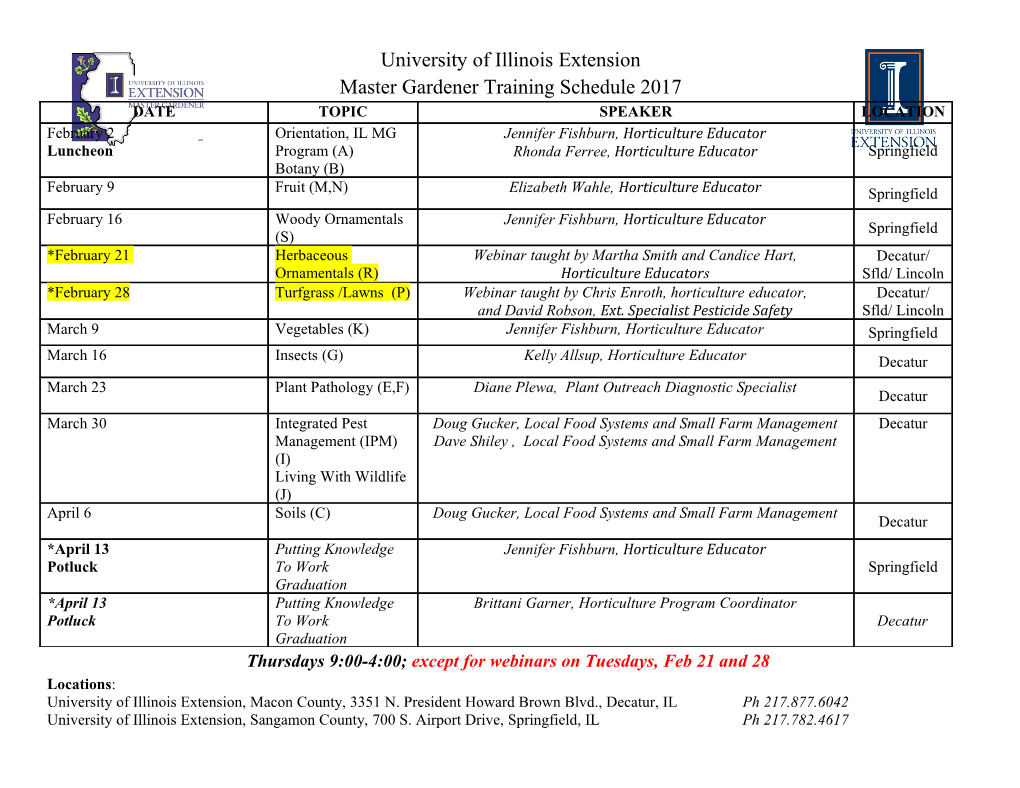
<p>Proteomics 1 Saurabh Sharma</p><p>Prote omics</p><p>Saurabh Sharma M. Sc.( Honors school) 1st Year 18th January 2008 Proteomics 2 Saurabh Sharma</p><p>Table of Contents Gel electrophoresis...... 4 Separation...... 4 Visualization...... 4 SDS-PAGE...... 5 Two-dimensional gel electrophoresis...... 6 Mass spectrometry...... 6 Working of a Mass Spectrometer...... 8 Instrumentation...... 8 Ion source...... 8 Mass analyzer...... 9 Types of Mass Analyzers...... 9 Sector field mass analyzer...... 9 Time-of-flight mass analyser...... 9 Quadrupole mass analyser...... 9 Figure: Quadrupole analyser...... 10 Quadrupole ion trap analyser...... 10 Fourier Transform Ion Cyclotron Resonance(FTICR) (analyser)...... 11 Orbitrap analyser...... 11 Detector...... 11 Tandem Mass Spectrometry...... 12 MALDI(Matrix assisted laser-desorption ionization)...... 12 Matrix...... 13 Laser...... 14 Reproducibility and performance...... 15 AP-MALDI...... 15 Surface-enhanced laser desorption/ionization(SELDI)...... 16 Data Representations...... 16 Mass Spectrum...... 17 Data analysis and interpretation...... 18 Reference...... 18</p><p>Introduction Large scale screening of proteins, their expression, modifications and interactions by using Proteomics 3 Saurabh Sharma high-throughput approaches. Proteins are vital parts of living organisms, as they are the main components of the physiological metabolic pathways of cells. The term "proteomics" was coined to make an analogy with genomics, the study of the genes. The word "proteome" is a combination of "protein" and "genome". The proteome of an organism is the set of proteins produced by it during its life, and its genome is its set of genes.</p><p>Proteomics is often considered the next step in the study of biological systems, after genomics. It is much more complicated than genomics, mostly because while an organism's genome is rather constant, a proteome differs from cell to cell and constantly changes through its biochemical interactions with the genome and the environment. One organism has radically different protein expression in different parts of its body, different stages of its life cycle and different environmental conditions. Another major difficulty is the complexity of proteins relative to nucleic acids. E.g., in human there are about 25 000 identified genes but an estimated >500 000 proteins that are derived from these genes. This increased complexity derives from mechanisms such as alternative splicing, protein modification (glycosylation,phosphorylation) and protein degradation. Scientists are very interested in proteomics because it gives a much better understanding of an organism than genomics. This is because of several reasons; 1. The level of transcription of a gene gives only a rough estimate of its level of expression into a protein. An mRNA produced in abundance may be degraded rapidly or translated inefficiently, resulting in a small amount of protein. 2. Many proteins experience post-translational modifications that profoundly affect their activities; for example some proteins are not active until they become phosphorylated. Methods such as phosphoproteomics and glycoproteomics are used to study post-translational modifications. 3. Many transcripts give rise to more than one protein, through alternative splicing or alternative post-translational modifications. 4. Finally, many proteins form complexes with other proteins or RNA molecules, and only function in the presence of these other molecules. Since proteins play a central role in the life of an organism, proteomics is instrumental in discovery of biomarkers, such as markers that indicate a particular disease. With the completion of a rough draft of the human genome, many researchers are looking at how genes and proteins interact to form other proteins. A surprising finding of the Human Genome Project is that there are far fewer protein-coding genes in the human genome than proteins in the human proteome (20,000 to 25,000 genes vs. > 500,000 proteins). The human body may even contain more than 2 million proteins, each having different functions. The protein diversity is thought to be due to alternative splicing and post-translational modification of proteins. The discrepancy implies that protein diversity cannot be fully characterized by gene expression analysis, thus proteomics is useful for characterizing cells and tissues.</p><p>Gel electrophoresis Gel electrophoresis is a technique used for the separation of deoxyribonucleic acid, ribonucleic acid, or protein molecules using an electric current applied to a gel matrix. It is usually performed for analytical purposes, but may be used as a preparative technique prior to use of other methods such as mass spectrometry, PCR, cloning, DNA sequencing, or Southern blotting for further characterization. Proteomics 4 Saurabh Sharma</p><p>Separation The term "gel" in this instance refers to the matrix used to contain, then separate the target molecules. In most cases the gel is a crosslinked polymer whose composition and porosity is chosen based on the specific weight and composition of the target to be analyzed. When separating proteins or small nucleic acids (DNA, RNA, or oligonucleotides) the gel is usually composed of different concentrations of acrylamide and a cross-linker, producing different sized mesh networks of polyacrylamide. When separating larger nucleic acids (greater than a few hundred bases), the preferred matrix is purified agarose. In both cases, the gel forms a solid, yet porous matrix. "Electrophoresis" refers to the electromotive force (EMF) that is used to move the molecules through the gel matrix. By placing the molecules in wells in the gel and applying an electric current, the molecules will move through the matrix at different rates, usually based on size, toward the negative cathode if positively charged or toward the positive anode if negatively charged. Figure: Gel electrophoresis apparatus - An agarose gel is placed in this buffer-filled box and electrical current is applied</p><p>Visualization After the electrophoresis is complete, the molecules in the gel can be stained to make them visible. Ethidium bromide, silver, or coomassie blue dye may be used for this process. Other methods may also be used to visualize the separation of the mixture's components on the gel. If the analyte molecules fluoresce under ultraviolet light, a photograph can be taken of the gel under ultraviolet lighting conditions. If the molecules to be separated contain radioactivity added for visibility, an autoradiogram can be recorded of the gel. If several mixtures have initially been injected next to each other, they will run parallel in individual lanes. Depending on the number of different molecules, each lane shows separation of the components from the original mixture as one or more distinct bands, one band per component. Incomplete separation of the components can lead to overlapping bands, or to indistinguishable smears representing multiple unresolved components. Bands in different lanes that end up at the same distance from the top contain molecules that passed through the gel with the same speed, which usually means they are approximately the same size. There are molecular weight size markers available that contain a mixture of molecules of known sizes. If such a marker was run on one lane in the gel parallel to the unknown samples, the bands observed can be compared to those of the Proteomics 5 Saurabh Sharma unknown in order to determine their size. The distance a band travels is approximately inversely proportional to the logarithm of the size of the molecule.</p><p>SDS-PAGE Proteins, unlike nucleic acids, can have varying charges and complex shapes, therefore they may not migrate into the gel at similar rates, or at all, when placing a negative to positive EMF on the sample. Proteins therefore, are usually denatured in the presence of a detergent such as sodium dodecyl sulfate/sodium dodecyl phosphate (SDS/SDP) that coats the proteins with a negative charge. Generally, the amount of SDS bound is relative to the size of the protein (usually 1.4g SDS per gram of protein), so that the resulting denatured proteins have an overall negative charge, and all the proteins have a similar charge to mass ratio. Since denatured proteins act like long rods instead of having a complex tertiary shape, the rate at which the resulting SDS coated proteins migrate in the gel is relative only to its size and not its charge or shape. Proteins are usually analyzed by sodium dodecyl sulfate polyacrylamide gel electrophoresis (SDS-PAGE), by native gel electrophoresis, by quantitative preparative native continuous polyacrylamide gel electrophoresis (QPNC-PAGE), or by 2-D electrophoresis.</p><p>Figure: SDS-PAGE autoradiography - The indicated proteins are present in different concentrations in the two samples.</p><p>Two-dimensional gel electrophoresis The two dimensions that proteins are separated into using this technique are isoelectric point and mass. It was invented by O' Ferrel and Klose independently in 1975. This is a method for the separation and identification of proteins in a sample by displacement in 2 dimensions oriented at right angles to one another. This allows the sample to separate over a larger area, increasing the resolution of each component. The model is based on two crucial observations: 1. A molecule placed between two oppositely charged poles will migrate between them at a speed inversely proportional to its mass-to-charge ratio (molecular weight / net charge). 2. Placed in an appropriate medium, a protein will migrate within a gradient of increasing pH until it reaches a spot where the net charge of all its side-chains is 0. This point the isoelectric point of the protein in the given medium.</p><p>The polyacrylamide gel provides a supporting matrix through which proteins can migrate. Proteomics 6 Saurabh Sharma</p><p>This gel has a pH gradient from top to bottom - the top is more acidic than the bottom. A complex protein mixture is loaded in the middle of the left side, where the pH is neutral, and a voltage is applied across the gel. The proteins then migrate through the gel until they reach their isoelectric point (the point at which their charge is the same as the surrounding pH). This technique is called isoelectric focussing.</p><p>The gel is then soaked in a denaturing solution (which causes the proteins to unfold) containing the detergent sodium dodecylsulphate (SDS). This molecule has a very strong negative charge and binds to all proteins, effectively making them all the same charge. A voltage is then applied across the gel from side to side with the anode (positive terminal) at the right. The proteins all move towards the anode, but smaller proteins move faster through the gel than larger ones, thus separating the proteins according to their size. Two-dimensional polyacrylamide gel electrophoresis (2D-PAGE) is used to separate mixtures of proteins, and is particularly useful for comparing related samples such as healthy and diseased tissue. The first step is to load the proteins onto the gel, which has a pH gradient from top to bottom. </p><p>Mass spectrometry Mass spectrometry is an analytical technique that measures the mass-to-charge ratio of ions. The underlying principle of all mass spectrometers is that the paths of gas phase ions in electric and magnetic fields are dependent on their mass-to-charge ratios which is used by the analyzer to distinguish the ions from one another. The mass spectrum is measured by a mass spectrometer.</p><p>Mass spectrometers consist of three basic parts: an ion source, a mass analyzer, and a detector system. The stages within the mass spectrometer are: Proteomics 7 Saurabh Sharma</p><p>1. Production of ions from the sample 2. Separation of ions with different masses 3. Detection of the number of ions of each mass produced 4. Collection of data to generate the mass spectrum</p><p>Working of a Mass Spectrometer Figure: Schematic diagram of working of a Mass spectrometer</p><p>Instrumentation</p><p>Ion source The ion source is the part of the mass spectrometer that ionizes the material under analysis (the analyte). The ions are then transported by magnetic or electric fields to the mass analyzer. Techniques for ionization have been key to determining what types of samples can be analyzed by mass spectrometry. Electron ionization and chemical ionization are used for gases and vapors. In chemical ionization sources, the analyte is ionized by chemical ion- molecule reactions during collisions in the source. The gas phase reaction producing electron ionization is Proteomics 8 Saurabh Sharma</p><p>+ °° where M is the atom of molecule being ionized, e − is the electron, and M is the resulting ion. Two techniques often used with liquid and solid biological samples include matrix-assisted laser desorption/ionization (MALDI, by F. Hillenkamp) and electrospray ionization (by John Fenn )</p><p>Mass analyzer Mass analyzers separate the ions according to their mass-to-charge ratio. All mass spectrometers are based on dynamics of charged particles in electric and magnetic fields in vacuum where the following two laws apply: F = q(E+v X B) (Lorentz force law)</p><p>F = ma (Newton's second law of motion) where F is the force applied to the ion, m is the mass of the ion, a is the acceleration, q is the ionic charge, E is the electric field, and v x B is the vector cross product of the ion velocity and the magnetic field Equating the above expressions for the force applied to the ion yields: (m/q)a = E + v X B This equation reveals that two particles with the same physical quantity m/q behave exactly the same. Together with the particle's initial conditions it completely determines the particle's motion in space and time and therefore is the basis of every mass spectrometer. There are many types of mass analyzers, using either static or dynamic fields, and magnetic or electric fields, but all operate according to this same law. Each analyzer type has its strengths and weaknesses. Many mass spectrometers use two or more mass analyzers for tandem mass spectrometry (MS/MS).</p><p>Types of Mass Analyzers</p><p>Sector field mass analyzer A sector field mass analyzer uses an electric and/or magnetic field to affect the path and/or velocity of the charged particles in some way. As shown in the figure, sector instruments change the direction of ions that are accelerated through the mass analyzer. The ions enter a magnetic or electric field which bends the ion paths depending on their mass-to-charge ratios, deflecting the more charged and faster-moving, lighter ions more. The ions eventually reach the detector and their relative abundances are measured. The analyzer can be used to select a narrow range of m/q or to scan through a range of m/q to catalog the ions present.</p><p>Time-of-flight mass analyser It uses an electric field to accelerate the ions through the same potential, and then measures the time they take to reach the detector. If the particles all have the same charge, the kinetic energies will be identical, and their velocities will depend only on their masses. Lighter ions will reach the detector first. Proteomics 9 Saurabh Sharma</p><p>Quadrupole mass analyser The quadrupole mass analyzer is one type of mass analyzer used in mass spectrometry. In a quadrupole mass spectrometer the quadrupole mass analyzer is the component of the instrument responsible for filtering sample ions, based on their mass-to-charge ratio (m/z). A quadrupole mass analyzer is essentially a mass filter that is capable of transmitting only the ion of choice. A mass spectrum is obtained by scanning through the mass range of interest over time.</p><p>Figure: Quadrupole analyser The quadrupole consists of four parallel metal rods. Each opposing rod pair is connected together electrically and a radio frequency voltage is applied between one pair of rods, and the other. A direct current voltage is then superimposed on the R.F. voltage. Ions travel down the quadrupole in between the rods. Only ions of a certain m/z will reach the detector for a given ratio of voltages: other ions have unstable trajectories and will collide with the rods. This allows selection of a particular ion, or scanning by varying the voltages.</p><p>Quadrupole ion trap analyser The quadrupole ion trap works on the same physical principles as the QMS, but the ions are trapped and sequentially ejected. Ions are created and trapped in a mainly quadrupole RF potential and separated by m/q, non-destructively or destructively.</p><p>Figure: Scheme of a Quadrupole ion trap of classical setup with a particle of positive charge (red), surrounded by a cloud of similarly charged particles (red). The electric field E (blue) is generated by a quadrupole of endcaps (a, positive) and a ring electrode (b). Picture 1 and 2 show two states during an AC cycle.</p><p>There are many mass/charge separation and isolation methods but most commonly used is the mass instability mode in which the RF potential is ramped so that the orbit of ions with a mass a > b are stable while ions with mass b become unstable and are ejected on the z- axis onto a detector. Ions may also be ejected by the resonance excitation method, whereby a supplemental oscillatory excitation voltage is applied to the endcap electrodes, and the trapping voltage Proteomics 10 Saurabh Sharma amplitude and/or excitation voltage frequency is varied to bring ions into a resonance condition in order of their mass/charge ratio. The cylindrical ion trap mass spectrometer is a derivative of the quadrupole ion trap mass spectrometer.</p><p>Fourier Transform Ion Cyclotron Resonance(FTICR) (analyser)</p><p>Fourier transform mass spectrometry, or more precisely Fourier transform ion cyclotron resonance MS, measures mass by detecting the image current produced by ions cyclotroning in the presence of a magnetic field. Instead of measuring the deflection of ions with a detector such as an electron multiplier, the ions are injected into a Penning trap (a static electric/magnetic ion trap) where they effectively form part of a circuit. Detectors at fixed positions in space measure the electrical signal of ions which pass near them over time producing cyclical signal. Since the frequency of an ion's cycling is determined by its mass to charge ratio, this can be deconvoluted by performing a Fourier transform on the signal. FTMS has the advantage of high sensitivity (since each ion is 'counted' more than once) and much high resolution and thus precision.</p><p>Orbitrap analyser</p><p>In the Orbitrap, ions are electrostatically trapped in an orbit around a central, spindle-shaped electrode. The electrode confines the ions so that they orbit around the central electrode and oscillate back and forth along the central electrode's long axis. This oscillation generates an image current in the detector plates which is recorded by the instrument. The frequencies of these image currents depend on the mass to charge ratios of the ions in the Orbitrap. Similar to Fourier transform ion cyclotron resonance mass spectrometers, Orbitraps have a high mass accuracy, high sensitivity and a good dynamic range</p><p>Detector The final element of the mass spectrometer is the detector. The detector records the charge induced or current produced when an ion passes by or hits a surface. Proteomics 11 Saurabh Sharma</p><p>Figure: Continuous dynode electron multiplier detector Typically, some type of electron multiplier is used, though other detectors including Faraday cups and ion-to-photon detectors are also used. Because the number of ions leaving the mass analyzer at a particular instant is typically quite small, significant amplification is often necessary to get a signal. Microchannel Plate Detectors are commonly used in modern commercial instruments. In FTMS and Orbitraps, the detector consists of a pair of metal surfaces within the mass analyzer/ion trap region which the ions only pass near as they oscillate. No DC current is produced, only a weak AC image current is produced in a circuit between the electrodes. Other inductive detectors have also been used.</p><p>Tandem Mass Spectrometry Multiple stages of mass analysis separation can be accomplished with individual mass spectrometer elements separated in space(i.e. they are separated physically) or in a single mass spectrometer with the MS steps separated in time(i.e. done at different time). In tandem mass spectrometry in space, the separation elements are physically separated and distinct, although there is a connection between the elements to maintain high vacuum. These elements can be sectors, transmission quadrupole, or time-of-flight. In a tandem mass spectrometry in time instrument, the separation is accomplished with ions trapped in the same place, with multiple separation steps taking place over time. A quadrupole ion trap or FTMS instrument can be used for such an analysis. Trapping instruments can perform multiple steps of analysis, which is sometimes referred to as MSn (MS to the n). Often the number of steps, n, is not indicated, but occasionally the value is specified; for example MS3 indicates three stages of separation. Proteomics 12 Saurabh Sharma</p><p>[EI: Electron Ionization, ESI: Electron spray Ionization]</p><p>MALDI(Matrix assisted laser-desorption ionization) Traditional Mass Spectometry fails with peptides, proteins, and DNA. Molecules of that size and charge are not volatile. Furthermore, under high energy electron bombardment, they would disintigrate into countless components.</p><p>However high molecular weight ions can be made to jump into the vacuum with the help of MALDI, developed by Franz Hillenkamp at the end of 1980's.</p><p>The proteins are first incorporated in the crystals of UV-adsorbant material. The proteins are first incorporated in the crystals of UV-adsorbant material. The protein doped crystals are then pushed in the high vacuum of the MS and irradiated with UV laser pulse. This explosively releases releases the UV-adsorbent molecules and with them the built-in protein ions. Molecule with such properties of co-crystal formation, proton transfer, UV adsorption are called matrix.</p><p>The proteins(+ protons) enter the gas phase without any hydrate and counter ions such as Na+ or Cl-. It is the single polypeptide chains that appear in the gas phase. Quarternary proteins disintigrate into their subunits in the acidic, denaturing matrix solution itself. Proteomics 13 Saurabh Sharma</p><p>Figure: Matrix-assisted laser desorption ionization depicted with matrix in blue and analyte in red</p><p>Matrix The matrix consists of crystallized molecules, of which the three most commonly used are 3,5-dimethoxy-4-hydroxycinnamic acid (sinapinic acid), α-cyano-4-hydroxycinnamic acid (alpha-cyano or alpha-matrix) and 2,5-dihydroxybenzoic acid (DHB) Matrix compounds is selected to some extent by trial and error, but they are based on some specific molecular design considerations:</p><p> They are of a fairly low molecular weight (to allow facile vaporization), but are large enough (with a low enough vapor pressure) not to evaporate during sample preparation or while standing in the spectrometer.</p><p> They are acidic, therefore act as a proton source to encourage ionization of the analyte.</p><p> They have a strong optical absorption in the UV, so that they rapidly and efficiently absorb the laser irradiation.</p><p> They are functionalized with polar groups, allowing their use in aqueous solutions.</p><p>The matrix solution is mixed with the analyte (e.g. protein-sample). The organic solvent allows hydrophobic molecules to dissolve into the solution, while the water allows for water- soluble (hydrophilic) molecules to do the same. This solution is spotted onto a MALDI plate (see figure). The solvents vaporize, leaving only the recrystallized matrix, but now with analyte molecules spread throughout the crystals. The matrix and the analyte are said to be co-crystallized in a MALDI spot. Proteomics 14 Saurabh Sharma</p><p>Figure: MALDI plate</p><p>Laser The laser is fired at the crystals in the MALDI spot. The matrix absorbs the laser energy and it is thought that primarily the matrix is ionized by this event. The matrix is then thought to transfer part of its charge to the analyte molecules (e.g. protein), thus ionizing them while still protecting them from the disruptive energy of the laser. Ions observed after this process consist of a neutral molecule [M] and an added or removed ion. Together, they form a quasimolecular ion, for example [M+H]+ in the case of an added proton, [M+Na]+in the case of an added sodium ion, or [M-H]- in the case of a removed proton. MALDI generally produces singly-charged ions, but multiply charged ions ([M+nH]n+) can also be observed, usually as a function of the matrix, the laser intensity and/or the voltage used. Note that these are all even-electron species. Ion signals of radical cations can be observed eg. in case of matrix molecules and other stable molecules. Lasers Used for MALDI Laser Wavelength (nm) Nitrogen laser 337 Nd:YAG(neodymium-doped 355, 266 yttrium aluminium garnet; </p><p>Nd:Y3Al5O12) Er:YAG (erbium-doped 2940 yttrium aluminium garnet (Er:Y3Al5O12)</p><p>CO2 10,600</p><p>Reproducibility and performance The sample preparation for MALDI is important for the result. Inorganic salts which are also part of protein extracts interfere with the ionization process. The salts are removed by solid Proteomics 15 Saurabh Sharma phase extraction or washing the final target spots with water. Both methods can also remove other substances from the sample. The matrix-protein mixture is not homogenous because the polarity difference leads to a separation of the two substances during crystallization. The spot diameter of the target is much larger than that of the laser, which makes it necessary to do several laser shots at different places of the target within the target spot. The matrix composition, the addition of trifluoroacetic acid and formic acid, delay between laser pulses, delay time of the acceleration power, laser wavelength, energy density of the laser and the impact angle of the laser on the target are among others the critical values for the quality and reproducibility of the method.</p><p>AP-MALDI Atmospheric pressure (AP) matrix-assisted laser desorption/ionization (MALDI) is an ionization technique (ion source) that in contrast to vacuum MALDI operates at normal atmospheric environment.The main difference between vacuum MALDI and AP-MALDI is the pressure in which the ions are created. In vacuum MALDI, ions are typically produced at 10 mTorr or less while in AP-MALDI ions are formed in atmospheric pressure. Disadvantage of the AP MALDI source is the limited sensitivity observed and the limited mass range. AP-MALDI is used in mass spectrometry (MS) in a variety of applications ranging from proteomics to drug discovery fields. Popular topics that are addressed by AP-MALDI mass spectrometry include: proteomics, DNA/RNA/PNA, lipids, oligosaccharides, phosphopeptides, bacteria, small molecules and synthetic polymers, similar applications as available also for vacuum MALDI instruments. The AP-MALDI ion source is easily coupled to an ion trap mass spectrometeror any other MS system equipped with ESI (electrospray ionization) or nanoESI source.</p><p>Surface-enhanced laser desorption/ionization(SELDI)</p><p>Surface-enhanced laser desorption/ionization (SELDI) is an ionization method in mass spectrometry that is used for the analysis of protein mixtures.SELDI is typically used with time-of-flight mass spectrometers and is used to detect proteins in tissue samples, blood, urine, or other clinical samples. Comparison of protein levels between patients with and without a disease can be used for biomarker discovery.</p><p>SELDI-TOF-MS is a variation of matrix-assisted laser desorption/ionization (MALDI) that uses a target modified to achieve biochemical affinity with the analyte compound. In MALDI, a protein or peptide sample is mixed with the matrix molecule in solution and small amounts of the mixture are deposited on a surface and allowed to dry. The sample and matrix co-crystallize as the solvent evaporates. Proteomics 16 Saurabh Sharma</p><p>In SELDI the protein mixture is spotted on a surface modified with a chemical functionality. Some proteins in the sample bind to the surface, while the others are removed by washing. After washing the spotted sample, the matrix is applied to the surface and allowed to crystallize with the sample peptides. Binding to the SELDI surface acts as a separation step and the subset of proteins that bind to the surface are easier to analyze. Common surfaces include CM10 (weak-positive ion exchange), H50 (hydrophobic surface, similar to C6-C12 reverse phase chromatography), IMAC30 (metal-binding surface), and Q10 (strong anion exchanger). Surfaces can also be functionalized with antibodies, other proteins, or DNA. </p><p>Spectrometric Data</p><p>Data Representations Most common type of data produced by a Mass spectrophotometer is a Mass Spectrum. However some MS's may also produce a Contour map ( The kind of map used to represent topology of a geographical area, points of same intensity joined to each other). Hence we get a 3D data representation. Proteomics 17 Saurabh Sharma</p><p>Mass Spectrum A mass spectrum is an intesity vs. m/z(mass to charge ratio) plot representing a chemical analysis. The x-axis of a mass spectrum represents a relationship between the mass of a given ion and the number of elementary charges that it carries. This is written as the IUPAC* standard m/z to denote the quantity formed by dividing the mass of an ion by the unified atomic mass unit and by its charge number (positive absolute value). This has been referred to as a mass-to-charge ratio. The y-axis of a mass spectrum represents signal intensity of the ions. The signal intensity may represent Counts per second(cps) or power of the signal sine wave, etc depending on the kind of Mass spectrometer and hence the detector being used; When using counting detectors the intensity is often measured in counts per second (cps). When using analog detection electronics the intensity is typically measured in volts. In FTICR(Fourier transform ion cyclotron resonance) and Orbitraps the frequency domain signal (the y-axis) is related to the power (~amplitude squared) of the signal sine wave (often reduced to an rms power); however, the axis is usually not labeled as such since in most forms of mass spectrometry, the intensity of ion current measured by the spectrometer does not accurately represent relative abundance, but correlates loosely with it. Therefore it is common to label the y-axis with "arbitrary units". Signal intensity may be dependent on many factors, especially the nature of the molecules being analyzed and how they ionize. The efficiency of ionization varies from molecule to molecule and from ion source to ion source. For example, in electrospray sources in positive ion mode a quaternary amine will ionize exceptionally well whereas a large hydrophobic alcohol will most likely not be seen no matter how concentrated. In an EI source these molecules will behave very differently. Additionally there may be factors that affect ion transmission disproportionally between ionization and detection. On the detection side there are many factors that can also affect signal intensity in a non-proportional way. The size of the ion will affect the velocity of impact and with certain detectors the velocity is proportional to the signal output. In other detection systems, such as FTICR, the number of charges on the ion are more important to signal intensity. In Fourier transform ion cyclotron resonance and Orbitrap type mass spectrometers the signal intensity (Y-axis) is related to the amplitude of the free induction decay signal. This is</p><p>* International Union of Pure and Applied Chemistry Proteomics 18 Saurabh Sharma fundamentally a power relationship (amplitude squared) but often computed as an [rms]. For decaying signals the rms is not equal to the average amplitude. Additionally the damping constant (decay rate of the signal in the fid) is not the same for all ions. In order to make conclusions about relative intensity a great deal of knowledge and care is required. A common way to get more quantitative information out of a mass spectrum is to create a standard curve to compare the sample to. This requires knowing what is to be quantitated ahead of time, having a standard available and designing the experiment specifically for this purpose. A more advanced variation on this the use of an internal standard which behaves very similarly to the analyte. This is often an isotopically labeled version of the analyte. There are forms of mass spectrometry, such as accelerator mass spectrometry that are designed from the bottom up to be quantitative.</p><p>Data analysis and interpretation Mass Spectrometric data analysis depends on the kind of experiment producing the data. There are several factors that should be kept in mind while analysing and interpreting the data provided by a Mass Spectrometer or a MALDI apparatus:</p><p>1. Different kinds of ion sources behave differently from each other and produce different kinds of data. For example, electron ionization source produces many fragments, Tandem mass spectrometry purposly produces fragment ions post source and has a great impact on the kind of Data achieved by an experiment. 2. Thee kind of sample being analysed also effects the data interpretation. For example if the sample is a relatively crude preparation of a biological sample, the sample likely contains a certain amount of salt that may form adducts with the analyte molecules in certain analyses. 3. How and from where a sample is prepared and how it is used/ introduced also has considerable effect on the result. For example, the kind of matrix used for MALDI. 4. Most importantly, one must understand the goal of the project (and collect the right kind of data in the first place) while interpreting a spectrometric data. One may be interested in knowing the masses of the molecules or the purity of the sample or the structure of the molecule or all the above.</p><p>Reference 1. http://www.iupac.org/goldbook/T06250.pdf 2. Atmospheric pressure matrix-assisted laser desorption/ionization(AP MALDI) on a quadrupole ion trap mass spectrometer. Susanne C. Moyer, Lisa A. Marzilli, Amina S. Woods, Victor V. Laikod, Vladimir M. Doroshenkod, Robert J. Cotter 3. Proteinchip surface enhanced laser desorption/ionization (SELDI) mass spectrometry: a novel protein biochip technology for detection of prostate cancer biomarkers in complex protein mixtures. GL Wright Jr, LH Cazares, S-M Leung, S Nasim, B-L Adam, T-T Yip, PF Schellhammer, L Gong & A Vlahou 4. Development of a Three-Dimensional Topographic Map Display for Capillary Electrophoresis/Mass Spectrometry with an Ion Trap/Reflectron Time-of-Flight Mass Spectrometer Detector: Applications to Proteomics 19 Saurabh Sharma</p><p>Tryptic Digests of Isoforms of Myelin Basic Protein. Michael X. Li, Jing-Tao Wu, Steve Parus and David M. Lubman 5. Preparative Native Continuous Polyacrylamide Gel Electrophoresis (PNC-PAGE): An Efficient Method for Isolating Cadmium Cofactors in Biological Systems. Bernd Kastenholz 6. 2D Gel Electrophoresis - An Overview. Louigi Addario-Berry; http://www.mcb.mcgill.ca/~hallett/GEP/PLecture3 7. http://genome.wellcome.ac.uk</p>
Details
-
File Typepdf
-
Upload Time-
-
Content LanguagesEnglish
-
Upload UserAnonymous/Not logged-in
-
File Pages19 Page
-
File Size-