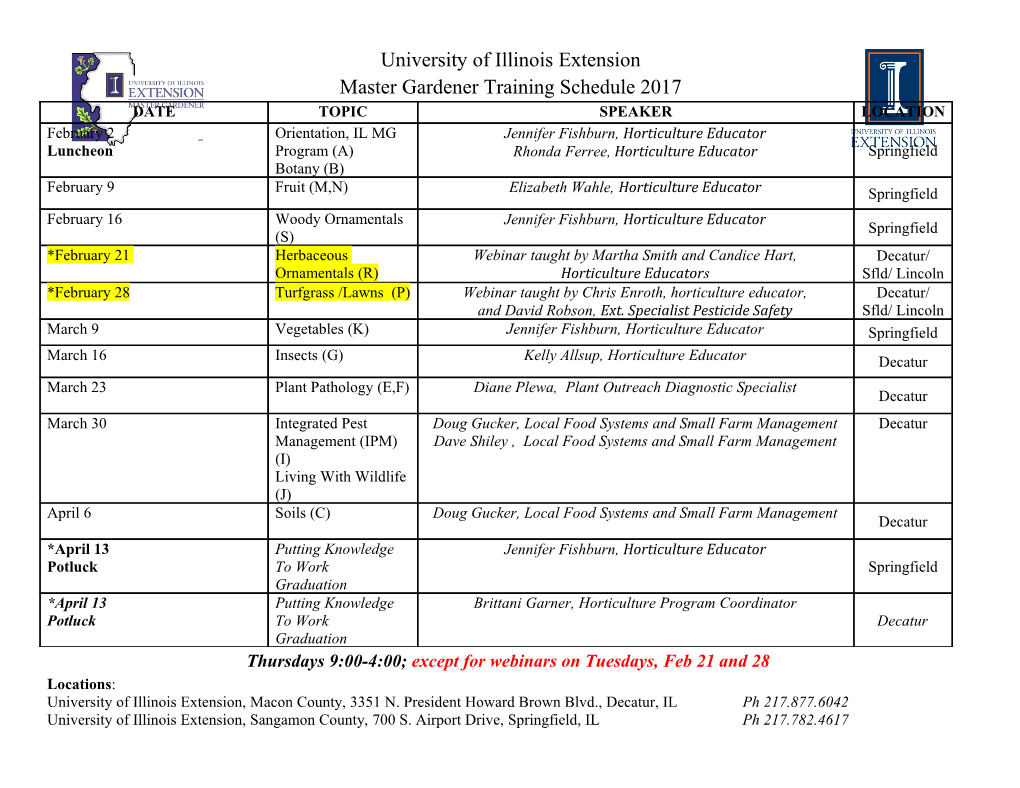
<p> 1SUPPLEMENTAL MATERIAL</p><p>2</p><p>3Text</p><p>4Characterization of pentapeptide insertion mutants</p><p>5 Cgs-region (1-418). The functional role of the extreme N-terminus of Cgs is not</p><p>6known although in silico analysis of the Cgs-region (1-418) predicted a possible protein-</p><p>7protein interaction module (2). In agreement with the motility phenotype, all insertions in</p><p>8this region were active or partially active for the synthesis of CG (Fig. 1B and S2A)</p><p>9indicating that this region can tolerate pentapeptide insertions without significant loss of</p><p>10activity. The inactive mutant Cgs-344 (Fig. 1B) did not produce a protein readily detected</p><p>11by Coomassie Blue-staining SDS-PAGE (Fig. S2B) or immunobloting (data not shown);</p><p>12therefore, it was not considered in the analysis. Interestingly, Cgs-160 and Cgs-302</p><p>13insertions produced CG with a DP higher than that of the wild type; this phenotype is</p><p>14similar to that previously observed with truncated or some pentapeptide insertion mutants</p><p>15in the C-terminal region of Cgs, which is involved in the control of CG DP (1). In these</p><p>16mutants an important reduction of the incorporation of [14C]-glucose in the protein</p><p>17intermediate was observed; probably do to instability of the proteins (Fig. S2).</p><p>18Furthermore, in Cgs-313 and Cgs-315 mutants the synthesis of CG was significantly</p><p>19reduced but the formation of the intermediate was less affected indicating that in these</p><p>20mutants glucose residues are incorporated in the linear -1-2-glucooligosaccharide</p><p>21protein-linked intermediate but are not efficiently released through the cyclization</p><p>22reaction (Fig. S2). Taken together these results suggest a role of this region in protein-</p><p>23protein interaction. We hypothesize that the N-terminal domain may be interacting with</p><p>1 1 24the cyclization and phosphorylase domains at the C-terminal region allowing the protein</p><p>25to reach the spatial conformation required for the catalysis of these reactions.</p><p>26 Cgs-region (475-818). Cgs pentapeptide insertion mutants Cgs-638, Cgs-681,</p><p>27Cgs-686, Cgs-728, Cgs-751, Cgs-778 and Cgs-785 were inactive while mutant Cgs-696</p><p>28was partially active for the synthesis of CG (Fig. S3A); these results are in agreement</p><p>29with the motility phenotype (Fig. 1B). Furthermore, in these mutants no incorporation of</p><p>30[14C]-glucose in the -1,2-glucooligosaccharide protein-linked intermediate was detected</p><p>31(mutants 638, 728, 751, 778 and 785) or it was significantly reduced (mutants 681, 686</p><p>32and 696) (Fig. S3B). These results are in agreement with those previously obtained after</p><p>33site-directed disruption of the GT-84 domain (2), which result in protein failure to</p><p>34catalyze the initiation and/or elongation reaction/s required for the synthesis of the linear</p><p>35-1,2-glucoologosaccharide protein-linked intermediate.</p><p>36 Cgs-region (870-938). In this small cytoplasmic loop only five pentapeptide</p><p>37insertion mutants were isolated (Fig. 1B). Four of them were inactive for the synthesis of</p><p>38CG in vivo; while surprisingly Cgs-918 was active in vivo but not in vitro (Fig. S4). In</p><p>39addition, Cgs-907 mutant did not produce a protein readily detected by Coomassie Blue-</p><p>40staining SDS-PAGE but it was still active for the incorporation of [14C]-glucose in the -</p><p>411,2-glucooligosaccharide protein-linked intermediate (Fig. S4B). These results indicate</p><p>42that this region may be structurally and/or functionally important for the synthesis of</p><p>43CG.</p><p>44 Cgs-region (1545-2867). Characterization of pentapeptide insertion mutants that</p><p>45map in this region was previously described (1). In agreement with the motility</p><p>46phenotype (Fig. 1B), all these mutants were active for the synthesis of CG.</p><p>2 2 47Legends to figures</p><p>48</p><p>49Figure S1: Screening: motility assay. Individual clones were inoculated in 96 wells</p><p>50plates and grown at 28°C during 48 h. These cultures were used as inoculums for the</p><p>51motility assay which was carried out in yeast extract-mannitol medium (3) with 0.3%</p><p>52agar using a 96 well replica plater. Plus sign, motile; minus sign, non-motile. A1045, A.</p><p>53tumefaciens A1045 mutant strain (chvB::Tn5). A1045 (pBA24), A1045 strain harboring</p><p>54the plasmid pBA24 which expresses wild type Cgs. A1045 strain overproduces</p><p>55exopolysaccharide (4) that accumulates on the surface of the plate.</p><p>56</p><p>57Figure S2: In vivo and in vitro characterization of Cgs-region (1-418) pentapeptide</p><p>58insertion mutants. (A) TLC analysis of CG produced by A1045 strain harboring the</p><p>59indicated plasmid. * and **, migration of anionic and neutral CG, respectively. In this</p><p>60system, slower migration of neutral-CG is higher DP. (B) Incorporation of [14C]-glucose</p><p>61into Cgs. Permeabilized cells of A. tumefaciens A1045 strain harboring the indicated</p><p>62plasmid were incubated with UDP-[14C]glucose during 20 min at 28C. TCA-insoluble</p><p>63fractions were analyzed by Coomassie Blue-staining 10% SDS-PAGE (upper panel) and</p><p>64the radioactivity was detected by autoradiography (lower panel). The position of</p><p>65molecular mass standard (in kDa) is indicated on the left. The arrows on the right indicate</p><p>66the position of wild type Cgs. pBA24, plasmid expressing wild type Cgs.</p><p>67</p><p>68Figure S3: In vivo and in vitro characterization of Cgs-region (475-818) pentapeptide</p><p>69insertion mutants. See legend of Fig. S2.</p><p>3 3 70Figure S4: In vivo and in vitro characterization of Cgs-region (870-938) pentapeptide</p><p>71insertion mutants. See legend of Fig. S2.</p><p>4 4 72References</p><p>73</p><p>741. Ciocchini, A. E., L. S. Guidolin, A. C. Casabuono, A. S. Couto, N. Iñón de</p><p>75 Iannino, and R. A. Ugalde. 2007. A glycosyltransferase with a length-</p><p>76 controlling activity as a mechanism to regulate the size of polysaccharides. Proc</p><p>77 Natl Acad Sci U S A 104:16492-7.</p><p>782. Ciocchini, A. E., M. S. Roset, G. Briones, N. Iñón de Iannino, and R. A.</p><p>79 Ugalde. 2006. Identification of active site residues of the inverting</p><p>80 glycosyltransferase Cgs required for the synthesis of cyclic beta-1,2-glucan, a</p><p>81 Brucella abortus virulence factor. Glycobiology 16:679-91.</p><p>823. de Iannino, N. I., and R. A. Ugalde. 1993. Biosynthesis of cyclic beta-(1-</p><p>83 3),beta-(1-6) glucan in Bradyrhizobium spp. Arch Microbiol 159:30-8.</p><p>844. Geremia, R. A., S. Cavaignac, A. Zorreguieta, N. Toro, J. Olivares, and R. A.</p><p>85 Ugalde. 1987. A Rhizobium meliloti mutant that forms ineffective pseudonodules</p><p>86 in alfalfa produces exopolysaccharide but fails to form beta-(1----2) glucan. J</p><p>87 Bacteriol 169:880-4.</p><p>88</p><p>5 5</p>
Details
-
File Typepdf
-
Upload Time-
-
Content LanguagesEnglish
-
Upload UserAnonymous/Not logged-in
-
File Pages5 Page
-
File Size-