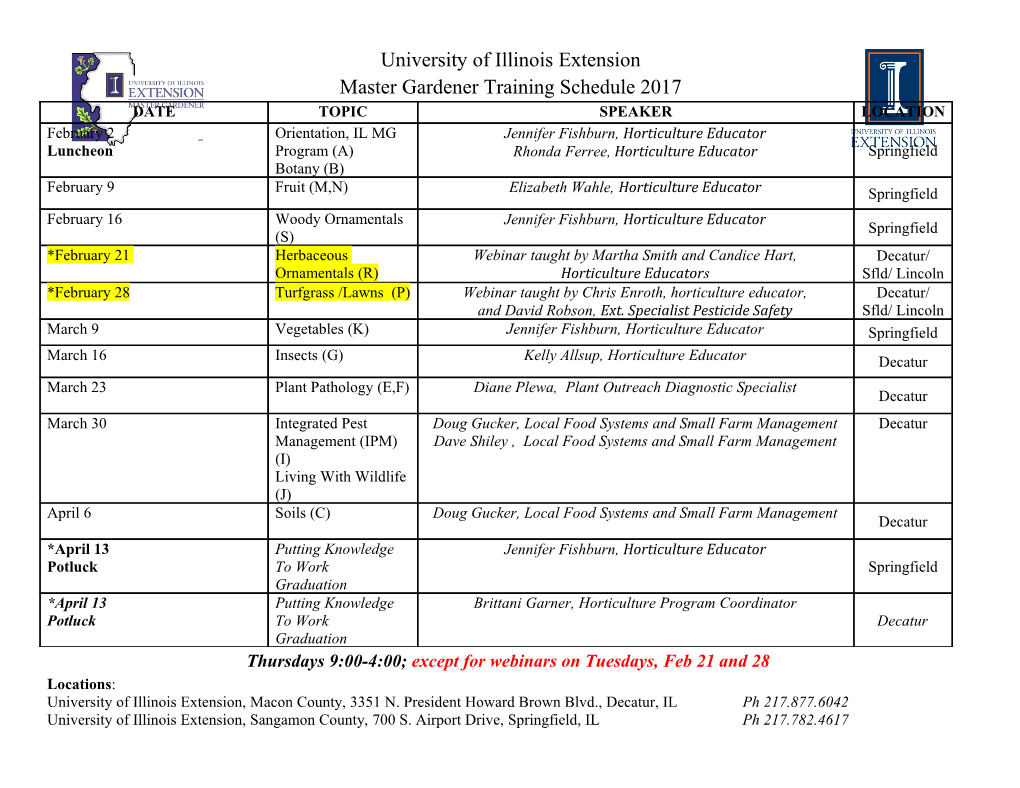
<p> Chapter 2 Materials of the Cell and Matrix </p><p>2.1 Basic Cellular Constituents </p><p>All cellular structures are made with same building blocks: atoms of (in descending concentrations) carbon, oxygen, hydrogen, nitrogen and phosphorus, sulphur, and several other atoms in minute quantities. These atomic building blocks are assembled into larger blocks, i.e. amino acids & sugars, that are then strung together in even larger blocks, ie. proteins and carbohydrates, schematically shown below: </p><p>Figure 2.1 Assembly of basic Proteins structural components of the cell Carbohydrates Biomembranes</p><p>Amino Acids Polymeric Structural Sugars Components Atoms Phospholipids</p><p>Carbon Monomeric Size and Oxygen Building Blocks Complexity Hydrogen Nitrogen Phosphorous, Sulphur, etc.</p><p>As you can see, our study of cell materials is greatly simplified by the fact that all structural components are polymers strung together with 3 types of building blocks. The structural components are mainly proteins, with some carbohydrates. The major categories of cellular constituents are listed in Table 1 below: Table 2.1 Chemical Components of Cells Compound Fraction in Cell Relative Size of Polarity of molecule (%) molecule</p><p>Water 70-80 Small Polarized</p><p>Protein 10-20 Large Regionally polarized (Polypeptide) Lipid 2-20 Medium Non-Polarized (Fat) Carbohydrate 1-2 Medium to large Regionally polarized (Sugars) Salts 1 Small Polarized (Electrolytes)</p><p>Note from the table that electrical polarity varies among the molecules. The importance of these differences to cell mechanics will be appreciated in our study of Energetics in Chapter 7. Note that lipids are non-polarized, however some amino acids are also non- polarized. Carbohydrates can exist as simple sugars, of medium size, or as larger complex chains, such as the backbone of DNA. 2</p><p>Besides polarity and size, other important properties of molecules are their solubility, stability, and shape. As we shall see, proteins are the molecules whose shape is the most varied, and most important determinant of their function.</p><p>In addition to the structural components, a second category of components is the electrolytes, and monomeric molecules dissolved within the cell soup; these include the major salts, and the building blocks: amino acids, sugars, and cell fuels such as ATP. The major electrolytes consist of (in order of descending concentration in the cell): Cl-, K+, Na++, Ca++, and Mg++. These salts are all surrounded by several water molecules, attached by hydrogen bonds. 3 2.2 Arrangement of Cellular Materials To help understand the material make-up of cells and matrix, it is entertaining to imagine how they first sprung up in evolution, even though its details will never be known. The following somewhat fanciful history is therefore to be pondered in that light. In the beginning there was primordial soup: a rich sea filled with organic compounds. Over the eons, as the chemicals began to aggregate in the slime, a critical mass of them was captured within a volume surrounded by a barrier: a lipid membrane. Since oil and water do not mix, a lipid bubble is the logical vessel for the aqueous contents of the cell to reside. Thus a thin hydrophobic shell separated the hydrophilic from their surroundings. With time this primitive cell, a prokaryote would have had to develop structures to survive, since lipid bubbles are easily squashed. Hence the CSK was needed, and the rest is history. Now lets take a look under the hood (Figure 2.2).</p><p>Figure 2.2 Peeling away the cell membrane</p><p>The first thing we notice is that the biomembrane is a lipid bilayer, arranged as an amphiphile. This means that the molecular structure has both hydrophilic and lipophilic parts, as shown in the schematic of a cross-section below. </p><p>H2PolarO Phosphate Heads Figure 2.4 Lipid Bilayer H2O</p><p>70 A</p><p>H2O Hydrophobic core</p><p>The Phosphate heads are negatively charged, so the outside layers of the phospho-lipid sandwich quite happily dissolve in water. The core, composed of long hydrocarbon tails, i.e. fatty acids, behaves oppositely, repelling water and all hydrophilic compounds. Thus the bilayer is elegantly arranged to exist with both faces in water, allowing the middle to act as a barrier to movement of water-based materials. Since the membrane separates the cell plasma from the extra-cellular milieu, it is called the plasma membrane (PM). </p><p>While the PM can be considered part of the CSK, it is relatively weak. It does have measurable stiffness, however, in shear, compression and bending, as will be seen in Chapter 4. Being essentially a Newtonian fluid, the bilayer by itself has no tensile strength. To illustrate, look at the picture sequence below, showing 2 vesicles whose walls are pure lipid bilayers. Starting from the bottom picture, you see the vesicles just touching. The next 2 pictures on top were made as the vesicle at right is pushed out by pressure from the pipette holding it. Note that the vesicles pass into each other. This ghost-like behaviour is due to their liquid nature. </p><p>3. Vesicles pass through each other. Figure 2.5 vesicle ghosts </p><p>2. Pipette pushes vesicle out.</p><p>1. Vesicles make contact </p><p>An anecdote about the biomembrane is that the first estimate of its thickness is attributed to Benjamin Franklin. The story goes that he poured a volume of cooking oil into a small pond until it was entirely covered with oil. He then divided the volume of oil poured by the area of the pond, finding the thickness was about 30 A. The close correspondence of this number with present day measurements of lipid monolayers illustrates the power of simple experiments. It also demonstrates the property of phospholipids to self-assemble into a structure, i.e. a membrane. 2.3 CSK Components Underneath the membrane, and penetrating it at many points, is the CSK. The first set of components that is encountered contains different proteins each with separate functions, as depicted in the schematic diagram below (Figure 2.6). </p><p>Figure 2.6 CSK</p><p>The extracellular matrix (ECM) consists of filamentous proteins, including collagen, fibronectin, vimentin, titin and others. Note that the CSK connects with ECM via Integrin molecules that consist of alpha and beta subunits. These subunits have ligands for specific receptors on matrix proteins. Integrin thus crosses the plasma membrane (PM) to serve as the connector. The standard active ligand of Integrin is the amino acid sequence, RGD. Circulating cells such as white blood cells, and platelets use their Integrin as an antenna to locate cells were their function is needed. The Figure below shows an Integrin of a platelet. With its 2 subunits, integrin resemble both in form and function, a staple. Several other cellular proteins bind with the ECM, as will be detailed in Chapter 11. 4</p><p>Figure 2.7 Integrin The most abundant filament in most cells is filamentous actin (F-actin). This microfilament is most prominent around cell perimeters, and serves as a rope tying the network of other proteins together. Actin can also sustain some compression. A notable exception to this cellular dependence on actin for rigidity is the red blood cell, whose CSK is rich in Spectrin. Actin (specifically F-actin) is integral to contraction and motility in most cells, Another type and configuration of actin is globular, or G-actin. Both types exist in cells, as seen below, with the F-actin stained green, and G-actin stained orange (different gray shades for this B/W rendering). </p><p>F- actin G actin</p><p>Figure 2.8 Actin in a fibroblast</p><p>Many other proteins link the main filamentous actin to the membrane, including tensin, talin, and - actinin, and vinculin, as shown in the cartoon. Although these many proteins, including Integrin, are not abundant, they play crucial roles in mechanical signalling, as we will see in subsequent chapters. </p><p>Recalling from Chapter 1, the CSK can be broken down into 3 major components: microfilaments, intermediate filaments, and microtubules. Single strand diameter of these proteins ranges from about 10 nM for microfilaments, 15 nM for intermediate filaments, and 20 nM for microtubules. Filaments usually form multi-stranded threads, so large bundles of microfilaments are common. Microtubules (MT) are seen in abundance in the cells below, differently shaded from actin. Note that MTs tend to be straighter and run closer to the nuclei, although they extend to cell perimeters as well. MTs are hollow tubes, whereas the other 2 filaments are ropes and rods (See Figure 1.7). MT Actin</p><p>Figure 2.9 </p><p>The CSK is thus structured as a porous network of struts. This plan is, in fact, no different than that of all living materials: even bone, the densest material in the body, is composed of geodesic structures at the meso-scale. The millimeter-size sub –units are arranged in a lattice oriented to maximize strength in the direction of principal stress. Bone, like the CSK is a strong, shock absorbing material that distributes mechanical energy to the network, but tends to focus large, chronic stresses to stronger and thicker support elements.</p><p>It should be noted that CSK composition varies from cell to cell, and even region to region within one cell depending on function as well as developmental state. For example, red cells are rich in spectrin, but deficient in actin. Muscle cells, in contrast, have a very high actin content. Growing or healing cells may have relatively high actin in their growing portions. Some cells, such as skin cells of young humans or certain animals (see below) are rich in elastin. Chemistry of CSK Proteins TO understand the assembly and maintenance of the CSK it is necessary to examine some details of the chemistry of the major proteins. This can be done by dissociating the 3 major proteins from cells, and re-assembling them separately in vitro to study their behaviour. These studies have found some features that are common to all 3, and some that are peculiar to only one. For example, all CSK proteins assemble from monomers, they are all helical, and hence possess multiple equivalent binding sites, they form diverse structures based on statistical mechanics rather than strictly deterministic pathways, and all are polar, that is they each have a chemically distinct head and tail. Actin starts out its existence as a single monomeric protein that is translated from its gene. </p><p>2.4 Cytogel The cytosol is a gel containing electrolytes, amino acids, carbohydrates, metabolic fuels and products, as well as cellular organelles, such as mitochondria and endoplasmic reticulum, and others depending on cell type. The cytoplasm, since it is a gel, contibutes some mechanical stiffness properties to the cell. Ca++ plays a major role in maintaining the ‘cytogel’ since it coordinates polymer-polymer interactions. The cytogel is formed when the negatively charged polymers in the cytosol (proteins and nucleotides) are trapped together, creating osmotic pressure for water influx. A schematic of a gel is shown in Figure 1.8 below. </p><p>Figure 2.10 Gel Gel</p><p>H20 Factors Ca++, pH</p><p>Swelling pressure = osmotic pressure- elastic (compressive) pressure</p><p>Polymer-polymer Intra-polymer osmosis</p><p>Focal adhesion complexes (FACs) are mechanical linkages signal relay stations between the cytoskeleton and ECM[1] Usually attached to the FACs, stress fibers are bundles of actin filaments with myosin; they are components of the cytoskeleton and generate contractile forces during cell crawling, thus serving as a mechanical actuator. </p><p>2. 9 Exercises 1. Analogy has been made between integrin and a staple. Explain how this might apply. 2. Draw a monovalent electrolyte in the hydrated state, using ball and sticks to represent molecules. You need not be quantitatively accurate about the hydration state. How would this compare with a divalent electrolyte? 3. What does polarity have to do with solubility? Give an example. 4. What cellular components serve as ropes or rods? 5. Describe what mechanical role the cytogel might serve. Make a Simulink model of it. 6. Which of the following statements are correct? Explain your answers. A. Proteins are so remarkably diverse because each is made from a unique mixture of amino acids that are linked in random order. B. Lipid bilayers are macromolecules that are made up mostly of phospholipid subunits. C. Nucleic acids contain sugar groups. D. Many amino acids have hydrophobic side chains. E. The hydrophobic tails of phospholipid molecules are repelled from water. F. DNA contains the four different bases A, G, U, and C.</p><p>2. http://www.bio.unc.edu/courses/2004spring/biol052-006/ch02final.pdf 3. http://www.science.uwaterloo.ca/~cchieh/cact/applychem/waterbio.html</p><p>4. Vuori, K., Integrin signaling: Tyrosine phosphorylation events in focal adhesions. Journal of Membrane Biology, 1998. 26(3): p. 191-199.</p>
Details
-
File Typepdf
-
Upload Time-
-
Content LanguagesEnglish
-
Upload UserAnonymous/Not logged-in
-
File Pages10 Page
-
File Size-