymous/Not logged-in
-
File Pages34 Page
-
File Size-