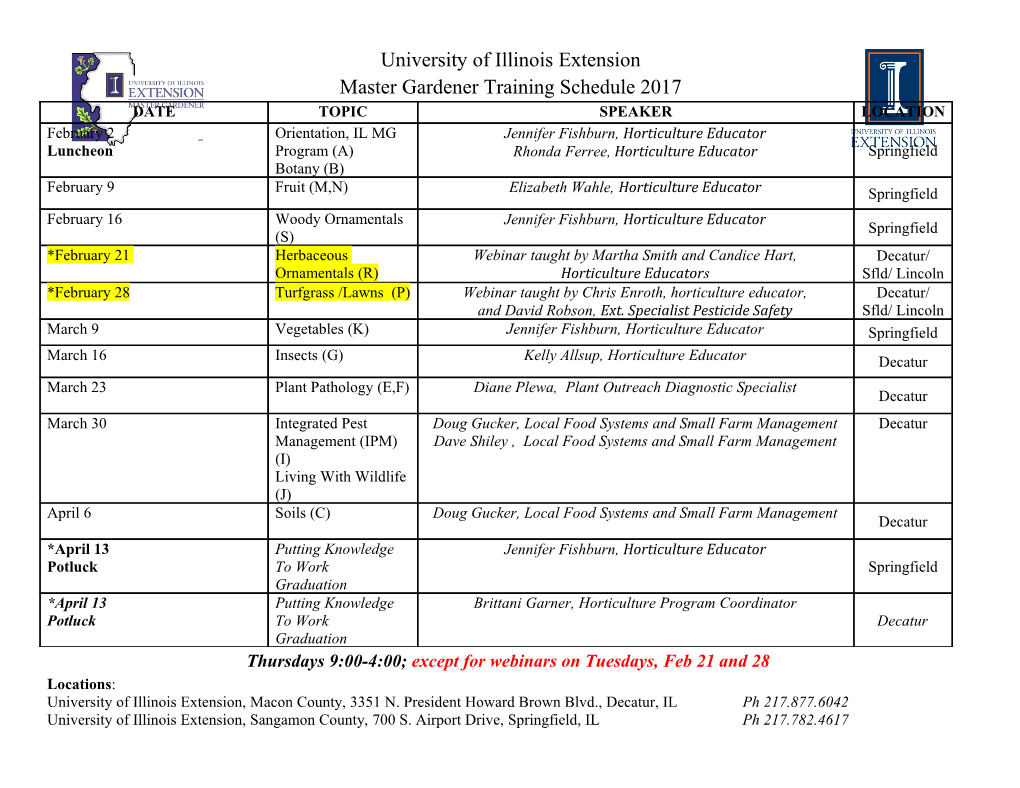
% !" #$%&!$! "!%" &!" '()(*()) ) +,!$$-$ ..V 2 ISBN C D E E F C F ! "# $ " % # "$ &'()" "*+,'-(. "/ GHJ K LK MM FM O P Till Sven och Freja List of Papers This thesis is based on the following published papers and manuscripts, which are referred to in the text by their Roman numerals. I Havelius, K. G. V., Su, J.-H., Feyziyev, Y., Mamedov, F. and Styring, S. (2006) The spectral resolution of the split EPR- signals induced by illumination at 5 K from the S1, S3 and S0 states of photosystem II, Biochemistry, 45:9279–9290. II Su, J.-H., Havelius, K. G. V., Feyziyev, Y., Mamedov, F., Ho, F. M. and Styring S. (2006) Split EPR signals from photosys- tem II are modified by methanol, reflecting S state dependent binding and alterations in the magnetic coupling in the CaMn4 cluster, Biochemistry, 45:7617–7627. III Havelius, K. G. V. and Styring, S. (2007) pH dependent compe- tition between YZ and YD in photosystem II probed by illumina- tion at 5 K, Biochemistry, 46:7865–7874. IV Su, J.-H., Havelius, K. G. V., Ho, F. M., Han, G., Mamedov, F. and Styring, S. (2007) Formation spectra of the EPR split signal from S0, S1 and S3 States in photosystem II induced by mono- chromatic light at 5 K, Biochemistry, 46:10703-10712. V Han, G., Ho, F. M., Havelius, K. G. V., Morvaridi, S. F., Ma- medov, F. and Styring, S. (2008) Direct quantification of the four individual S states in photosystem II using EPR spectros- copy, Biochim. Biophys. Acta, 1777:496-503. VI Havelius, K. G. V., Ho, F. M., Su, J.-H., Han, G., Mamedov, F. and Styring, S. (2009) The same origin of the split EPR signal induced by visible or near-infrared light at liquid helium tem- perature from the S3-state of photosystem II, Manuscript VII Sjöholm, J., Havelius, K. G. V., Mamedov, F. and Styring, S. (2009) The S0 state of water oxidizing complex in photosystem II: pH dependence of the EPR split signal, induction and mechanistic implications, Manuscript VIII Ho, F. M., Havelius, K. G. V., Sjöholm, J. and Styring, S. (2009) Investigation and characterisation of EPR split signals in the native S2 state of photosystem II, Early manuscript Reprints were made with permission from the respective publishers. The following publication is not included in this thesis and deals with the work of the master degree thesis (Filsofie Magister) published under my maiden name Sigfridsson. IX Sigfridsson, K. G. V., Bernát, G., Mamedov, F. and Styring, S. (2004) Molecular interference of Cd2+ with photosystem II, Biochim. Biophys. Acta, 1659:19–31. Contents Introduction.....................................................................................................9 1. Why do we study Photosystem II?.........................................................9 1.1. We need energy...............................................................................9 1.2. Be biomimetic ...............................................................................10 2. Oxygenic Photosynthesis .....................................................................11 2.1. Light reactions..............................................................................11 2.3. Dark reactions (not light-dependent) ...........................................14 4. Photosystem II......................................................................................14 4.1. Structure .......................................................................................14 4.2. Redox-active cofactors..................................................................15 5. The Oxygen-Evolving Complex ..........................................................18 5.1. Metal organization........................................................................18 5.2. The catalytic S-state cycle ............................................................19 5.3. Protein ligands, channels and water binding ...............................21 6. The two redox-active tyrosines ............................................................23 • • 6.1. The electronic structure of YZ and YD .........................................23 6.2 Oxidation and reduction................................................................24 • 7. Metalloradical EPR signals I – Mn-YZ interaction..............................25 7.1. Background to the split EPR signals ............................................25 7.2. Inactive PSII .................................................................................26 7.3. Active PSII ....................................................................................27 8. Metalloradical EPR signals II – Induction pathways ...........................32 • + 8.1. YZ formed via P680 ....................................................................32 • 8.2. YZ formed via the “NIR pathway” ...............................................34 9. Metalloradical EPR signals III – Methanol and pH dependence of the OEC................................................................................................39 9.1. Methanol.......................................................................................39 9.2 pH ..................................................................................................42 10. Conclusions and future perspectives ..................................................51 10.1. Concerning YZ oxidation.............................................................51 10.2. Concerning the metalloradical EPR signals ..............................51 Acknowledgement ........................................................................................52 Svensk sammanfattning ................................................................................55 References.....................................................................................................58 Abbreviations ATP adenosine triphosphate Car !-carotene Chl chlorophyll DCMU 3-(3,4adichlorophenyl)-1,1-dimethylurea EPR electron paramagnetic resonance ESE electron spin echo ESEEM ESE envelope modulation EXAFS extended X-ray absorption fine structure FAD flavin adenine dinucleotide FNR FAD-containing ferredoxin-NADP+ reductase GAP glyceraldehydes-3-phosphate HisZ histidine 190 on D1 HYSCORE hyperfine sublevel of correlation, a pulsed EPR technique LHC light harvesting complex MeOH methanol NADPH nicotinamide adenine dinucleotide phosphate NIR near-infrared (700-900 nm) OEC oxygen evolving complex PCET proton coupled electron transfer Pheo pheophytin PQ plastoquinone PSI photosystem I PSII photosystem II QA primary quinone acceptor in PSII QB secondary quinone acceptor in PSII Ru5P ribulose-5-phosphate RuBisCO ribulose biphosphate carboxylase-oxygenase XANES X-ray absorption near-edge structure XES X-ray emission spectroscopy YD tyrosine 160 on D2 YZ tyrosine 161 on D1 Introduction In this thesis I will present new useful probes for one of the most fascinating enzyme mechanisms in the world, namely light-driven water oxidation. I be- lieve that the metalloradical EPR signals presented here will have great poten- tial in the future to help elucidate the mechanism of the catalytic reaction. 1. Why do we study Photosystem II? At the dawn of life on Earth there was no oxygen in the atmosphere. Photo- trophic cyanobacteria evolved about three billion years ago, capable of using the energy of sunlight to extract electrons from the abundant supply of water and use these electrons for production of carbohydrates out of carbon dioxide. Oxygen was released as a by-product and started to accumulate in the atmos- phere, thus changing the evolutionary pressure for all organisms. It is because of oxygenic photosynthesis that we, the aerobic life form, could evolve and populate the planet. Via respiration we utilize the oxygen released in the light reaction of photosynthesis to extract the energy stored in glucose, a product of the dark reaction of photosynthesis. Photosystem II is the enzyme that catalyzes the remarkable light-driven chemistry of water splitting. 1.1. We need energy Energy is the costs to be ordered in a universe that constantly strives to in- crease disorder. By breaking chemical bonds of molecules like sugar and the carbohydrates of gasoline, energy is released and this can be used for making new compounds in cells or when driving a car. Photosynthesis is the process by which energy from the sun is stored in chemical bonds. Mankind has al- ways thrived from this energy by eating plants to fuel her body or by burning firewood, oil, coal or natural gases to for example keep warm. In this way we can say that the energy used for all human activities today almost exclusively comes from the sun, but mostly in an indirect way via the use of fossil fuels (~80% of the global energy consumption comes from fossil fuels (1)). The oil and coal reserves we use today as fossil fuels were deposited dur- ing the age of the big fern forests 300-350 million years ago (Carboniferous), but these resources are limited and will eventually run out. The burning of fossil fuels for human activities, which on a geological time line can be con- 9 sidered instantaneous, releases most of
Details
-
File Typepdf
-
Upload Time-
-
Content LanguagesEnglish
-
Upload UserAnonymous/Not logged-in
-
File Pages74 Page
-
File Size-