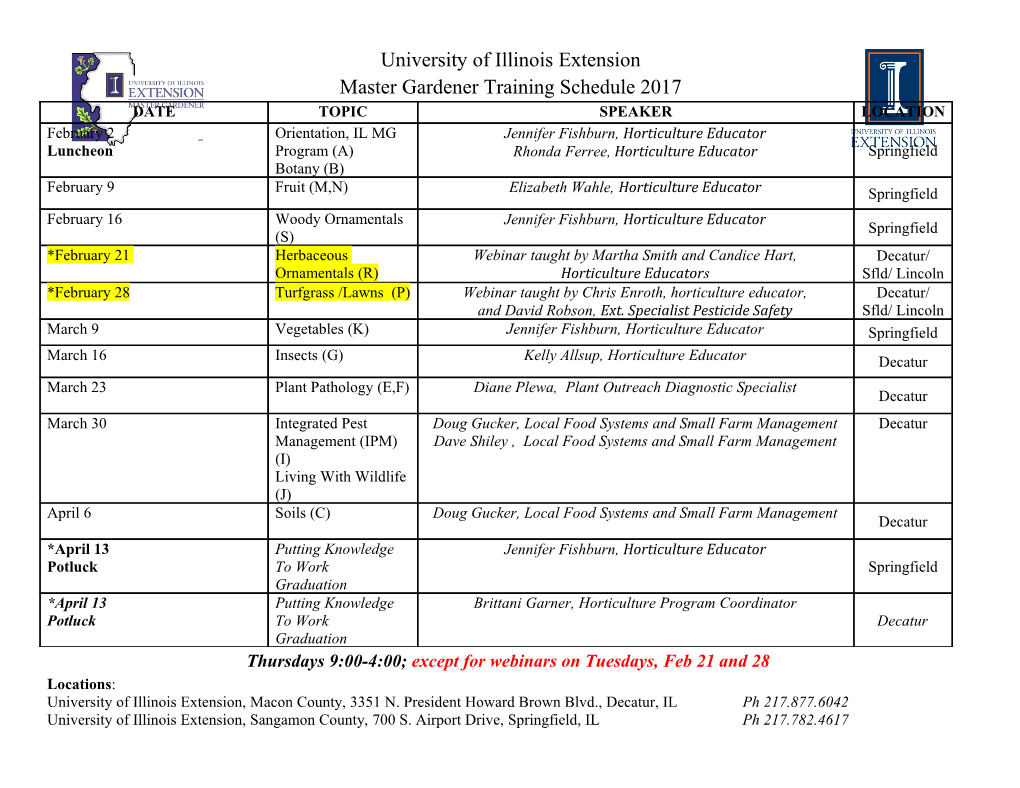
<p> APPENDICES TO BODY OF REPORT OD2010</p><p>Because this work was carried out at three different institutions, different abbreviations have been used for some of the different disinfectants and for the bacterial mutants derived from growth with the disinfectants.</p><p>The five biocides used were:-</p><p> A compound disinfectant containing a mix of Quaternary ammonium compounds, formaldehyde and glutaraldehyde. The abbreviations used for this disinfectant are QACFG, SK and ABD (aldehyde based disinfectant). An oxidising compound based disinfectant with the abbreviations OXC and VS. A tar oil phenol disinfectant with the abbreviations TOP and FFS. A dairy sterilizing disinfectant which comprised a quaternary ammonium biocide, non-ionic surfactant and excipients. The abbreviations used this disinfectant are DSD. Triclosan has the abbreviation T or TRIC.</p><p>A.1</p><p>Table 1. Strains used in this study to select mutants and control strains.</p><p>Designation Description Reference Parent strains L354 SL1344 20 L108 SL1344 (tolC::aph) 21</p><p>L357 DT104 21</p><p>L358 DT104 (penta resistant, GyrA Phe83) 21</p><p>L378 Ciprofloxacin resistant field isolate, GyrA Phe83 This study</p><p>L643 SL1344 (acrB::aph) 14</p><p>L696 SL1344 (GyrA Asp87) 10</p><p>L699 SL1344 (cyclohexane resistant) 23</p><p>Control strains AG100 E. coli K12 24</p><p>AG102 AG100 (cyclohexane tolerant, ∆marR) 24 Table 2. Susceptibility of mutants to antibiotics and disinfectants and times for a 5-log reduction in viable numbers.</p><p>Antibiotic susceptibility Disinfectant susceptibility Strain Selective MIC (µg/ml) Time (min) for 5-log kill MIC (%, v/v) agent Cip Chl Tet EtBr AF Kan OXC QACFG DSD TOP OXC QAC DSD TOP (0.6%, (0.025%, (0.2%, (0.075%, FG v/v) v/v) v/v) v/v) L357 0.015 2 1 512 128 2 14.5 +/- 0.5 0.4 0.02 0.1 0.2 5 TOP1 TOP 0.015 4 1 1024 128 2 10.6 +/- 0.3 0.2 TOP1 TOP 0.015 4 1 1024 128 8 6.2 +/- 0.3 0.2 L378 4 >256 256 2048 256 2 38 +/- 3.2 0.4 0.02 1.6 0.2 5 DSD1 DSD 4 >256 128 1024 256 2 34 +/- 2.1 >3.2 L108 <0.015 1 0.5 16 8 >32 15 +/- 0.8 0.4 0.00 <0.003 0.025 6 DSD2 DSD <0.015 1 0.5 16 8 >32 25 +/- 1.2 0.12 L358 0.5 >256 128 1024 256 2 12.1 +/- 0.2 0.8 0.02 0.8 0.2 5 QACF QACFG 2 >256 128 1024 256 2 13.5 +/- 0.7 0.02 G1 5 QACF QACFG 2 >256 256 1024 256 2 10.2 +/- 0.5 0.02 G2 5 QACF QACFG 2 >256 256 1024 256 2 14.2 +/- 0.7 0.02 G3 5 L354 0.015 4 1 1024 128 2 8.2 +/- 0.4 0.02 0.1 0.2 0.8 5 OXC1 OXC 0.015 4 0.5 1024 128 2 13 +/- 2.1 0.8 OXC2 OXC 0.015 8 0.5 1024 128 2 11.5 +/- 0.8 1</p><p>Values in bold indicate changes in tolerance to antibiotics or disinfectants of mutants compared to their respective parent strains. Cip = ciprofloxacin, Chl = chloramphenicol, Tet = tetracycline, EtBr = ethidium bromide, AF = acriflavine, Kan = kanamycin, OXC = oxidizing compound, QACFG = Quaternary ammonium compound + aldehyde + formaldehyde farm disinfectant, DSD = dairy sanitizer disinfectant, TOP = Tar oil Phenol. +/- values indicate standard deviation from the mean. OXC1, TOP1 etc are mutants derived from growth with specific compounds Table 3. Summary of protein changes after disinfectant exposure.</p><p>Exposure Number of proteins (mean + s.d.) Control Treated Control Treatment Common to Increased Decreased only only treatment and expressi expressio control on n (P<0.05) (P<0.05) 0.04% TOP 437 429 79 70 360 12 23 (696 + 129) (682 + 69)</p><p>0.15 w/v OXC 596 511 173 88 424 32 67 (927 + 91) (861 + 45)</p><p>OXC = oxidizing compound, TOP = Tar oil phenol disinfectant. Table 4. Proteins with statistically significantly altered expression after treatment with TOP</p><p>Group Description Protein Ratio Efflux RND family, multidrug efflux pump AcrB 2.8** Multidrug efflux pump EmrA 2.7** Membrane fusion protein of AcrAB-TolC AcrA 1.9** Outer membrane efflux channel TolC 1.8*** OmpF assembly AsmA 0.5* ATP synthesis Membrane-bound ATP synthase, F1 sector, beta-subunit AtpD 0.9* Membrane-bound ATP synthase, F0 sector, subunit b AtpF 0.8* Chemotaxis Methyl-accepting chemotaxis protein I TsR 0.4** Methyl-accepting transmembrane citrate/phenol chemoreceptor TcP 0.3*** Methyl accepting chemotaxis protein II CheM 0.2*** Hydrogenase/dehydrogenase Pyruvate dehydrogenase, dihydrolipoyltransacetylase component AceF 2.2** Pyruvate dehydrogenase, decarboxylase component AceE 2.0*** Putative hydrogenase, membrane component OmpA 1.3* 2-oxoglutarate dehydrogenase SucA 0.5* Plasma membrane proline dehydrogenase/ pyrroline-5-carboxylate dehydrogenase PutA 0.3** Miscellaneous Sigma D (sigma 70) factor of RNA polymerase RpoD 2.5* Alkyl hydroperoxide reductase AhpC 2.2** Fructose-bisphosphatase Fbp 1.7* 50S ribosomal subunit protein L6 RplF 1.6* Pyruvate kinase I PykF 1.4* Transcriptional activator of ntrl gene OsmE 0.8* DNA-binding protein HU-alpha HupA 0.7* Succinyl-coa synthetase, beta subunit SucC 0.7*** Part of modulator for protease specific for ftsh phage lambda cii repressor HflC 0.6** Glycoprotein/polysaccharide metabolism YbaY 0.6* ATP-dependent protease, Hsp 100 ClpB 0.6* Phosphoenolpyruvate carboxykinase PckA 0.5* Periplasmic L-asparaginase II AnsB 0.5* Glycerophosphodiester phosphodiesterase GlpQ 0.5* Mannose-specific enzyme IID ManZ 0.4* Putative outer membrane lipoprotein STM1607 0.4* Glutathione oxidoreductase Gor 0.4* Stringent starvation protein A, transcriptional regulator SspA 0.4** Aspartate ammonia-lyase (aspartase) AspA 0.4* Scaffolding protein for murein-synthesizing holoenzyme MipA 0.4*</p><p>Ratio indicates protein expression after disinfectant treatment divided by expression in untreated control. * = P <0.05, ** = P <0.01, *** = P <0.001. TOP = Tar oil phenol disinfectant. Table 5. Ten most statistically significantly over-expressed and repressed proteins after treatment with OXC</p><p>Description Protein Ratio 30S ribosomal subunit protein S3 RpsC 8.0*** uptake of enterochelin; tonB-dependent uptake of B colicins ExbB 6.5*** membrane-bound lytic murein transglycosylase A MltA 3.8*** subunit of cysteine synthase A and O-acetylserine sulfhydrolase A CysK 3.6*** ABC superfamily (atp&memb), cytochrome-related transporter CydC 3.5** periplasmic serine protease Do, heat shock protein HtrA 3.5** glucose dehydrogenase Gcd 3.3* outer membrane protein receptor / transporter for ferrichrome, colicin M, and phages T1, T5, and phi80 FhuA 3.3*** putative outer membrane lipoprotein YiaD 2.6** putative helicase RhlB 2.4* phosphoenolpyruvate synthase Pps 0.2* glycine cleavage complex protein P, glycine decarboxylase GcvP 0.2* putative methyl-accepting chemotaxis protein STM3138 0.1** methyl-accepting chemotaxis protein III, ribose and galactose sensor receptor Trg 0.1** L-lactate dehydrogenase LldD 0.1* periplasmic L-asparaginase II AnsB 0.1** phosphoenolpyruvate carboxykinase PckA 0.1*** fumarate reductase, anaerobic, flavoprotein subunit FrdA 0.1* flagellar biosynthesis; flagellin, filament structural protein FliC 0.1*** putative methyl-accepting chemotaxis protein STM3216 0.1***</p><p>Ratio indicates protein expression after disinfectant treatment divided by expression in untreated control. * = P <0.05, ** = P <0.01, *** = P <0.001 OXC = oxidizing compound A.2</p><p>FIGURE LEGENDS</p><p>Figure 1. Survival of SL1344 (open bars), OXC-1 (grey bars) and OXC-2 (black bars) after exposure to OXC (0.6%). </p><p>P>0.05 relative to parent strain at the same time point. OXC = oxidizing compound, OXC-1 and 2 a mutants derived from growth with OXC</p><p>Figure 2. Norfloxacin accumulation. </p><p>Open bars = - CCCP, hatched bars = + CCCP. * = P>0.05 relative to parent strain at the same time point. QACFG = Quaternary ammonium compound + aldehyde + formaldehyde farm disinfectant. Figure 3. Growth of L108 and DSD-2 with and without exposure to DSD. </p><p>Each point is the average of 9 values, error bars are omitted for clarity. The arrow indicates the time at which DSD was added to each well. Grey symbols = L108, open symbols = DSD-2, triangles = no disinfectant, squares = 0.1% DSD, circles = 0.2% DSD. DSD = Dairy sanitizing disinfectant, DSD-1 is a mutant derived from growth with DSD. Figure 4 Colonisation and persistence of L700 (LoT), L701 (MeT), and L702 (HiT) in chicks for up to 27 day post inoculation.</p><p>The error bars represent +/- 1 standard deviation. * P<0.05, ** P<0.01 and *** P<0.001. Values in parentheses indicate the number of birds in which the had no detectable cfu following plating. A.3</p><p>1. Randall, L. P., S. W. Cooles, L. J. Piddock, and M. J. Woodward. 2004. Effect of triclosan or a phenolic farm disinfectant upon the selection of antibiotic resistant Salmonella enterica. J. Antimicrob. Chemother. 54: 621-627. 2. Randall, L. P., C. S. Clouting, K. O. Gradel, F. A. Clifton-Hadley, R. D. Davies, and M. J. Woodward. 2005. Farm disinfectants select for cyclohexane resistance, a marker of multiple antibiotic resistance, in Escherichia coli. J. Appl. Microbiol. 98: 556-563. 3. Alekshun, M. N., and S. B. Levy. 1997. Regulation of chromosomally meditated multiple antibiotic resistance: the mar regulon. Antimicrob. Agents Chemother. 41: 2067-2075. 4. Randall, L. P., S. W. Cooles, A. R. Sayers, and M. J. Woodward. 2001. Cyclohexane resistance in Salmonella of different serovars is associated with increased resistance to multiple antibiotics, disinfectants and dyes. J. Med. Microbiol. 50: 1-6. 5. Randall, L. P., and M. J. Woodward. 2002. The multiple antibiotic resistance (mar) locus and its significance. Res. Vet. Sci. 72: 87-93. 6. Russell, A. D. 2000. Do biocides select for antibiotic resistance? J. Pharm. Pharmacol. 52: 227-233. 7. Russell, A. D. 2002. Introduction of biocides into clinical practice and the impact on antibiotic-resistant bacteria. J. App. Microbiol. 92: S121-S135 8. Jones, R. D., H. B. Jampani, J. L. Newman, and A. S. Lee. 2000. Triclosan: a review of effectiveness and safety in health care settings. Am. J. Inf. Cont. 28: 184-196. 9. Piddock, L. J. 2006 Multidrug-resistance efflux pumps - not just for resistance. Nat. Rev. Microbiol. 4: 629-36. 10. Ricci, V., P. Tzakas, A. M. Buckley, and L. J. Piddock. 2006. Ciprofloxacin- resistant Salmonella enterica serovar Typhimurium strains are difficult to select in the absence of AcrB and TolC. Antimicrob. Agents. Chemother. 50: 38-42. 11. Mazzola, P.G., T. C. Penna, and A. M. Martins. 2003. Determination of decimal reduction time (D value) of chemical agents used in hospitals for disinfection purposes. BMC Infect. Dis. 17: 3-24. 12. Andrews, J. M. 2001. Determination of minimum inhibitory concentrations. J. Antimicrob. Chemother. 48: S1,5-16. 13. Everett, M. J., Y. F. Jin, V. Ricci, and L. J. Piddock. 1996. Contributions of individual mechanisms to fluoroquinolone resistance in 36 Escherichia coli strains isolated from humans and animals. Antimicrob. Agents Chemother. 40: 2380-6. 14. Eaves, D. J., V. Ricci, and L. J. Piddock. 2004. Expression of acrB, acrF, acrD, marA, and soxS in Salmonella enterica serovar Typhimurium: role in multiple antibiotic resistance. Antimicrob. Agents Chemother. 48: 1145-50. 15. Mortimer, P.G., and L. J. Piddock. 1991. A comparison of methods used for measuring the accumulation of quinolones by Enterobacteriaceae, Pseudomonas aeruginosa and Staphylococcus aureus. J. Antimicrob. Chemother. 28: 639-53. 16. Coldham, N.G., and M. J. Woodward. 2004. Characterisation of the Salmonella Typhimurium proteome by semi-automated two-dimensional HPLC-mass spectrometry: detection of proteins implicated in multiple antibiotic resistance. J. Proteome Res. 3:595-603. 17. Coldham, N.G., L. P. Randall., L. J. Piddock, and M. J. Woodward. 2006. Effect of fluoroquinolone exposure on the proteome of Salmonella enterica serovar Typhimurium. J. Antimicrob. Chemother. 58: 1145-1153. 18. Liu, H., R. G. Sadygov, and J. R. Yates. 2004. A model for random sampling and estimation of relative protein abundance in shotgun proteomics. Anal Chem. 76: 4193-201. 19. Gao, J., M. S. Friedrichs, and A. R. Dongre. 2004. Guidelines for the routine application of the peptide hits technique. J. Am. Soc. Mass. Spectrom. 16: 1231-8. 20. Randall, L. P., and M. J. Woodward. 2001. Role of the mar locus in virulence of Salmonella enterica serovar Typhimurium DT104 in chickens. J. Med. Microbiol. 50: 770-779. 21. Wray, C., and W. J. Sojka. 1978. Res. Vet. Sci. 25:139-43. 22. Buckley, A. M., M. A. Webber, S. W. Cooles, L. P. Randall, R. M. La Ragione, M. J. Woodward, and Piddock L.J. 2006. The AcrAB-TolC efflux system of Salmonella enterica serovar Typhimurium plays a role in pathogenesis. Cell. Microbiol. 8: 847-56. 23. Webber, M. A., A. M. Buckley, L. P. Randall, M. J. Woodward, and L. J. Piddock. 2006. Over-expression of marA, soxS and acrB in veterinary isolates of Salmonella enterica rarely correlates with cyclohexane tolerance. J. Antimicrob. Chemother. 57: 673-9. 24. George, A. M., and S. B. Levy. 1983. Amplifiable resistance to tetracycline, chloramphenicol, and other antibiotics in Escherichia coli: involvement of a non- plasmid-determined efflux of tetracycline. J. Bacteriol. 155: 531-40. B.1 TABLE 1. MICs of populations selected following sequential growth experiments a</p><p>Population MICs of selected antimicrobial agents CIP CHL TET KAN AMP TRIC OXC TOP QACFG DSD OXC 7d <0.03 8-16 2-4 2-4 2-8 0.25 0.20 0.40 <0.05 0.40 TOP 7d <0.03 8 2 2-4 2 0.12 0.20 0.40 <0.05 0.2-0.4 QACFG 7d <0.03 16 2-4 2-4 16 1.00 0.20 0.40 <0.05 0.40 16TRIC 16d <0.03 16 2-4 4 8-16 64.00 0.20 0.40 <0.05 0.2-0.4 LB 7d <0.03 8 2 2 2-4 0.25 0.20 0.40 <0.05 0.2-0.4 LB 1d <0.03 8 2 2 2-4 0.06 0.20 0.2-0.4 <0.05 0.20 0.12- L696 OXC 7d 8 1-2 2-4 2 0.06 0.20 0.40 <0.05 0.40 0.25 L696 TOP 7d 0.12 8 1-2 2-4 2-4 0.25 0.20 0.40 <0.05 0.2-0.4 L696 QACFG 7d 0.25 16 2-8 2 16 0.25 0.20 0.40 <0.05 0.20 L696 16TRIC 16d 0.25 16 2-4 2 8-16 >128 0.20 0.40 <0.05 0.20 0.06- L696 LB 7d 8 2-4 2-4 2-4 0.25 0.20 0.40 <0.05 0.20 0.12 0.12- L696 LB 1d 8 2-4 2 2 0.06 0.20 0.40 <0.05 0.2-0.4 0.25 a MICs of ciprofloxacin (CIP), chloramphenicol (CHL), tetracycline (TET), kanamycin (KAN) ampicillin (AMP) and triclosan (TRIC) are presented in μg ml-1, while those of OXC, TOP, QACFG and DSD are presented in %. Populations are named as follows (parent strain, if not mentioned it is the wild type) (disinfectant and concentration if it is not close to the MIC, from which population was isolated) (days of sequential growth in disinfectant). For example, population OXC 7d is derived from wild type, was isolated from LB broth containing OXC and was sequentially grown for 7 days etc. </p><p>TABLE 2. MICs of isolated strains a</p><p>Isolate MICs of selected antimicrobial agents CIP CHL TET KAN AMP TRIC OXC TOP QACFG DSD OXC R1 0.06 32 2 1 8 0.25 0.20 0.40 <0.05 0.40 OXC R2 <0.03 8 2 2 2 0.12 0.20 0.40 <0.05 0.20 QACFG R1 <0.03 8 2 1 8 0.25 0.20 0.20 <0.05 0.20 QACFG R2 <0.03 32 16 2 8 0.25 0.20 <0.05 <0.05 0.1 TOP R1 <0.03 8 2 2 4 0.12 0.20 0.40 <0.05 0.2 TOP R2 <0.03 16 16 2 4 0.25 0.20 0.10 <0.05 0.10 TRIC R1 <0.03 8-16 2-4 2 8 64 0.20 0.40 <0.05 0.40 TRIC R2 <0.03 16 2-4 2 8 64 0.20 0.40 <0.05 0.2-0.4 WT <0.03 8 2 2 2-4 0.06 0.20 0.2-0.4 <0.05 0.20 a MICs of ciprofloxacin (CIP), chloramphenicol (CHL), tetracycline (TET), kanamycin (KAN) ampicillin (AMP) and triclosan (TRIC) are presented in μg ml-1, while those of OXC, TOP, QACFG and DSD are presented in %. B.2</p><p>FIG. 1. Frequency of variants tolerant to 0.05 μg ml-1 triclosan (1A), 400 μg ml-1 acriflavine (1B), 1.2 μg ml-1 tetracycline (1C), 6 μg ml-1 chloramphenicol (1D) and 2 μg ml-1 ampicillin (1E) within L354 populations obtained following overnight growth in LB broth (LB1d) or sequential growth in LB (LB 7d) without biocide or supplemented with 0.2% VS (VS 7d), 0.025% FFS (FFS 7d), 0.006% SK (SK 7d) or 0.06 μg ml-1 triclosan (16T 16d). Sequential growth at rising concentrations, as described in text was for seven days in all cases, except with Triclosan, which was for 16 days.</p><p>FIG. 2. mRNA levels of transcripts of acrB gene in logarithmic phase cultures of OXCR1, QACFG R2, TOP R2, TRIC R1 and TRIC R2. 80000 )</p><p> s 70000 t i n u</p><p>60000 y r a</p><p> r 50000 t i b r</p><p> a 40000 (</p><p> e c</p><p> n 30000 a b r</p><p> o 20000 s b</p><p>A 10000</p><p>0</p><p>Strain See top of appendices for disinfectant abbreviations OXCR1, QACFGR2 etc are mutants from growth with respective disinfectants. B.3</p><p>1. Andrews, J.M. (2001) Determination of minimum inhibitory concentrations. Journal of Antimicrobial Chemotherapy 48: Suppl. S1, 5–16. 2. Baucheron, S., Tyler, S., Boyd, D., Mulvey, M.R., Chaslus-Dancla, E., and Cloeckaert, A. (2004) AcrAB-TolC Directs Efflux-Mediated Multidrug Resistance in Salmonella enterica Serovar Typhimurium DT104. Antimicrobial Agents and Chemotherapy 48(10): 3729-3735. 3. Buckley, A.M., Webber, M.A., Cooles, S., Randall, L.P., La Ragione, R.M., Woodward, M.J. and Piddock, L.J.V. (2006) The AcrAB–TolC efflux system of Salmonella enterica serovar Typhimurium plays a role in pathogenesis. Cellular Microbiology 8(5): 847–856. 4. Eaves, D.J., Ricci, V., and Piddock L.J.V. (2004) Expression of acrB, acrF, acrD, marA, and soxS in Salmonella enterica serovar Typhimurium: role in multiple antibiotic resistance. Antimicrobial Agents and Chemotherapy 48:1145- 1150. 5. Fraise, A.P. (2002) Biocide abuse and antimicrobial resistance—a cause for concern? Journal of Antimicrobial Chemotherapy 49: 11-12. 6. Giraud, E., Cloeckaert, A., Kerboeuf, D. and Chaslus-Dancla, E. (2000) Evidence for Active Efflux as the Primary Mechanism of Resistance to Ciprofloxacin in Salmonella enterica Serovar Typhimurium. Antimicrobial Agents and Chemotherapy 44: 1223-1228. 7. Heath, R.J. and Rock, C.O. (2000) A triclosan-resistant bacterial enzyme. Nature 406: 145–6. 8. Heath, R.J., Rubin, J.R., Holland, D.R., Zhang, E., Snow, M.E. and Rock, C.O. (1999) Mechanism of triclosan inhibition of bacterial fatty acid synthesis. Journal of Biological Chemistry 274: 11110–11114. 9. Levy, S.B. (1992) Active efflux mechanisms for antimicrobial resistance. Antimicrobial Agents and Chemotherapy 36:695-703. 10. Levy, S.B. (2002) Active efflux, a common mechanism for biocide and antibiotic resistance. Journal of Applied Microbiology 92:65S-71S. 11. Mcdonnell, G. and Russell, A.D. (1999) Antiseptics and Disinfectants: Activity, Action, and Resistance. Clinical Microbiology Reviews 12: 147-179. 12. McMurry, L.M., Oethinger, M. and Levy, S.B. (1998). a Triclosan targets lipid synthesis. Nature 394: 531–2. 13. McMurry, L.M., Oethinger, M. and Levy, S.B. (1998). b Overexpression of marA, soxS, or acrAB produces resistance to triclosan in laboratory and clinical strains of Escherichia coli. FEMS Microbiology Letters 166: 305–9. 14. Moken, M.C., McMurry, L.M. and Levy, S.B. (1997) Selection of multiple- antibiotic-resistant (Mar) mutants of Escherichia coli by using the disinfectant pine oil: Roles of the mar and acrAB loci. Antimicrobial Agents and Chemotherapy 41(12) 2770-2772. 15. Nishino, K., Latifi, T. and Groisman, E.A.. (2006) Virulence and drug resistance roles of multidrug efflux systems of Salmonella enterica serovar Typhimurium. Molecular Microbiology 59: 126-141. 16. Okusu, H., Ma, D. and Nikaido, H. (1996) AcrAB efflux pump plays a major role in the antibiotic resistance phenotype of Escherichia coli multiple-antibiotic- resistance (mar) mutants. Journal of Bacteriology 178: 306–308. 17. Piddock, L.J.V. (2002) Fluoroquinolone resistance in Salmonella serovars isolated from humans and food animals. FEMS Microbiology Reviews 26:3-16. 18. Piddock LJ, White DG, Gensberg K, Pumbwe L, Griggs DJ. 2000. Evidence for an efflux pump mediating multiple antibiotic resistance in Salmonella enterica serovar Typhimurium. Antimicrob Agents Chemother. 44:3118-21. 19. Poole, K. (2002) Mechanisms of bacterial biocide and antibiotic resistance. Symp.Ser. Soc. Apl. Microbiol. 31:55S-64S. 20. Randall, L.P., Cooles, S.W., Sayers, R. and Woodward, M.J. (2001) Association between cyclohexane resistance in Salmonella of different serovars and increased resistance to multiple antibiotics, disinfectants and dyes. Journal of Medical Microbiology 50:919-924. 21. Randall, L.P., Eaves, D.J., Cooles, S.W., Ricci, V., Buckley, A., Woodward, M.J. and Piddock, L.J.V. (2005) Fluoroquinolone treatment of experimental Salmonella enterica serovar Typhimurium DT104 infections in chickens selects for both gyrA mutations and changes in efflux pump gene expression. Journal of Antimicrobial Chemotherapy 56: 297–306. 22. Randall, L.P., S. W. Cooles, L.J.V. Piddock and M. J. Woodward. (2004) Effect of triclosan or a phenolic farm disinfectant on the selection of antibiotic-resistant Salmonella enterica. Journal of Antimicrobial Chemotherapy 54:621-627. 23. Russell, A.D. (2000) Do Biocides Select for Antibiotic Resistance? Journal of Pharmacy and Pharmacology. 52: 227-233. 24. Russell, A.D. (2003) Biocide use and antibiotic resistance: the relevance of laboratory findings to clinical and environmental situations. The Lancet Infectious Diseases. 3 :794-803. 25. Thanassi, D.G., Cheng, L. W. and Nikaido, H. (1997) Active efflux of bile salts by Escherichia coli. Journal of Bacteriology 179: 2512-2518. 26. van Bambekel, F., Glupczynski, Y., Plésiat, P., Pechère, J.C. and Tulkens, P.M. (2003) Antibiotic efflux pumps in prokaryotic cells: occurrence, impact on resistance and strategies for the future of antimicrobial therapy. Journal of Antimicrobial Chemotherapy 51: 1055–1065. 27. Visalli, M.A., Murphy, E., Projan, S.J. and Bradford, P.A. (2003) AcrAB Multidrug Efflux Pump Is Associated with Reduced Levels of Susceptibility to Tigecycline (GAR-936) in Proteus mirabilis. Antimicrobial Agents and Chemotherapy 47(2): 665-669. 28. Wray, C. and W.J., Sojka. (1978) Experimental Salmonella Typhimurium infection in calves. Res. Vet. Sci. 25:139-43. C.1 Table 1. S. Typhimurium parent strains used in this study</p><p>Strain Comment Origin/Reference L354 SL1344 Wray and Soijka 1978 L643 SL1344 acrB::aph Eaves et al., 2004 L108 SL1344 tolC::aph Eaves et al., 2004 L696 SL1344 GyrA Asp 87 Ricci et al., 2006 L699 SL1344 cyclohexane tolerant Webber et al., 2006 L357 DT104 fully antibiotic sensitive strain Randall et al., 2005 L358 DT104 penta resistant veterinary isolate, Randall et al., 2005 </p><p>GyrA Phe 83 L359 DT104 multiply antibiotic resistant Randall et al., 2005 </p><p> derivative of L357 L378 S. Typhimurium veterinary isolate, GyrA Veterinary laboratories agency, </p><p>Phe 83 U.K Table 2. Mutation selection frequency and phenotype of selected triclosan resistant mutants.</p><p>Strain Parent/Mutant Origin Selection MIC (mg/L)a FabI frequency Tric Cip EtBr L354 Parent SL1344 N/A 0.06 0.03 1024 WT L707 Mutant Low L354 7.0 X 10-9 4 (32) 0.03 1024 WT</p><p>L709 Mutant Low L354 7.0 X 10-9 4 (32) 0.03 1024 G93S L378 Parent CipR field N/A 0.25 1 1024 WT isolate L691 Mutant Low L378 5.8 X 10-8 4 (16) 1 1024 WT L690 Mutant High L378 6.0 X 10-8 >128 1 1024 G93V L696 Parent CipR mutant N/A 0.25 0.25 1024 WT of L354 L700 Mutant Low L696 9.6 X 10-8 4 (16)b 0.25 1024 WT L701 Mutant Int L696 9.2 X 10-8 32 0.25 1024 G93V (128) L702 Mutant High L696 9.4 X 10-8 128 0.25 1024 G93V (512) L699 Parent Cyclohexane N/A 0.5 0.12 1024 WT tolerant L703 Mutant Int L699 8.6 X 10-7 32 (64) 0.12 1024 G93V L705 Mutant High L699 9.0 X 10-7 64 0.12 1024 WT (128) a Tric = triclosan, Cip = ciprofloxacin, EtBr = ethidium bromide. b Numbers in brackets are fold increase in triclosan resistance compared to parent. Table 3. Effect of gene disruptions and pBAD-fabI over-expression on triclosan susceptibility.</p><p>MIC triclosan (g/ml) Strain Wild-type tolC::ap acrB::ap marA::ap ramA::ap +pBADfabI</p><p> h h h h a SL1344 0.06 0.015 (4) 0.06 (0) 0.06 (0) 0.06 (0) 0.06 (0) L696 0.25 0.06 (4) 0.25 (0) 0.25 (0) 0.25 (0) 1 (4)</p><p>L700 4 0.12 (33) 2 (2) 2 (2) 2 (2) 8 (2) L701 32 8 (4) 1 (32) 16 (2) 2 (16) 32 (0) L702 128 0.5 (256) 4 (32) 2 (64) 4 (32) 16 (8) a Experiments performed in the presence of 0.002% arabinose. Numbers in brackets refer to fold change in triclosan susceptibility relative to parent. Table 4. Proteins with significantly altered expression in all three triclosan resistant mutants.</p><p>Expression ratioa Function Protein Description L700 (Lot) L701 (Met) L702 (Hit) Average Generation of ArcA Putative arginine deiminase (arcA) 7.0 3.0 3.3 4.4 pyruvate/fatty acid GcvP Glycine cleavage complex protein P 2.2 5.6 4.6 4.1 PpS Phosphoenolpyruvate synthase 1.5 3.7 2.8 2.7 FadB 3-hydroxyacyl-coA dehydrogenase 3.0 2.0 2.0 2.3 MaeB Malate transferase 2.1 2.3 1.7 2.0 GltA Citrate synthase 1.3 1.7 2.3 1.8 GapA Glyceraldehyde-3-phosphate dehydrogenase A 1.3 1.7 1.9 1.6 GlpK Glycerol kinase 1.5 1.7 1.5 1.6 MdH Malate dehydrogenase 1.4 1.5 1.8 1.6 Stress HtrA Periplasmic serine protease, heat shock protein 2.3 3.6 6.2 4.0 response/regulation HNS DNA-binding protein, pleiotropic regulator 2.4 2.0 2.3 2.2 CspC Cold shock protein, putative regulator 1.6 1.8 1.5 1.6 Porphyrin HemL Glutamate-1-semialdehyde aminotransferase 3.3 3.0 4.0 3.4 biosynthesis HemX Uroporphyrinogen methylase 1.4 2.1 2.3 1.9 Miscellaneous AhpC Alkyl hydroperoxide reductase 1.6 2.5 2.3 2.1 TktA Transketolase 1 isozyme 1.4 1.6 1.8 1.6 YbhC Putative pectinesterase 1.5 1.5 1.8 1.6 RpsA 30S ribosomal subunit protein S1 1.5 1.7 1.5 1.6 TufA Protein chain elongation factor 1.3 1.6 1.6 1.5 WzzB Regulator of length of O-antigen of LPS 0.5 1.6 1.9 1.3 LamB Phage lambda receptor protein 0.5 1.5 0.5 0.8 STM3152 Putative methyl-accepting chemotaxis protein 0.3 0.6 0.3 0.4 NupC Nucleoside transport 0.5 0.5 0.2 0.4 YjiJ Putative carbon starvation protein 0.5 0.3 0.4 0.4 CheA Sensory histidine protein kinase 0.2 0.6 0.2 0.3 a Expression ratio relative to L696. Table 5. Selected proteins with differential expression in L700 (LoT) compared to L696.</p><p>Function Protein Description Expression ratioa Fatty acid FabI Enoyl-[acyl-carrier-protein] reductase (NADH) 3.8 biosynthesis/metabolism FabB 3-oxoacyl-[acyl-carrier-protein] synthase I 2.2 FadB 3-hydroxyacyl-coA dehydrogenase 3.0 Global regulation HNS DNA-binding protein; pleiotropic regulator 2.0 Cold shock CspE RNA chaperone, negative regulator of cspA transcription 2.1 CspC Cold shock protein, putative regulator 1.6 Amino acid metabolism STM4467 Putative arginine deiminase (arcA) 7.0 GcvP Glycine cleavage complex protein P, glycine decarboxylase 2.2 Motility/chemotaxis FliC Flagellar biosynthesis; flagellin, filament structural protein 0.3 STM3216 Putative methyl-accepting chemotaxis protein 0.3 Tsr Methyl-accepting chemotaxis protein I, serine sensor receptor 0.2 CheA Sensory histidine protein kinase 0.2 STM3152 Putative methyl-accepting chemotaxis protein 0.2 CheM Methyl accepting chemotaxis protein II 0.2 aRatio indicates expression in L700 (LoT) relative to L696. Table 6. Selected proteins with significantly altered expression in both L701 (MeT) and L702 (HiT) compared to L696.</p><p>Function Protein Description Expression ratioa L701 L702 (MeT) (HiT) Amino acid biosynthesis/metabolism Gnd Gluconate-6-phosphate dehydrogenase 5.3 5.6 GcvP Glycine cleavage complex protein P 5.6 4.6 HemL Glutamate-1-semialdehyde aminotransferase 3.0 4.0 MetK Methionine adenosyltransferase 1 3.4 3.8 STM4467 Putative arginine deiminase 3.0 3.3 Miscellaneous YbbN Paral putative thioredoxin protein 3.0 4.0 MalK ABC superfamily maltose transport protein 0.6 0.6 HupB DNA-binding protein 0.6 0.6 LamB Phage lambda receptor protein 1.5 0.5 PtsG Sugar Specific PTS family 0.6 0.5 FadL Transport of long-chain fatty acids 0.5 0.4 NupC NUP family, nucleoside transport 0.5 0.2 Motlility/chemotaxis STM3152 Putative methyl-accepting chemotaxis protein 0.6 0.3 CheA Sensory histitine protein kinase 0.6 0.2 STM3216 Putative methyl-accepting chemotaxis protein 0.4 0.1 Stress response HtrA Periplasmic serine protease Do, heat shock protein 3.6 6.2 UspA Universal stress protein A 3.6 4.4 IbpB Small heat shock protein 2.1 3.5 MopA Chaperone Hsp60 2.8 3.4 YjiY Putative carbon starvation protein 0.3 0.4 aRatio indicates expression relative to L696. C.2</p><p>Figure 1. Accumulation of norfloxacin or Hoechst 33342 after incubation for 5 min. </p><p>Panel A shows accumulation of norfloxacin (ng of norfloxacin per mg of dry cell weight), panel B shows accumulation of Hoechst 33342 (fluorescence, excitation 350nm, emission 461nm). Open boxes indicate accumulation in the absence of CCCP, shaded boxes indicate accumulation after addition of 100M CCCP. Asterixes indicate values significantly different to L696 (P < 0.05) Figure 2. Triclosan resistance network. Proteins with increased expression in all three triclosan resistant mutants studied are shown in bold. Figure 3. Growth kinetics of triclosan mutants in the presence and absence of triclosan. </p><p>Plain line SL1344, lines with crosses L696, lines with open circles L700, lines with open boxes L701, lines with open diamonds L702. For the sake of clarity error bars have been omitted. C.3 1. Allmyr, M., M, Adolfsson-Erici., M.S, McLachlan., G, Sandborgh-Englund. (2006). Triclosan in plasma and milk from Swedish nursing mothers and their exposure via personal care products. Sci Total Environ. 15:87-93. 2. Amblar, M and Arraiano, C.M (2005). A single mutation in Escherichia coli ribonuclease II inactivates the enzyme without affecting RNA binding. FEBS Journal 272:363-374.</p><p>3. Andrews, J. M. (2001). Determination of minimum inhibitory concentrations. J Antimicrob Chemother 48 Suppl 1:5-16.</p><p>4. Baucheron, S., H. Imberechts, E. Chaslus-Dancla, and A. Cloeckaert. (2002). The AcrB multidrug transporter plays a major role in high-level fluoroquinolone resistance in Salmonella enterica serovar typhimurium phage type DT204. Microb.Drug Resist. 8:281-289.</p><p>5. Baucheron, S., C. Mouline, K. Praud, E. Chaslus-Dancla, and A. Cloeckaert. (2005). TolC but not AcrB is essential for multidrug-resistant Salmonella enterica serotype Typhimurium colonization of chicks. J Antimicrob Chemother 55:707-712.</p><p>6. Buckley A. M, Webber M.A, Cooles S, Randall L.R, LaRagione R, Woodward M. J, Piddock L. J. V (2006). The AcrAB-TolC efflux system of Salmonella enterica serovar Typhimurium plays a role in pathogenesis. Cell Microbiol. 8:847-56</p><p>7. Carraminana, J. J., C. Rota, I. Agustin, and A. Herrera. (2004). High prevalence of multiple resistance to antibiotics in Salmonella serovars isolated from a poultry slaughterhouse in Spain. Vet.Microbiol. 104:133-139.</p><p>8. Chuanchuen, R., R. R. Karkhoff-Schweizer, and H. P. Schweizer. (2003). High- level triclosan resistance in Pseudomonas aeruginosa is solely a result of efflux. Am.J Infect.Control 31:124-127.</p><p>9. Coldham, N.G., and M.J. Woodward. (2004). Characterization of the Salmonella Typhimurium Proteome by semi-automated HPLC-mass spectrometry: detection of proteins implicated in multiple antibiotic resistance. J. Proteome Research 3:595-603.</p><p>10. Coldham, N.G, Randall, L.P, Piddock, L.J.V, Woodward, M.J. (2006) Effect of fluoroquinolone exposure on the proteome of Salmonella enterica serovar Typhimurium. J. Antimicrob. Chemother. 58:1145-1153.</p><p>11. Cutter, C. N. (1999). The effectiveness of triclosan-incorporated plastic against bacteria on beef surfaces. J Food Prot. 62:474-9.</p><p>12. Eaves, D. J., V. Ricci, and L. J. Piddock. (2004). Expression of acrB, acrF, acrD, marA, and soxS in Salmonella enterica serovar Typhimurium: role in multiple antibiotic resistance. Antimicrob Agents Chemother 48:1145-1150.</p><p>13. Eng J.K., A.L. McCormack, and J.R.Yates III. (1994). An approach to correlate tandem mass spectral data of peptides with amino acid sequence in a protein database. J. Am Soc Mass Spectrom. 5:976-989.</p><p>14. Fan F, Yan K, Wallis NG, Reed S, Moore TD, Rittenhouse SF, DeWolf WE Jr, Huang J, McDevitt D, Miller WH, Seefeld MA, Newlander KA, Jakas DR, Head MS, Payne DJ (2002). Defining and combating the mechanisms of triclosan resistance in clinical isolates of Staphylococcus aureus. Antimicrob Agents Chemother. 46:3343-7. 15. Fluit, A. C. (2005). Towards more virulent and antibiotic-resistant Salmonella? FEMS Immunol.Med.Microbiol. 43:1-11.</p><p>16. Gao J, Friedrichs M.S, Dongre A.R. (2004) Guidelines for the routine application of the peptide hits technique. J Am Soc Mass Spectrom 16: 1231-8.</p><p>17. Gyorgy, B, E Toth, E Tarcsa, A Falus, E.I. Buzas. (2006). Citrullination: a posttranslational modification in health and disease. Int J Biochem Cell Biol. 38:1662- 77.</p><p>18. Heath, R. J., N. Su., C. K. Murphy., C. O. Rock. (2000). The enoyl-[acyl-carrier- protein] reductases FabI and FabL from Bacillus subtilis. J Biol Chem. 275:40128-33.</p><p>19. Helms, M, P. Vastrup, P. Gerner-Smidt, K. Molbak. (2002). Excess mortality associated with antimicrobial drug-resistant Salmonella typhimurium. Emerg Infect Dis. 8:490-5. </p><p>20. Kolpin, DW, Furlong ET, Meyer MT, Thurman EM, Zaugg SD, Barber LB, Buxton HT. (2002). Pharmaceuticals, hormones, and other organic wastewater contaminants in U.S. streams, 1999-2000: a national reconnaissance. Environ Sci Technol. 15:1202-11.</p><p>21. Liu, H., R.G. Sadygov, and J.R.Yates III. (2004). A model for random sampling and estimation of relative protein abundance in shotgun proteomics. Anal. Chem 76:4193- 4201.</p><p>22. Medlin, J (1997). Germ warfare. Env. Health Perspec. 105:291-293. </p><p>23. McMurry, L. M., M. Oethinger, and S. B. Levy. (1998). Triclosan targets lipid synthesis. Nature 394:531-532.</p><p>24. McMurry, L. M., M. Oethinger, and S. B. Levy. (1998). Overexpression of marA, soxS, or acrAB produces resistance to triclosan in laboratory and clinical strains of Escherichia coli. FEMS Microbiol.Lett. 166:305-309.</p><p>25. Michael, G. B., P. Butaye, A. Cloeckaert, S. Schwarz. (2006). Genes and mutations conferring antimicrobial resistance in Salmonella: an update. Microbes Infect. 8:1898- 914. </p><p>26. Moretro, T., T, Sonerud., E, Mangelrod., S, Langsrud. (2006). Evaluation of the antibacterial effect of a triclosan-containing floor used in the food industry. J Food Prot. 69:627-33.</p><p>27. Mortimer P. G and Piddock L. J. V. (1991). A comparison of methods used for measuring the accumulation of quinolones by Enterobacteriaceae, Pseudomonas aeruginosa and Staphylococcus aureus. J Antimicrob Chemother. 28:639-53.</p><p>28. Oethinger M, W. V. Kern, A. S. Jellen-Ritter, L. M. McMurry, and S. B. Levy. (2000). Ineffectiveness of topoisomerase mutations in mediating clinically significant fluoroquinolone resistance in Escherichia coli in the absence of the AcrAB efflux pump. Antimicrob Agents Chemother. 44:10-3.</p><p>29. Piddock L.J.V. (2006). Multidrug-resistance efflux pumps - not just for resistance. Nat Rev Microbiol. 4:629-36</p><p>30. Randall, L. P., D. J. Eaves, S. W. Cooles, V. Ricci, A. Buckley, M. J. Woodward, and L. J. Piddock. (2005). Fluoroquinolone treatment of experimental Salmonella enterica serovar Typhimurium DT104 infections in chickens selects for both gyrA mutations and changes in efflux pump gene expression. J Antimicrob Chemother 56:297-306.</p><p>31. Ricci V, Tzakas P, Buckley A, Piddock LJ. (2006). Ciprofloxacin-resistant Salmonella enterica serovar Typhimurium strains are difficult to select in the absence of AcrB and TolC. Antimicrob Agents Chemother. 50:38-42. </p><p>32. Roberts, J. A., P. Cumberland, P. N. Sockett, J. Wheeler, L. C. Rodrigues, D. Sethi, and P. J. Roderick. (2003). The study of infectious intestinal disease in England: socio-economic impact. Epidemiol.Infect. 130:1-11.</p><p>33. Russell, A. D. (2004). Whither triclosan? J Antimicrob Chemother 53:693-695.</p><p>34. Singer, H., S, Muller., C, Tixier., L, Pillonel. (2002). Triclosan: occurrence and fate of a widely used biocide in the aquatic environment: field measurements in wastewater treatment plants, surface waters, and lake sediments. Environ Sci Technol. 1:4998-5004.</p><p>35. Sivaraman S, Zwahlen J, Bell AF, Hedstrom L and Tonge PJ (2003). Structure- activity studies of the inhibition of FabI, the enoyl reductase from Escherichia coli, by triclosan: kinetic analysis of mutant FabIs. Biochemistry. 42:4406-13.</p><p>36. Tabb, D.L., W.H. McDonald, and J.R.Yates. (2002). DTASelect and contrast: tools for assembling and comparing protein identifications from shotgun proteomics. J. Proteome Res. 1:21-26.</p><p>37. Turner AK, Nair S, Wain J. (2006). The acquisition of full fluoroquinolone resistance in Salmonella Typhi by accumulation of point mutations in the topoisomerase targets. J Antimicrob Chemother. 58:733-40.</p><p>38. Wang, R. F. & Kushner, S. (1991). Construction of versatile low-copy-number vectors for cloning, sequencing and gene expression in Escherichia coli. Gene 100:195–199.</p><p>39. Webber, M. A. and L. J. Piddock. (2003). The importance of efflux pumps in bacterial antibiotic resistance. J Antimicrob Chemother 51:9-11.</p><p>40. Webber M.A, A.M. Buckley, L.P. Randall, M.J. Woodward, and L.J. Piddock. (2006). Over-expression of marA, soxS and acrB in veterinary isolates of Salmonella enterica rarely correlates with cyclohexane tolerance. J Antimicrob Chemother. 57:673-9</p><p>41. Weill, F. X., R. Lailler, K. Praud, A. Kerouanton, L. Fabre, A. Brisabois, P. A. Grimont, and A. Cloeckaert. (2004). Emergence of extended-spectrum-beta- lactamase (CTX-M-9)-producing multiresistant strains of Salmonella enterica serotype Virchow in poultry and humans in France. J Clin.Microbiol. 42:5767-5773.</p><p>42. Wray, C. and W. J. Sojka. (1978). Experimental Salmonella typhimurium infection in calves. Res Vet.Sci. 25:139-143.</p>
Details
-
File Typepdf
-
Upload Time-
-
Content LanguagesEnglish
-
Upload UserAnonymous/Not logged-in
-
File Pages30 Page
-
File Size-