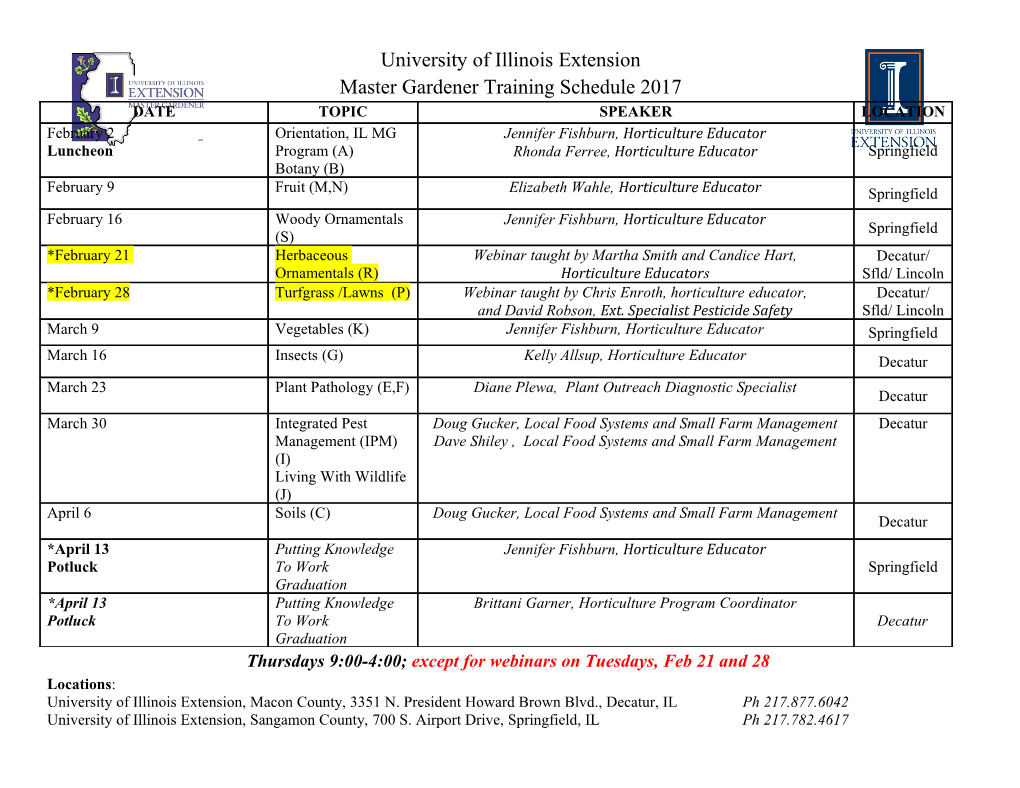
<p>In vitro site specific mutagenesis </p><p>Background</p><p>Site specific in vitro mutagenesis is the introduction of a specific preconceived mutation. This could be done to test a specific hypothesis about a DNA regulatory sequence, or a protein sequence, or an RNA sequence.</p><p>General Methods</p><p>Phagemid-based methods</p><p>Of the non-PCR procedures, the most common is referred to as the Kunkel method (Kunkel TA 1985. PNAS 82:488-492). In this method, the plasmid must be prepared as a single stranded circle. To facilitate this, the plasmid will have a single stranded origin of replication of the type used by bacteriophage fd (or M13). Phage fd replicates and packages a single stranded circle of its genome during infection. If an fd helper phage infects cells carrying a plasmid with a single stranded ori, the plasmid will be packaged as a single stranded circle also. Such plasmids are called "phagemids". The single stranded circle can be recovered from the viral capsids and primed for DNA replication with a mutagenic oligonucleotide. After extension to complete the circle, transformation will result in a mixture of wild type and mutant plasmids.</p><p>Unfortunately, the E. coli mismatch repair systems can determine which was the parental strand by its content of methyl groups, and will fix the mismatch mainly to the wild type. This system is used by E. coli to proofread its finished replicated DNA for replication errors. Hence, without some additional method to favor the mutant sequence, 90-97% of the clones will be wild type. For example, in this early forerunner of the Kunkel method the target gene was cloned directly in an M13 vector, mutagenized, and the resulting clones screened by hybridization for mutants. The mutation frequency was only 1%.</p><p>Figure modified from Winter et al., Nature 299: 756-758 (1982).</p><p>1 The initial Kunkel method circumvented this problem by producing the phagemid in a dut- ung- host. The dut- mutation causes dUTP to accumulate resulting in incorporation of uracil into the DNA. The ung- mutation inactivates a repair system that would have caused repair resynthesis removing the uracil. After priming with the mutagenic oligo, extension in vitro, and transformation into ung+ E. coli, the ung repair system will be activated. The parental strand will be nicked and gapped to remove the uracil stimulating repair synthesis using the mutated strand as template. </p><p>Instead of using uracil incorporation, may of the kits vended to support this style of mutagenesis make use of the methylation-dependent restriction enzyme DpnI. DpnI nicks the parental strand at the same methylated sites that are normally used to distinguish the parental strand from the daughter strand by the mismatch repair system. The unmethylated newly synthesized strand is not nicked. This causes repair synthesis using the parental strand as template after transformation. </p><p>Alternatively, one can use a MutS host. The MutS mutation knocks out the mismatch repair system that would resolve the heteroduplex. After an additional round of replication both mutant and wild type DNA will be present in the cell, and this will resolve to an equal number of mutant and wild type clones. </p><p>Finally, if one uses more than one mutagenic oligonucleotide at the same time, there is a greater than 70% comutation frequency. The GeneEditor kit from Promega uses this fact in combination with oligonucleotides that change the specificity of beta-lactamase for different variations of ampicillin to positively select for mutations.</p><p>The major problem with Kunkel mutagenesis is that there is no good way to be sure that the polymerase is making it all the way around the circle. The thermostable polymerases used with the PCR version of this strategy seem to have less trouble getting around the circle, possibly because the higher temperature of polymerization destabilizes secondary structure in the template. The thermostable polymerases can be used in the Kunkel method also, but one has to be sure to avoid a polymerase with an effective 5'->3' exonuclease activity, because it may take out the mutant oligo as the circle is cloned.</p><p>2 Whole plasmid linear amplification.</p><p>This strategy is probably the most popular in current practice. It eliminates the need for the phagemid, and can work on any circular plasmid. It requires two synthetic oligos, each complementary to the other, and both carrying the change to be introduced. This method is typically done in a thermal cycler with a thermostable proofreading DNA polymerase. Because the conditions are like PCR, this method is often referred to as a PCR method. It is not a chain reaction, however, because the product of each cycle is not a template for further synthesis. The products build up proportional to the number of cycles. This is called 'linear amplification'. The linear amplification compensates for the fact that only a portion of the initial plasmid is nicked during the first denaturation releasing single stranded template. During denaturation and renaturation, the doubly mutated nicked circle on the right is mostly formed. The major unwanted species is the original plasmid surviving because it was never nicked. The unmutated plasmid is destroyed with DpnI which destroys any methylated DNA. The methylation will have happened when the plasmid was originally grown up as long as it was grown in a dam+ host. This method is incorporated in Agilent's Quick Change kit (http://www.genomics.agilent.com/CollectionSubpage.aspx? PageType=Product&SubPageType=ProductDetail&PageID=387), although there is no real reason to buy a kit for this method.</p><p>Keep in mind that you are vulnerable to secondary mutations anywhere in the vector. So you should sequence the entire insert plus regions of the vector important to your experiment (like the promoter) to confirm your construct. It is, however, true that linear amplification only copies the template once to make each product, so the incidence of polymerization errors encountered is lower than what is found in a PCR product generated with the same enzyme. It will be necessary to use a polymerase that does not displace the oligonucleotide.</p><p>3 Troubleshooting the linear amplification strategy:</p><p>This procedure is the most common one brought to me by people having trouble getting their mutant clones. First recognize that this is not PCR, but is linear amplification. Small fragments amplifying dramatically would indicate false priming in a way to generate an actual PCR product. These are at best superfluous, and at worst may compete with forming the desired products. The product formed by annealing the two amplified strands should correspond to the nicked circle form of the initial plasmid. However, it may not be reasonable to expect to build up enough of that product to exceed the amount of nicked circle contributed from the initial starting plasmid. An excess of one single strand without the other would be difficult to observe, because single stranded DNA stains less intensely, and is distributed into a heterogeneous smear due to the abundance of different ways secondary structure can form. I recommend putting each primer in a tube separately with the starting template for thermal cycling. This would prevent primer dimer formation between the two primers. More importantly, it allows examination of each reaction product separately. If one of the primers is not priming, it may become apparent from running the product on an agarose gel. Quantitative PCR could be performed to rigorously confirm if linear amplification is occurring in each of the separated reactions. Another potential problem is that the polymerase may not be making it all the way around the circle. You could assay whether the far end of the linearly amplified strand has actually amplified by quantitative PCR. If the polymerase doesn't make it around the circle, try increasing the extension times, or try a different polymerase. </p><p>In cases were one wants to incorporate a complex set of changes within the mutagenic oligo, it may be hard to get the oligos to prime at the desired sites without also false priming at another site and creating superfluous PCR products. In that case, a cassette method wherein the amount of template in the reaction is reduced may be a preferable approach.</p><p>Methods involving a cassette.</p><p>A cassette is a small region which one first arranges to be bounded by two restriction sites that are each unique within the plasmid. Often it will require a preliminary mutagenesis experiment to place the bounding restriction sites. Because of the overhead involved, cassettes are often used when multiple mutations are to be created in the same small region. The purpose of the cassette is to allow a small region to be mutagenized separately and then used to replace the wild type version of the cassette in the initial construct by directional cloning. Cassettes may also be used to allow moving a mutagenized region from one vector to another. This may be done to guard against inadvertently introducing second site mutations, especially if PCR is involved in the method of construction. The usual method of guarding against second site mutations is to resequence. In a cassette strategy, one would only have to resequence the cassette.</p><p>4 Back to back primers</p><p>In the strategy shown below, X and Y represent unique restriction sites defining a cassette. </p><p>One flanking primer is paired with a mutagenic primer, and the other is paired with a second primer that is back to back with the mutagenic primer. The idea is to create two PCR fragments that can be pieced together by blunt end ligation. Cleavage at X and Y allow this mutagenized insert to be put back in the cloning vector.</p><p>Ausubel, Current Protocols in Molecular Biology.</p><p>Overlapping mutagenic primers</p><p>A problem with the above strategy is the usual difficulty obtaining blunt end ligation with PCR products. The strategy below removes the blunt ended ligation from the procedure. Again the insert is amplified as two adjacent fragments, but in this case the two fragments overlap at the site of the mutation. Both fragments are synthesized with mutagenic primers. </p><p>Instead of blunt end ligation, a second round of PCR is used to fuse the two fragments. To accomplish this, small amounts of the overlapping fragments are mixed together in a PCR reaction with only the outside primers present. At some frequency, one of the fragments will prime on the other and</p><p>5 generate a full length insert, which is then rescued by amplification with the outside primers. Ausubel,</p><p>Current Protocols in Molecular Biology. </p><p>These methods have become less popular due to the simplicity of the linear amplification strategy given above. But in a situation where the mutagenesis is not working, the above strategy has the advantage that intermediates can be examined to figure out what is going wrong.</p><p>Resynthesized cassettes.</p><p>Oligonucleotides can now be made of greater than 100 nt. This allows complete resynthesis of a cassette of up to 200 bp by the procedure below. This is particularly useful for saturation mutagenesis approaches because there is no need for priming to include extensively mismatched regions. </p><p>The mixture is cloned, and individual clones are sequenced and subjected to characterization.</p><p>Saturation mutagenesis is an in vitro mutagenesis strategy wherein one tries to generate all (or most) possible mutations within a narrow region of a gene. The two most popular ways to make the mutations are cassette mutagenesis, or oligo-directed mutagenesis. Both depend on synthesizing oligonucleotides covering the regions to be mutagenized such that the oligos contain a random assortment</p><p>6 of incorrect bases. A third way is to conduct PCR under conditions that favor a high degree of misincorporation.</p><p>In cassette mutagenesis, the region to be mutagenized is between two unique restriction sites such that it can be cut out and replaced with a double stranded mutagenic oligo. When the mutagenic oligos are synthesized, the substrate for each position to be mutagenized is drawn from a reagent bottle with a fixed amount of the incorrect bases mixed in with the correct bases. The amount of misincorporation is adjusted to yield some preconceived average total number of changes per oligo. This is frequently adjusted to be an average of one change per cassette, but some experiments have been done to try to sample all double mutations, or an assortment of totally random sequences at the mutagenized positions. The mutagenized residues do not need to be adjacent. </p><p>Creation of deletions</p><p>Small deletion can be created in the same way as point mutations.</p><p>Larger deletion might be used to remove protein domains or to map enhancers and promoters in noncoding regions.</p><p>7 Insertions can also be arranged by PCR fusion.</p><p>8 Linker Scanning</p><p>Noncoding regions may be scanned for relevant control sequences by linker scanning mutagenesis. Linker scanning mutagenesis is a systematic method of making a series of mutations where successive regions of about 10 bases are substituted by an arbitrary sequence (usually containing a restriction site). The essence of linker scanning is to first generate a series of clones with the region truncated at random positions from the right and the left, and then to combine leftwards and rightwards deleted regions so that the only change is at the junction.</p><p>Linker Scanning. After McKnight and Kingsbury, Science 217:316-324 (1982).</p><p>It is also possible to emulate a linker scanning experiment with more ordinary oligo-directed mutagenesis. Sometimes, when people do that, they still call it 'linker scanning'.</p><p>Targets</p><p>Promoter/Enhancer elements.</p><p>Generally, the targeted element will need to be in the context of a functional promoter and set up do drive a reporter gene in an expression vector. If the vector will be assayed in a host other than E. coli, it will generally need both an E. coli origin of replication (ori) and selectable marker, and an ori for the</p><p>9 targeted host. This is because after mutagenesis, the clone will be grown up for sequence confirmation in E. coli, and then transferred into the appropriate host. </p><p>This is a pair of vectors from the Promega catalog to enable a dual color luciferase assay for mutagenized promoters or enhancers. Notice that the f1 (aka fd) ori is present to allow Kunkel mutagenesis directly in the assay vector:</p><p>Notice that two different luciferase proteins are being expressed by these two vectors. They emit light at different frequencies. The idea is to put the wild type promoter/enhancer sequence in one of the plasmids, and a mutated version in the other. Ultimately, mammalian cells will be transformed with a mixture of the two, and the relative amount of mutant and wild type luciferase expressed will be assayed in a dual frequency luminometer. This corrects for variation in transformation efficiency or numbers of cells harvested.</p><p>If the assay is to be in vitro transcription followed by gel analysis of the product, a convenient restriction site should be downstream to linearize the template and fix the length of the transcripts. Other types of regulatory sites (splice sites, ori's, recombination sites, etc.) will similarly have to be within a context wherein they can be assayed.</p><p>Promoter/enhancer regions are usually first characterized by a series of deletion mutants to localize a region for more detailed studies. Historically, one would take advantage of available restriction sites to make a nested set of deletions. PCR now makes it possible to form deletions starting at any arbitrary positions.</p><p>With mammalian genes, much of the preliminaries may be substituted by a bioinformatics analysis based on conservation of noncoding sequences. (See Vista at http://pipeline.lbl.gov/cgi-bin/gateway2).</p><p>10 The above is a screen from the VISTA Browser tool showing the likely proximal promoter region for a human gene by virtue of conservation in the dog and horse genomes. A possible enhancer region may be indicated by conservation in the mouse and rat genomes, although the presence of repetitive DNA must be accounted for.</p><p>Finally, given that a specific motif was implicated by the above methods, one might make single base changes at the conserved position of the motif, monitoring changes in gel mobility shift, a footprint, and expression in coordination.</p><p>Proteins that recognize a nucleic acid sequence.</p><p>Proteins that recognize a nucleic acid sequence provide the possibility of mutating both the protein and the binding sequence. Successful prediction of a mutation in one that compensates a mutation in the other is generally considered as a powerful indication that one's model of the specific contacts that enforce specificity is accurate.</p><p>Ribozymes</p><p>One of the newest targets of mutagenesis are ribozymes, RNA molecules with catalytic activity. Ribozymes would generally be produced by in vitro transcription. If they are to be delivered in vivo, then a transcription terminator will probably be required. Since making ribozymes is an extensive design</p><p>11 problem, some sort of evolutionary strategy is often used (several rounds of saturation mutagenesis interspersed with selection for improved function).</p><p>Other RNAs</p><p> rRNA, tRNA, snRNA, etc. can be the target of in vitro mutagenesis. Because these molecules are heavily modified, they will have to be produced in the appropriate host cells. Some provision will have to be made to separate them from the endogenous host product. For example, one might alter their length. Since these are usually essential genes, it will not be possible to make a null host strain, except by a colony sectoring approach.</p><p>Signals that affect translation efficiency or half life of mRNAs may be identified by in vitro mutagenesis. </p><p>Proteins</p><p>Mutagenized proteins for biochemical characterization will generally have to be in an expression vector designed for mass production of the protein. The vector may also have features that aid the purification of the protein. The promoter should be regulatable. Mutagenized proteins designed for genetic testing will need to be in the appropriate expression vector for that target host cell. A regulatable promoter will aid proving that the phenotypic change is really due to expression of your protein. A regulatable promoter also helps prevent selection against your gene during construction and growth. The promoters in many expression vectors are leaky enough that selection against the insert is a problem even though the vector is grown under "non-expressing" conditions. Hence if the construction is complicated, many investigators would do all the steps in some other vector, including sequence verification. Then a restriction fragment carrying the gene would be moved to the expression vector in the last step.</p><p>One will have to provide for separation of the mutagenized protein from the endogenous protein. Possible solutions are 1) use heterologous host, 2) delete the gene from the host, 3) use a thermostable subject protein, or 4) use an affinity tag purification system. </p><p>Exploring active sites</p><p>Crystal structures generally lead to a hypothesis about the roles of active site residues. However, if a substrate analogue wasn't actually present in the crystal, the placement of substrate in the active site can be quite speculative. Even if the enzyme was crystallized with its substrate, the resolution of a crystal structure is generally insufficient to distinguish whether a proposed interaction (say a hydrogen bond) is positioned well enough to contribute positively to binding energy. Therefore, the contribution of all the proposed contacts to the activity of the enzyme still requires biochemical characterization.</p><p>One is immediately faced with a decision of what to replace these residues with. Experience has shown that putting in a large side chain frequently kills the enzyme, leaving one to wonder if the substrate has been completely blocked out of the active site, or if the conformation of the enzyme has been distorted. Also, introduction of bulky side chains tend to promote aggregation either directly or by</p><p>12 disrupting structure and exposing hydrophobic groups. So this kind of mutant is often uninformative. Substituting an Ala has been most consistently useful as a means of withdrawing a specific substrate- enzyme contact without otherwise distorting the interaction. Gly tends to cause conformational distortion because too much of a hole has been left in the packing of the side chains. </p><p>Other strategies are to pick chemically similar side chains to substitute, or to pick residues appearing at this position in homologous proteins (particularly useful if the homologous protein has altered function). One really should anticipate making a number of replacements at this position and finding that some of them fail to fold and behave in a tractable manner. Strategies that involve changing more than one residue at a time have an increased risk that the final product will not fold or suffer from intractable aggregation problems..</p><p>Active site residues can be conceptualized as performing two different kinds of roles: substrate binding, and catalysis. Mutation of catalytic residues (those that specifically stabilize the transition state) generally kill the activity of the enzyme. One certainly has to do this experiment, because if the enzyme still worked the experiment would reject the proposed mechanism. However, the negative result (dead enzyme) is a weak result. The mutation may have killed the enzyme by altering its conformation in some unexpected way, and the residue may in fact have nothing to do with catalysis. The overall structural integrity of the mutant enzyme could be supported by the following observations: unaltered susceptibility to proteolysis, demonstration that the substrate(s) still binds, demonstration that other aspects of the function are still carried out (e.g. partial reactions not including the mutated step), demonstration that physical properties are unaltered (e.g. fluorescence, circular dichroism, quaternary structure). For small proteins (<45 kD), an NMR method called HSQC could both confirm minimal conformational distortion and that the substrate still binds.</p><p>Here's an example of mutations at catalytic residues involved in the conversion of ATP + tyrosine to tyr-AMP by tyr-tRNA synthetase. In this case there are two catalytic residues, neither one of which is completely essential. </p><p>Enzyme k(rate limiting) KD tyr KD ATP</p><p> wild type 38 s-1 12 uM 4.7 mM His-45 --> Gly 0.16 10 1.2 Thr-40 --> Ala 0.0055 8.0 3.8 His-45 --> Gly & 0.00012 4.5 1.1 Thr-40 --> Ala</p><p>When ATP reacts with tyrosine, the alpha phosphate must go from its normal tetrahedral configuration through a five bonded intermediate in the transition state. This causes the other two phosphates to be physically displaced in the active site. Thr-40 and His-45 are thought to bind the gamma phosphate in the transition state, thus stabilizing it.</p><p>13 From Leatherbarrow et al. (1985) PNAS 82, 7840-7844.</p><p>The effects of altering a substrate contact.</p><p>Mutations to binding residues normally have subtle effects on the Km ~= KD, or substrate specificity (kcat/Km). These two different measures have the following interpretations: The change in binding energy in the [ES] state caused by a mutant is taken as G = -RT ln [Km(wt)/Km(mut)]. This basically assumes that the affect of the mutant on the energy of [ES] is the same as the effect on the transition state [ES]*. In other words, it assumes that the mutant has no measurable effect on kcat. Many investigators use this assumption because Km is much easier to measure than kcat. The change in binding energy in the transition state [ES]* is taken as G = -RT ln [(kcat/Km)mut/(kcat/Km)wt]. This acknowledges that if one does measure kcat for each mutation carefully, one will find that most substrate binding residues really are optimized to contribute at least a little bit to stabilizing [ES]* over [ES]. Justification for this </p><p>14 is found in Fersht A., Enzyme Structure and Mechanism, 2nd Ed. 1985. W.H. FreeMan and Co. </p><p>The substrate is usually bound by many such contacts, so disrupting one of them is insufficient to kill activity. The binding energy contributed by the various contacts is often approximately additive.</p><p>Again looking at tyrosyl-tRNA synthetase, Thr 51 was proposed as a hydrogen bonding contact for the ribose of the ATP. </p><p>Tyrosyl-AMP in the active site of tyrosyl-tRNA synthetase from Fersht et al., Bioch. 24, 5858</p><p>Kinetic effects of various changes to Thr-51</p><p>-1 Enzyme kcat(s ) KM (mM ATP) delta G</p><p> wild type (Thr-51) 8.35 1.08 Cys-51 12.4 0.35 -0.90 Ala-51 8.75 0.54 -0.44 Ser-51 1.88 1.16 +0.92 Gly-51 6.0 1.25 +0.28</p><p>15 From Fersht and Wilkinson (1985) Bioch. 24, 5858-5861. The tabulated delta G values are computed from kcat /KM for ATP and represent the binding energy in the transition state.</p><p>Here we see an advantage of making the comparisons as delta G values. These values are additive, and can be broken down to individual contributions of different atoms in the side chains. If you just look at thr -> gly, you would see that you lost .28 kcal/mol in binding energy, and you might (mistakenly) attribute that to the loss of the hydrogen bond to the substrate. However, you really removed a hydrogen bond, and potential interactions with 2 carbon groups (beta and gamma). The change from thr -> ser shows that you lose 0.92 kcal/mol by withdrawing the contact with the gamma methyl group. The change from ser -> ala shows that you actually gain 1.36 kcal/mol from withdrawing the hydrogen bond, and the change from ala -> glycine shows that you lose another 0.72 kcal/mol from withdrawing the beta methyl group. So thr 51 really contributes favorable hydrophobic contacts plus an unfavorable hydrogen bond.</p><p> group contribution to binding energy (kcal/mol) gamma methyl -0.92 gamma SH -0.46 gamma OH +1.36 beta methyl -0.72</p><p>A negative number denotes improved binding. Note that these numbers are in the range you would expect for individual hydrogen bonds and Van der Waals contacts. The hydroxyl forms an unfavorable H-bond because the distance is 0.5 angstroms too long. The unfavorable bonding energy means that this thr residue forms a 1.36 Kcal better bond to water than the substrate, therefore it disfavors substrate binding. Sulfhydrals tend to form longer H-bonds, therefore the cys residue makes a better contact from this position. You could not get this information by gazing at the crystal structure, because X-ray structures are not accurate to 0.5 angstrom resolution.</p><p>Note that Cys actually makes a better contact with the substrate than the wild type residue, thr. If you look at the velocity versus [ATP] curves for these two enzymes, you see that each has a range of [ATP] where the velocity is greater than the other. This serves to show that tighter binding is not necessarily better.</p><p>16 Figure modified from Fersht and Wilkinson Biochemistry 24: 585-5861 (1985) Mutations that alter structure.</p><p>Mutations that alter structure often have non additive effects on activity when coupled with other mutations.</p><p>In the example of Thr 51 given above, you would expect a proline at residue 51 to contribute no binding energy. Unexpectedly, pro 51 actually improves binding by -1.9 kcal. This is an example of a conformational effect. Pro 51 improves substrate binding by disrupting an alpha helix and making a better contact for His 48. This is shown by observing non additivity in double mutant Thr -> Pro 51, His -> Gly 48 (panel d below). Panels b and c show that there is not much of a conformational effect of pro 51 on binding by Cys 35, or between mutations at residue 48 and 35.</p><p>From Carter et al. (1984) Cell 38, 835-840.</p><p>17 Characterization of quaternary structure.</p><p>Refs: Jones et al., (1985) Bioch. 25, 5852-5857. Ward et al. (1986) JBC 261, 9576-9578.</p><p>The interface between enzyme subunits can be disrupted by placing charged residues in it. The following is an example where complementary changes are made at an interface to convert a homodimer into a heterodimer. </p><p>Tyrosyl-tRNA synthetase is a dimeric enzyme that shows half-of-the-site reactivity. That is, although there are two active sites, they interact with negative cooperativity such that only one is active at a time. The experiment is to set up a situation where one can maneuver a mutation into only one of the two subunits and thereby measure the effect on the other. In this case to physically demonstrate the heterodimer, one of the subunits will be a version carrying a deletion to alter its size. </p><p>By engineering a negatively charged residue into the subunit interface, it was possible to cause dissociation under native conditions by varying the pH. By engineering a positively charged residue into the subunit interface of another variant, it was possible to form heterodimers wherein the two residues formed a salt bridge. This verified that the pH effect was due to subunit dissociation and not due to a generalized unfolding of the enzyme. Further, other mutations could be introduced specifically into one of the subunits of the heterodimer. </p><p>18 Scanning Strategies</p><p>Any strategy that seeks to systematically make changes across a structure for the purpose of mapping function may be referred to as 'scanning'. The most popular strategy is called alanine scanning. The initial paper on alanine scanning was Cunningham and Wells (J. Mol. Biol. 234: 554-563 [1993]). The subject was human growth hormone binding to its receptor. X-ray structures of the bound complex were available, and residues of hGH in the interface were known. In the first paper above, alanine scanning was used to replace each residue of human growth hormone buried in the interface with the following results:</p><p>19 Most mutated proteins could be recovered as well behaved soluble proteins. Only a subset of the buried residues, concentrated in the center of the interface, contributed significantly to the strength of the binding. Most mutations that altered binding did so by withdrawing a favorable contact and increasing the off rate. Mutations that decreased the on rate generally withdrew a charged side chain. This is interpreted as meaning that the protein had evolved an electric dipole that would tend to rotate it towards a productive orientation during an impending collision with the receptor.</p><p>Other scanning strategies</p><p>Alanine scanning is often reduced to substitution of Ala at every charged residue (assuming that these have to be on the surface). Alanine scanning is used to assign functions to different domains, and to create tentative sets of active site residues. A variation called Cys scanning additionally allows one to covalently attach chemical moieties at the substituted positions. Trp scanning (PNAS 92(17):7946-50 </p><p>20 (1995)), has been used to characterize proteins with multiple transmembrane helixes on the principle that Trp residues will be tolerated on surfaces exposed to the lipid environment, but not elsewhere.</p><p>Saturation Mutagenesis</p><p>Saturation mutagenesis can generate a large collection of clones. It is helpful to have a selection to sort out the desired phenotypes. In a follow up of the above paper (in Loweman and Wells, 1993. J. Mol. Biol. 234:564-578.) recombinant phage display was used to saturate different sets of residues and then select variants that bound more tightly to the receptor. Then these regions were artificially combined to make even more strongly binding versions of human growth hormone.</p><p>General lessons from this study were:</p><p> In order to get a big enough difference to discriminate from wild type, one has to saturate at 4 or 5 positions at once. After a tight binding variant is found (and sequenced), it is possible to test each of the varied residues one at a time using site directed mutagenesis.</p><p>21 Each varied residues contributes only a small improvement. The improvements yielded by the individual residue changes generally add up to the total effect for all the varied residues together. i.e. if we define Kd = Kd (wt)/Kd (mut), then for mutant residues A, B, C: Kd(ABC) usually was ~= Kd(A) * Kd(B) * Kd(C) Or if G = -RT ln (Kd(wt)/Kd(mut)) then for mutant residues A, B, C: G(ABC) ~= G(A) + G(B) + G(C)</p><p>A cataloging approach to saturation mutagenesis</p><p>Saturation mutagenesis may be tied to a screen instead of a selection. In these cases, the screened mutants with a specific phenotype are sequenced, and conclusions are drawn the collection. In a classic study (Lim and Sauer, nature 339:31[1989]) the hydrophobic core residues of lambda repressor were altered and screened for the capability of the repressor to still fold and carry out its function of resisting infection by a lambdoid phage. The result of the study is that many different packing arrangements of the hydrophobic core are satisfactory as long as the total volume is about right.</p><p>22 The mutagenized repressors were driven by an inducible tac promoter. Some were able to repress the incoming lambda phage when expressed at the basal level, and others could only successfully repress if the tac promoter was induced. Therefore, the mutants were divided into three classes: totally functional (similar to the wild type); partially functionally (requires over expression); and nonfunctional. 30-100 mutants of each class were sequenced.</p><p>The technical aspect of the experiment that I would like to emphasize is the placement of the selection before the sequencing. Only about 1% of the mutants survived the screen. If they had sequenced at random, and then tested the phenotype later, they would have obtained most of the data from the nonfunctional set. The application of the selection first allowed them to pick similar numbers of mutants to sequence from each set.</p><p>Mutagenesis to add a label to a protein.</p><p>Physical-chemical studies of proteins often make use of fluorescence of endogenous trp residues to report on the conformation of the protein. With site-specific mutagenesis, one might arrange for the placement of trp residues in ideal positions for the anticipated study. This may involve both removing endogenous trp residues and adding new ones. (JBC 269(11):7919-25, 1994). Note that one could use difference spectra to cancel out the signal from other trp residues present in both the wild type and mutant proteins.</p><p>Other kinds of reporter groups may be added to the protein. One relatively flexible strategy is to add a Cys at a predetermined position, and to chemically modify the Cys to attach some other moiety.</p><p>Other Objectives</p><p>1. Investigating modifications (phosphorylation, glycosylation, palmitylation, etc.)</p><p>One can remove putative modification sites identified by sequence motifs to see if the resulting protein is less modified. Further one can ask if the unmodified protein produced in this way has altered function.</p><p>2. Investigating genetic disease:</p><p>Now that defective genes of all descriptions are being isolated by positional cloning, one is often confronted with a defective gene with several differences from the "wild type". Mutagenesis may be conducted to isolate each of the differences to distinguish the actual defect from inconsequential polymorphisms. This will require an in vitro assay or a cell culture assay to act as a surrogate for the disease phenotype. One may wish to introduce other mutations into the target gene to see how specific the disease phenotype is with respect to different mutations. Site specific mutagenesis may also be used to introduce the same defect into a mouse by gene replacement.</p><p>23 3. Identify a compartmentalization signal.</p><p>4. Assign functions to particular protein domains.</p><p>5. Delete domains for functional assignment or to stabilize for crystallography.</p><p>6. Add a tag.</p><p>24 Study Questions</p><p>1. It is proposed that the following network of hydrogen bonds is involved in stabilizing substrate binding in a particular enzyme. How could you test this by in vitro mutagenesis? substrate--HO Ser118 main chain NH--OH Thr46</p><p>2. What would be a good method if you wanted to map monoclonal antibody epitopes on a protein?</p><p>3. A protein has 5 trp residues. You try to change 4 of the trp residues to Ala so that the 5th can be used as a probe of conformational change. This results in a protein that aggregates intractably. How might you approach solving this problem?</p><p>4. A particular 200 bp promoter fragment is sufficient to confer a heat shock response on a reporter gene. Can you think of a reason why a complete set of linker scanning mutants might fail to identify the heat shock response element?</p><p>5. How could you use the fact that human ras will complement yeast ras to carry out saturation mutagenesis looking for other mutations (besides val 119) that produce an activated ras? (Yeast ras functions in a pathway that signals vegetative growth under conditions of good nutrition and sporulation under conditions of poor nutrition).</p><p>Last revised 4/9/2011 - Steve Hardies</p><p>25</p>
Details
-
File Typepdf
-
Upload Time-
-
Content LanguagesEnglish
-
Upload UserAnonymous/Not logged-in
-
File Pages25 Page
-
File Size-