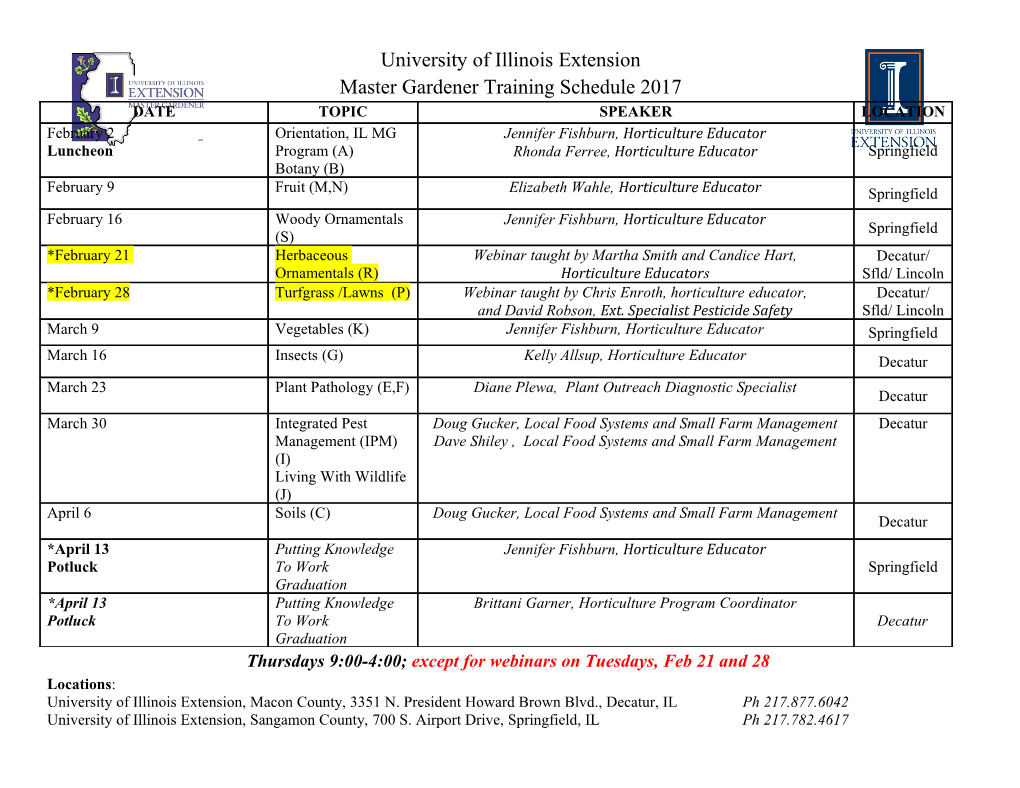
<p>A.P. Biology Notes Cells & Energy</p><p>Cell Theory 1. Cells are the basic unit of structure. [All living organisms are composed of cells.] 2. Cells are the basic unit of function. [Cells are the smallest units of life.] 3. All cells come from preexisting cells.</p><p>History of Cell Theory: Robert Hooke [1665]: coined term cells (from cork observations) M.J. Schleiden [1838]: all plants composed of cells Theodor Schwann [1839]: all organisms composed of cells Rudolf Virchow [1858]: all cells come from preexisting cells</p><p>Cell Size 1 meter = 103 mm = 106 µm = 109 nm most cells 1 — 100 µm diameter surface to volume ratio limits size: as diameter increases (r) surface area increases as square (r2) volume increases as cube (r3) • so as r increases - the ratio of surface to volume, r2/r3, decreases - materials cannot pass through membrane fast enough</p><p> A cell is a metabolic compartment where a multitude of chemical reactions occur. The number of reactions increase as the volume within a cell increases. All raw materials necessary for metabolism can enter the cell only through its cell membrane. The greater the surface area the larger the amount of raw materials that can enter at one time. Each unit of volume requires a specific amount of surface area to supply its metabolism with raw materials. The amount of surface area available to each unit of volume varies with the size of a cell. As a cell grows its SA:V decreases. At some point in its growth its SA:V becomes so small that its surface area is too small to supply its raw materials to its volume. At this point the cell cannot get larger. So what can cells do about this: a. Divide b. Slow down metabolism A.P. Biology Notes The Cell Page 2 c. Change shape to increase the surface area (ex. get long thin or flat, or develop many folds in the cell membrane to increase SA and therefore SA:V ratio</p><p>Types of Cells prokaryotes- bacteria and cyanobacteria (archaebacteria) lack nuclear membrane, DNA without histones, no distinct organelles (except ribosomes) eukaryotes- animal, plants, protists, and fungi distinct nucleus, DNA associated with histones in complex chromosomes, organelles, intercellular membrane system plant cells: cell walls, chloroplasts, large vacuole, (no centrioles) animal cells: centrioles, (no cell walls)</p><p>Cell Components (eukaryotes): membranes: composed of phospholipid bilayer (tails in, heads out) proteins embedded in bilayer • compartmentalize cell • provide platform for reactions • used to establish gradients (for work or storage) plasma membrane: membrane enclosing cell • regulate entry and exit of materials • receive messages, identify type and status of cell</p><p>The Nucleus nuclear envelope = double membrane (two phospholipid bilayers) chromosomes: genetic information (DNA + histone proteins) synthesis of mRNA (messenger RNA) ( pattern for proteins) nucleolus: synthesis of ribosomes (used for protein synthesis) ribosomes composed of rRNA (ribosomal RNA) and proteins large subunit + small subunit sites of protein assembly • free ribosomes suspended in cytoplasm proteins used within cell at large • bound ribosomes attached to endoplasmic reticulum proteins to be modified, exported, kept in vacuoles, used in membranes</p><p>The Endomembrane System endoplasmic reticulum continuous with nuclear membrane provides “platform” for reactions A.P. Biology Notes The Cell Page 3 - enzymes in common pathways may be grouped for efficiency rough ER: studded with ribosomes • protein synthesis of certain proteins: – specialized proteins for export (insulin) – membrane proteins (channels) – those requiring specialized processing (insulin) – those to be kept in vacuoles for special occasion (lysozyme) smooth ER responsible for lipid synthesis, membrane production</p><p> golgi complex flattened membrane sacs between ER and plasma membrane site of reactions and packaging for export • vesicles bud off ER and fuse with golgi (forming face / cis face) • additional processing of proteins may occur here • vesicles with products bud off maturing face / trans face - fuse with plasma membrane (export) - or serve intracellular function (lysosomes) lysosomes membrane bag of hydrolytic enzymes: • acid pH, digestive function • rest of cell protected due to membrane-bound • kept concentrated enough for efficiency merge with food vacuoles or damaged organelles - food vacuole will fuse = hydrolysis of macromolecules Vacuoles Formed by (animal cells): endocytosis: phagocytosis: food engulfed by plasma membrane pinocytosis: liquids engulfed by plasma membrane exocytosis: excretion of vacuole by fusion with plasma membrane</p><p> central vacuole: large vacuole found in plant cells - used for storage food, vitamin/minerals, toxins, waste disposal, hydrolysis</p><p>Other Organelles outer inner m em brane m em brane mitochondria: the "powerhouse" of the cell most cells contain 100—1000 mitochondria aprox. 1—10 µm long by 1 µm diameter double membrane: • smooth outer membrane • highly folded inner membrane</p><p> cristae m atrix A.P. Biology Notes The Cell Page 4 - cristae: internal foldings; platform for reactions - matrix: internal fluid site of cellular respiration • final breakdown of glucose - release of energy • ATP is regenerated contain own DNA chloroplasts (plant & algae cells only) outer inner structure: membrane membrane • outer membrane + inner membrane</p><p>• thylakoids: coin shaped sacs stroma - thylakoid membrane: platform for reactions – light converted to chemical energy - NADPH is produced – ATP is produced - grana are stacks of thylakoids stroma - interconnected by thylakoid </p><p> membranes granum thylakoid • stroma: intergrana fluid - synthesis of sugar</p><p> ENDOSYMBIOTIC THEORY! Extracellular Components cytoskeleton: structure, motility, movement microtubules: hollow rods of tubulin protein subunits (25 nm dia.) • cillia and flagella: - responsible for locomotion of motile cells (sperm, protists) • centrioles: - found in animal cells - origin of spindle fibers for separating chromosomes • framework for organelle attachment - guide for motion of organelles and proteins (like a rail system)</p><p> microfilaments: — thin fibers composed of actin — responsible for motion, shape changes of cell — assist in movement of organelles cell connections plasmodesmata: channels of cytoplasm through plant cell walls desmosomes: fix animal cells together A.P. Biology Notes The Cell Page 5 tight junctions: also hold animal cells together but form barrier to travel of extra-cellular fluids – WHY?! gap junctions: cytoplasmic passages between animal cells</p><p>Extracellular Matrix (ECM) - Proteins, glycoproteins, fluid between neighboring cells Animal Cells Ex. Collagen – most abundant glycoprotein in animal cells. Fibronectin – glycoproteins that connect to proteins embedded in plasma membrane (integrins); integrins are further connected to the cytoskeleton attached to the inner side of the membrane. - Since integrins integrate through entire thickness of the plasma membrane, this connection between Fibronectin (ECM) to integrins (through plasma membrane) to cytoskeleton (outside of inner membrane) helps cell communicate what is going on outside the cell to the inside and vise versa. . ECM can influence the activity of genes in the nucleus. . ECM helps coordinate the cells of all the same tissue type.</p><p>Membranes plasma membrane: regulates entry into and out of cells; it is a barrier to the diffusion of polar molecules • phospholipid bilayer - glycerol + 2 fatty acids + phosphate compound - hydrophobic fatty acid tails in toward each other - hydrophylic charged phosphate heads out functions: ◊ barrier to large polar molecules ◊ gives membrane fluid properties • proteins - peripheral: on surface - integral: embedded, spanning membrane functions: ◊ transport across membrane ◊ enzymes ◊ receptors for hormones or other extracellular chemicals ◊ identification (maybe complexed with polysaccharides) A.P. Biology Notes The Cell Page 6 ◊ structural: attachment to other cells attachment to cytoskeleton • glycoproteins (carbohydrate + protein) - receptors - cell identification and recognition • cholesterol: increases membrane fluidity</p><p> glycoprotein phosphate head fatty acid tails </p><p> lipid bilayer </p><p> protein cholesterol </p><p>The fluid mosaic model: A phyiscal description of the cell membrane Fluid part =</p><p>Mosaic part = </p><p>Membrane Transport gradient: difference in concentration between two places (especially across a membrane) simple diffusion: tendency toward even distribution of molecules - net movement of molecules from high to low concentration (driven by second law of thermodynamics) facilitated diffusion: moves molecules from [high] to [low] — molecules pass through carrier proteins in membranes — these proteins are selective — may slow down or regulate rate of diffusion osmosis: diffusion of water across selectively permeable membrane - travels from [high] to [low] of water - pure water has [water] of 100% - the greater the [solute], the lower the [water] (as [solute] increases, [water] must decrease)</p><p> Free water molecules = water not bound to a solute A.P. Biology Notes The Cell Page 7 Clustering of water molecules around hydrophilic substances makes some of the water molecules in a cell “unavailable” to move. • Water moves in response to solute concentration gradients across the membrane. When comparing two solution: - one with greater [solute] is hypertonic - one with lower [solute] is hypotonic - isotonic means solute concentrations are equal</p><p> osmoregulation: control of water balance if solution cells are in is: - too hypertonic plasmolysis (shrinking of cells) - too hypotonic cytolysis (bursting), or turgidity (firmness) [specifically = plants]</p><p>Water Potential (ψ)</p><p>Defn: The combined effects of solute concentration and physical pressure</p><p> Free water moves from a region of high water potential to regions of low water potential. The movement of water can perform WORK W=F/D ψ is measured in megapascals (MPa or Bars) ψ of pure water = 0 in a container open to the atmosphere; at sea level and at room temp.</p><p> water potential equation</p><p>ψ = ψS + ψP A.P. Biology Notes The Cell Page 8</p><p> ψS = solute potential – also called osmotic potential because solutes affect the direction of osmosis.</p><p>- Water molecules bind to solutes reducing the amount of Work the water molecules can perform. - Hence, lowers water potential (ψ)</p><p>- ψS of a solution is always negative; the greater the solute concentration, the more </p><p> negative the ψS </p><p> Given the molarity of a solution, you may need to figure out solute potential (ψS)</p><p>ψS = -iCRT i = inoization constant (how many ions {particles} in water after dissolved [no units] C = concentration of substance (mol/L) R = Pressure constant (always 8.31 liter bars/mol K) T = Temperature (273 + C)</p><p> ψP = pressure potential = physical pressure on a solution (pressure exerted by the air on a solution) - can be positive or negative - application of physical pressure increases ψ</p><p>Active Transport: transport of substances against [gradient] - requires energy (ATP) - allows cells to be “different” than surroundings - proton pump is a great example!</p><p>Cotransport: one molecule is brought across membrane by another which is driven by diffusion potential gradients: (referring to electrical potential energy) - ions inside cell mostly negative; positive ions outside cell - this charge difference attracts ions (opposites attract) the proton pump • protons are “pumped” across a membrane - by light energy in chloroplasts - by electron transport in mitochondria • this builds up gradient (potential energy) - protons diffuse back through enzymes (ATPase) A.P. Biology Notes The Cell Page 9 - converting ADP + P ATP</p><p>Cellular Energetics energy: the ability to do work (work is a force acting over distance) kinetic energy: energy of motion heat energy: molecular motion potential energy: stored energy bond energy: stored in chemical bonds Thermodynamics: study of energy transformations 1st law: energy is not created nor destroyed, can only change form 2nd law: a closed system tends toward maximum disorder entropy (S): a measure of that disorder (randomness) - entropy tends to increase in a closed system (ΔS goes up) • but may decrease in part of system [life] • with bigger increase in rest of system [sun] (lost as heat) Heat of Reaction (ΔH) (Δ = “change in”) difference in total bond energy between reactants and products bond energy: amount needed to break a chemical bond or released when a chemical bond forms reactants products + ΔH ΔH is negative if energy is “lost” to surroundings - these reactions proceed spontaneously (dependant on entropy) [may still require energy to get started] - termed exothermic (output of heat) ΔH is positive if energy is absorbed from surroudings - these reactions need a constant input of energy - termed endothermic (requires input of heat) free energy: ΔG ΔG = ΔH - TΔS equal to heat of reaction with entropy taken into account</p><p> G G</p><p>T im e T im e exergonic reaction endergonic reaction</p><p> exergonic: -ΔG spontaneous, energy is released endergonic: +ΔG must input energy A.P. Biology Notes The Cell Page 10 coupled reactions: energy from an exergonic reaction can drive an endergonic reaction </p><p>ATP: the energy currency of the cell P P P + + P P P adenine ribose 3 phosphates adenosine triphosphate ( indicates high energy bond) energy from the P P bonds can be used to do cellular work: - synthesis: formation of other bonds - transport: moving molecules across membranes - movement: contracting muscles ATP ADP + P + energy ATP can be reformed: - using energy from breaking of other bonds ADP + P + energy ATP</p><p>Equilibrium: most reactions reversible (at least somewhat) reactants products + ΔG rate of a reaction depends on: - ΔG - the concentrations of reactants (or products) - activation energy (“kick” needed to start reaction) equilibrium: when the amount of R P = P R • the forward rate is equal to the reverse rate (rf = rr) • at this point [P] and [R] are constant (but generally not equal) (brackets signify concentration; eg [P] means concentration of products) ] activation energy: energy needed to start reaction</p><p> activation energy</p><p> activation energy with enzyme Reaction enzymes: lower the activation energy speeds up the reaction rate substrate: the reactants for an enzyme enzyme-substrate complex: temporary intermediate A.P. Biology Notes The Cell Page 11 E + S E • S E + P active site: place on enzyme where substrate(s) binds induced fit: - enzyme undergoes conformational change - substrate bonds "stretched" ( more reactive substrate) specificity: one enzyme one reaction (generally) </p><p>[Substrate] Reaction Rate: depends on activation energy (depends on temp) directly proportional to concentration of enzyme max rate when enzyme is saturated</p><p> competitive inhibition molecules similar to substrate bond at active site - no reaction takes place with new molecule - reaction rate with substrate slowed (some enzyme "out of circulation") At very high concentrations of substrate, the inhibitor is swamped and the rate approaches that of the uninhibited reaction.</p><p>[Substrate] irreversible inhibition: inhibitor permanantly binds to enzyme (nerve gases) 1st Substrate allosteric control (allo– = “other”) enzy me 1</p><p>— regulation of enzyme by molecule other than substrate Intermediate A — occurs at effector site (not active site) enzy me 2 product enzyme in equilibrium between active and inactive form binds with Intermediate B enzyme 1 Eactive Einactive enzy me 3 to block promotor: binds to active form excess inhibitor: binds to inactive form Intermediate C production enzy me 4 regulatory enzymes: usually at beginning of pathway negative feedback: final product of pathway inhibits regulatory enzyme Final Product A.P. Biology Notes The Cell Page 12</p><p>Enzyme activity and environment various factors affect enzyme rates and activity ex: temperature, pH, [substrate], ionic conditions, concentrations of enzyme and substrate, inhibitors A.P. Biology Notes The Cell Overview Energy: required by all living systems for synthesis, transport & movement</p><p> cellular respiration: process of converting food energy to ATP ATP: a usable form of energy (“energy currency”)</p><p> phosphorylation: ATP can "energize" other reactions</p><p> oxidation-reduction: reactions in which energy is transferred between molecules </p><p>• glycolysis (anaerobic): glucose pyruvate (= pyruvic acid) Glucose is the basis source of cellular energy aerobic respiration: in the presence of oxygen [most efficient] - + • Kreb’s cycle: pyruvate CO2 + e 's (+ H 's + Energy ) [also called citric acid cycle] - + • electron transport system: e + O2 H2O + H gradient chemiosmosis: H+ gradient ATP anaerobic respiration: without oxygen (fermentation) Pyruvate lactic acid (lactic acid fermentation) Pyruvate ethanol (alcohol fermentation) Proteins and lipids can also be metabolized</p><p>Chemical Energy: stored in chemical bonds combustion: breaking of bonds to release energy (in living systems, C-H bonds store the most usable energy) controlled combustion: when that energy is harnessed (coupled) some bonds specialize in transferring energy: - usually phosphate bonds in living systems - usually transferred from ATP P P P + + P P P adenine ribose 3 phosphates adenosine triphosphate ( indicates high energy bond) phosphorylation: a P is transferred to a molecule (increased E) P P P P P + P The P can be attached to another molecule to make it more reactive. ATP ADP + PO4 </p><p> attachment and release of P used to drive synthesis, transport & movement A.P. Biology Notes Cells & Energy Page 14</p><p>Oxidation - reduction (REDOX) electrons energy associated with stability • with highly electronegative atom (ex: O of O2): – more tightly held – more stable – less energy to be extracted • with less (or equal) electronegative atom: – less tightly held – less stable – energy can be yielded if moved to lower energy state (w/ more electronegative atom)</p><p> oxidation: removal of electrons from a compound/atom (also associated with change of e- attraction relative to another atom) - sometimes whole H atoms removed (dehydrogenation) [e- + proton] - may also happen with addition of oxygen (inorganically = "rusting") - releases energy lowers energy in cmp which is oxidized reduction: addition of electrons - also, addition of whole H atoms (or removal of oxygen) - requires energy raises energy in cmp which is reduced Oxidation-reduction always occurs together - if reduction requires energy, oxidation must supply it - reactions can be coupled to transfer energy</p><p>Example: CH is oxidized CH CO 4 4 2 (energy is released) O2 is reduced O 2 H 2 O (energy is absorbed)</p><p>[oxidation & reduction refer not to isolated substances but to changes occurring to a substance during a rxn] - compounds have lower energy after oxidization - so, if we can reduce them again they'll be "recharged" (capable of yielding electrons and energy again) A.P. Biology Notes Cells & Energy Page 15</p><p>Electron carriers in biological systems: NAD+: nicotinamide adenine dinucleotide (oxidized form) + + NADH: reduced NAD [also written: NADH + H or NADH2] - carries electrons (and Energy ) from glycolysis & citric acid cycle to ETS - oxidation of NADH will ultimately yield ATP (≈3 ATP / NADH) - contains the vitamin niacin - FAD & FADH2: carry e 's from one step of Kreb's (≈2 ATP / FADH2) FMN & FMNH2: gateway to electron transport system - both require the vitamin riboflavin NADP+ & NADPH: involved in photosynthesis (later) </p><p>Glycolysis [ Refer to Glycolysis diagram]</p><p> converted to Glucose-6-phosphate after passing through cell membrane </p><p> amylose (plant storage) glycogen (animal storage) other carbohydrates, sugars & disaccharides Focus: - takes place in cytoplasm - conversion of 6-carbon sugar to two 3-carbon compounds (pyruvate) - initial energy investment via phosphorylation - utilizes coupled reactions to transfer energy to usable forms - net production of ATP (“substrate level”) - removal of electrons for later conversion to ATP (each NADH will yield 3 ATP's in the Electron Transport System) - each reaction requires a specific enzyme - net energy gain is 2 ATP + 2 NADH = 8 ATP</p><p>Aerobic Respiration • begins with glycolysis • requires O2 as the final electron acceptor • occurs in the mitochondria — some enzymes on the inside membrane — others in the matrix • ATP required to move NADH (from glycolysis) into mitochondria — -ATP x 2 = -2 ATP</p><p>+ C O 2 NAD NADH Formation of acetyl coenzymeA: C O O H H CoA</p><p>C O C O C O </p><p>C H 3 C H 3 H CoA C H 3 Pyruvate Acetaldehyde Acetyl coenzym e A A.P. Biology Notes Cells & Energy Page 16 (“Junction reaction”)</p><p>Energy gain is 1 NADH x 2 = 6 ATP (2 pyruvates from each glucose) Kreb's (citric acid) cycle: 2-carbon acetyl-CoA [from glycolysis or fat metabolism] + 4-carbon oxaloacetate 6-carbon citrate - once around the cycle and citrate becomes oxaloacetate again - two carbons are removed as CO2 (not original acetyl carbons) - the original glucose sends two acetyls (2C @) around the cycle Net energy gains from cycle: These gains are approximate under 1 ATP = 2 ATP optimal conditions. 3 NADH = 18 ATP 1 FADH = 4 ATP 12 ATP x 2 pyruvate = 24 ATP</p><p>Aerobic respiration energy balance sheet: 8 ATP Glycolysis (net) -2 ATP NADH into mitochondria 6 ATP Pyruvate Acetyl-CoA 24 ATP Citric acid cycle 36 ATP from one Glucose molecule</p><p>The Electron Transport System: - Transfer of e 's from reduced carriers (NADH & FADH2) to ETS • oxidized carriers (NAD+ & FAD) return to Kreb's cycle & glycolysis • e-'s transported "down" chain liberating energy - • O2 is final e acceptor • will result in creation of ATP (by chemiosmosis) - each NADH will generate 3 ATP's - each FADH2 will generate 2 ATP's Chemiosmosis • electron (& proton) transfer in ETS is coupled to ATP production • ETS carriers bound/embedded in inner mitochondrial membrane — several carriers are cytochromes — contain poryphrin ring (= heme, also in hemoglobin) • as electrons are shuffled between carriers: — they move across (but within) the membrane several times — carrying H+ from matrix to intermembrane space [a proton pump] A.P. Biology Notes Cells & Energy Page 17 • build up of H+ gradient (concentration gradient / chemiosmotic gradient / electrochemical gradient) across inner membrane results in potential energy due to electrochemical gradient [= concentration gradient + electrical gradient] • H+ ions diffuse back to matrix through specialized enzymes — enzymes = ATPase — ATPase activated by passing proton — activated ATPase synthesizes ATP from ADP + P</p><p>Anaerobic Respiration (without oxygen) Not very productive energy wise • can only sustain yeast, bacteria and smallest organisms • use in higher organisms for brief time in high demand situations Glycolysis pyruvate (+ 2 ATP + 2 NADH) • NAD+ must be reformed from NADH for more glycolysis to occur — animals: pyruvate lactate Pyruvate is proton (and e-) acceptor for NADH (as opposed to oxygen for aerobic) — plants & microorganisms: pyruvate ethanol Acetaldehyde acts as H+ (and e-) acceptor (as opposed to oxygen for aerobic) • so: net gain is only 2 ATP per glucose </p><p>Energy from other metabolic pathways Lipids Protein We will not focus on these, but you should review your text for an overview of how to obtain energy from these two macromolecules</p><p>Photosynthesis</p><p>Overview: Reverse of respiration: energy is stored in chemical bonds 6 CO2 + 6H2O C6H12O6 + 6 O2 light reactions: energy is required (sunlight) results in ATP production and reduction of NADPH dark reactions: • CO2 is incorporated into organic molecules ("fixed") via: the Calvin cycle (C3 cycle) (also called Calvin-Benson cycle) • may be made more efficient by — C4 photosynthesis (the Hatch-Slack cycle) or — CAM plants Product of dark reactions is glyceraldehyde phosphate (G3P) G3P can then be: A.P. Biology Notes Cells & Energy Page 18 — used to synthesize macromolecules — used directly for energy — converted to more stable glucose/sucrose to be stored/transport</p><p>Chloroplasts site of photosynthesis (light and dark reactions) double membraned ( endosymbiont hypothesis) grana are stacks of flattened sacs, each termed a thylakoid thylakoid membrane: • where light energy is converted to chemical energy • lipid bilayer (forming thylakoids) also contains - chlorophyll and pigments - cytochromes and enzymes necessary for photosynthesis stroma: fluid surrounding the grana (singular = granum) • where CO2 is fixed into organic compounds (“dark rxns”) </p><p>The Light Reactions chlorophyll: a magnesium containing poryphrin (related to heme) chlorophyll a: - most common plant pigment - the heart of the photosynthetic process (reaction center) - absorbs light energy at a specific wavelength (680 or 700 nm) accessory pigments: carotenoids, xanthophylls and other chlorophylls - form antenna complex along with chlorophyll a - absorb light at other wavelengths - transfer this energy to chlorophyll a - enables more of suns energy spectrum to be utilized [most visible light used except green! (reflected or transmitted)]</p><p> photophosphorylation: light energy is captured in chemical bonds non–cyclic: electrons travel one way from water to use in synthesis. Photosystem I 1. light drives e-'s from PS I chlorophyll to electron acceptor ( E) - 2. e 's passed to ferrodoxin, then used to reduce NADP to NADPH2 • NADPH2 is energy rich • used in Calvin cycle (dark reactions) to make sugars But: the chlorophyll of PS I needs e-'s to recycle, so . . . Photosystem II A.P. Biology Notes Cells & Energy Page 19 3. light drives e-'s from PS II to another acceptor ( E) 4. e-'s passed down electron transport chain (cytochromes) • ATP is generated by chemiosmosis • ATP to be used in Calvin cycle • e-'s finally accepted by PS I chlorophyll But: the chlorophyll of PS II also needs e-'s to recycle, so . . .</p><p> photolysis: 5. water is split providing: • electrons to recycle PS II • protons for reduction of NADP+ to NADPH • oxygen as a by-product cyclic photophosphorylation • uses only photosystem I • electrons from PS I are returned via electron transport chain - ATP is formed - no NADPH2 is produced - no water is used nor is oxygen liberated • used by plants (& prokaryotes?) – in addition to non-cyclic - increases ATP production (ratio of ATP/NADPH2 production in light rxn's not as high as demands of Calvin cycle) chemiosmosis in thylakoids: • protons cleaved from water by light in thylakoid space • additional protons pumped by electron transport into thylakoid space • pass through ATP Synthase (ATPase) to produce ATP in stroma - can be used by dark reactions (w/NADPH2)</p><p>The Light Independent / Dark Reactions (CO2 fixation): in stroma + - - uses H and e from NADPH2 and uses ATP {from light reactions} - uses CO2 {from atmosphere} - produces glyceraldehyde–3–phosphate (G3P) (can also be called 3-phosphoglyceraldehyde) the basic building block for organic synthesis</p><p>The C3 cycle ( Calvin-Benson cycle ) Phase 1: Carbon-Fixation</p><p>- Initial incorporation of CO2 into organic material (RuBP)</p><p> o Using the enzyme rubisco (most abundant protein on earth), C of CO2 attaches RuBP </p><p>--- (3 CO2’s enter at one time) A.P. Biology Notes Cells & Energy Page 20 o Creates a 6-C very unstable molecule – splits immediately = 2 X 3-C molecules</p><p> Phase 2: Reduction</p><p> o Each 3-C molecule is phosphorylated (using ATP)</p><p> o NADPH donates (reduces) these making G3P (where is respiration did we see this molecule?)</p><p> Remember 3 CO2’s entered Calvin Cycle and a split happened (Phase 1), so 6 G3P’s were just made.</p><p> Some G3P’s will be used for phase three</p><p> Some G3P’s will go directly to CR or incorporated into glucose then starch for storage (in roots)</p><p> Phase 3: Regeneration of RuBP</p><p>- Need to make more of that CO2 acceptor RuBP!</p><p> o Some of the G3P just made are rearranged back into the 5-C RuBP (Using more ATP)</p><p>G3P can have two fates : 1. Conversion back to RuBP via the "sugar shuffle" - requires ATP - requires 5 G3P (3-C) to form 3 RuBP (5-C) 2. or: G3P can exit Calvin cycle for use in - respiration (G3P is glycolysis intermediate) - synthesis of glucose and many other organic molecules</p><p>G3P is too reactive for transport or storage • can be used immediately in respiration or • used in synthetic pathways or • converted to glucose or sucrose for transport - can be stored as starches (plants) or glycogen (animals)</p><p>Why C4 pathways? C3 cycle: dependent on CO2 concentration • if high [CO2] Calvin cycle • at low [CO2] photorespiration - RuBP is oxidized by O2 and CO2 is released - no energy is gained – probably evolutionary baggage in C4 plants: PEP carboxylase more efficient at low [CO2] than RuBP carboxylase A.P. Biology Notes Cells & Energy Page 21 - CO2 can be concentrated in bundle-sheath cells (specialized cells) Useful in areas of high temperature and low humidity - stomata can be kept partially closed (antidessication)</p><p>CAM plants stomata: pores in leaf for gas exchange • closed during day prevents desiccation (drying out) • open at night fix CO2 as malate (or aspartate) during day (in light) CO2 is released for Calvin cycle similar to C4 in function except: - happens in same cells - but at different time</p>
Details
-
File Typepdf
-
Upload Time-
-
Content LanguagesEnglish
-
Upload UserAnonymous/Not logged-in
-
File Pages21 Page
-
File Size-