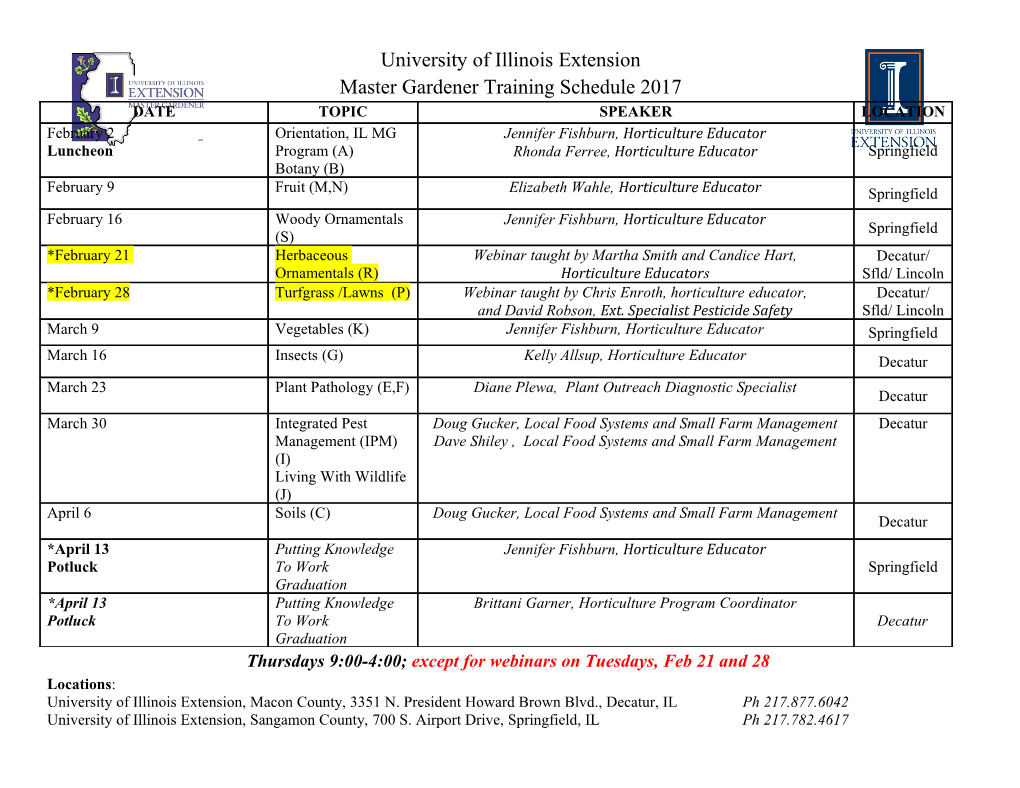
<p> 3.1 Transit Orbits Kassandra De Voto</p><p>Nomenclature Earth Atmospheric Entry Theoretical atmosphere limit, 200 km altitude above Earth LEO Low Earth orbit (300 km altitude) LMO Low Mars orbit (200 km altitude) Mars Atmospheric Entry Theoretical atmosphere limit, 100 km altitude above Mars MIDAS Mission Design and Analysis Software ΔV Delta vee, required velocity change in units of length/time</p><p>3.1.1 Introduction Before we can do anything on Mars, we first have to get there. How we choose to do so can affect virtually every aspect of mission and vehicle design, from how long we are on Mars to the size and characteristics of the ship we can take there. In order to design the “best” mission, we look at how changing a single aspect of the trajectory affects other areas of the mission, and how multiple changes interrelate. Then, using the MIDAS optimization software developed by JPL,1 we find the trajectories that meet all given mission parameters, and choose the one we feel would be most cost effective.</p><p>3.1.2 Trajectory Determination Determining an interplanetary trajectory is a complex process involving a number of different variables and effects. Besides the obvious requirements that we begin at the launch site and end up where we want to go, we must also choose a trajectory that has a launch velocity and maneuvers we can actually produce, an arrival velocity that our vehicle (and crew) can withstand, and for manned missions, a flight time and path that the crew can survive. Each of these significantly affect our mission design, and each depends on the others. In order to produce a “minimum cost” mission that accomplishes all our goals, we have to make trade-offs between these different requirements.</p><p>3-1-1 This report is merely a feasibility study, so a number of minor effects and requirements were either neglected or “black boxed;” assumed to have a specific value. These include such effects as orbital correction maneuvers required due to errors from thruster misalignment, solar wind effects, etc., or determining the launch window from the surface of the planet to the low orbit from which we insert into the transit trajectory. While knowing these things would be required for an actual mission, they have little or no effect on design at this high a level. To produce the actual trajectories, we used the MIDAS software developed by the Jet Propulsion Laboratory, which produces an optimized patched-conic trajectory based on a user input file. For examples of the input files and their results, see Appendix 3.1.1. For each flight, we tried optimizing the trajectory around different parameters, including time of flight (TOF), launch velocity, and arrival velocity. We compared the effects of optimizing each parameter, and chose the one we felt was best. The specifics of each trajectory we chose and the reasoning behind them are explained in the following sections.</p><p>3.1.3 Habitat Trajectory The mission parameters make the habitat trajectory the most limited, so we choose this one first and design the others around it. For crew safety, we require that there be an abort-to-Earth scenario, while limiting ourselves to NASA’s recommendation of a TOF to Mars of 180 days or less, with a total flight time of less than 2.2 years, or 800 days, in the case of an abort.2 The only trajectory which meets all these requirements in the next two decades is an Earth-Mars-Venus-Earth free return with an optimal launch date of 14 January 2014.3 Figure 3.1.1 shows this trajectory, viewed as if looking at the solar ecliptic plane, while Table 3.1.1 gives the launch and arrival specifics. </p><p>3-1-2 H a b i t a t T r a j e c t o r y 2</p><p>1 . 5 E a r t h L a u n c h 1</p><p>0 . 5 V e n u s )</p><p>U E a r t h f l y - b y A</p><p>( 0</p><p>A r r i v a l </p><p>Y - 0 . 5</p><p>- 1</p><p>M a r s - 1 . 5 A r r i v a l </p><p>- 2 - 2 - 1 . 5 - 1 - 0 . 5 0 0 . 5 1 1 . 5 X ( A U )</p><p>Fig. 3.1.1: Trajectory of Habitat module</p><p>Table 3.1.1 Specifics of Habitat trajectory Launch ΔV to LEO 7.31 km/s Transit Insertion ΔV 3.78 km/s Launch V∞ 3.61 km/s Mars Arrival V∞ 6.77 km/s Mars Atmospheric Entry Velocity 8.39 km/s Mars Atmospheric Entry Flight Path Angle 9.5o Earth Arrival V∞ (Abort) 3.84 km/s Earth Atmospheric Entry Velocity (Abort) 11.65 km/s Earth Atmospheric Entry Flight Path Angle (Abort) 9.5o Launch Date 14 January 2014 TOF to Mars 172 days Mars Arrival Date 04 July 2014 TOF to Venus (Abort) 427 days Total TOF (Abort) 793 days Earth Arrival Date (Abort) 17 March 2016</p><p>3-1-3 It is possible to launch earlier or later than the optimal date of 14 January and still have an EMVE free return; however, any other date requires a small correctional maneuver (20~30 m/s) in deep space, applied about 200 days after the Venus flyby. This launch window is limited to about 15 days before and 10 days after 14 January; beyond that, the maneuvers required to achieve an EMVE trajectory become prohibitively expensive. The only other maneuver required by this trajectory, besides basic course corrections, is one to get us off the free return and on an approach to Mars that gives us the entry flight path angle necessary for aerobraking. Applying this maneuver a day out from Mars, at the same time we cut the tether, allows us to use the ΔV from the tether spin to assist us, as well as sending the now unnecessary NTR away from the planet. Theoretically, we could also use the ΔV from tether spin-up to assist us, at the other end of our trajectory, to do the transit orbit injection. However, there are a number of problems with trying to do this. For one thing, in order to affect the velocity of the center of mass of the vehicle, we would have to use decoupled thrusting. The most efficient decoupled thrusting would be on the lighter weight, in this case the NTR, since thrusting on the heavier weight (the Hab) is actually more fuel-expensive than coupled thrusting. However, both crew and mission safety dictate that we have thrusters on the Hab section, in case of tether failure and for landing control. Having two systems with enough fuel to do the entire job is simply wasted tonnage. Secondly, using decoupled thrusting to impart a translational velocity change means we can only thrust for a very short time, when our thrusters are pointing in the right direction – if they ever do. This means we need a very large number of very short burns over a long time, which can lead to a buildup of pointing error as well as increasing mission risk every time we have to do a burn. Also, using the decoupled spin to help get the final injection ΔV requires doing the injection in two stages: the NTR burn and then the spin-up, with its associated velocity change. This means that we are not on the free return trajectory when we leave Earth orbit – and if something goes wrong with either thrusters or tether early on, we may not be able to get on it, even if we fix the problem later.</p><p>3-1-4 Basically, while decoupled thrusting for spinup/spindown is a possibility, it adds complications and risks that outweigh the possible benefits. A more detailed design might find ways around these problems, but for a study of this level we feel that coupled thrusting is effective enough for our needs.</p><p>3.1.4 Earth Return Assembly Trajectory: Earth to Mars Since the Earth Return Assembly (ERA) contains the in-situ propellant production plant, it needs to arrive on Mars early enough to produce all the propellant needed for the return trip before the launch of the manned Hab module. The Hab launches on 14 January 2014; the ERA needs to arrive at least a year prior to that. The minimum cost trajectory that meets that requirement is a launch in November of 2011, arriving on 11 September 2012, 16 months before the manned launch. Specifics of the orbit are given in Table 3.1.2 and Fig. 3.1.2. Launching the entire ERA from the surface of Mars is too expensive, so the Earth Return Vehicle (ERV) itself is remaining in orbit around Mars, while only the Mars Launch Vehicle (MLV) and a Crew Transfer Vehicle (CTV) are sent to the surface. We insert into LMO using aerocapture, as described in the aerodynamics section, so the only propulsive maneuvers needed by the ERV are any course corrections necessary for the desired entry flight path angle, and a maneuver to circularize the orbit after aerobraking and separation from the landing assembly.</p><p>3-1-5 E R V L a u n c h - E a r t h t o M a r s 1 . 5</p><p>1</p><p>0 . 5 E a r t h </p><p>) L a u n c h U A (</p><p>0</p><p>Y</p><p>- 0 . 5</p><p>- 1</p><p>M a r s A r r i v a l </p><p>- 1 . 5 - 2 - 1 . 5 - 1 - 0 . 5 0 0 . 5 1 X ( A U )</p><p>Fig. 3.1.2: Earth-to-Mars trajectory of Earth Return Vehicle</p><p>Table 3.1.2 Specifics of ERV Earth-Mars trajectory Launch ΔV to LEO 7.31 km/s Transit Insertion ΔV 3.60 km/s Launch V∞ 2.99 km/s Mars Arrival V∞ 2.71 km/s Mars Atmospheric Entry Velocity 5.64 km/s Launch Date 09 November 2011 TOF to Mars 307 days Mars Arrival Date 11 September 2012</p><p>3-1-6 3.1.5 ERV Return: Mars to Earth The driving force behind this mission is the exploration of Mars, which requires that we spend a reasonable amount of time on its surface. With the arrival of the crew on 04 July 2014, the next available launch window is in December of 2015, a year and a half later – plenty of time to wander around. Originally, we were planning to limit the TOF of the return trip to 180 days, but doing so drove up both the launch ΔV and the arrival velocity. A longer flight time of 230 days produces a more reasonable cost in terms of ΔV, but we have to include a tether to offset the effects of prolonged weightlessness. The inclusion of the tether makes things interesting when we first launch from Mars. The ERV is met in orbit by the CTV and MLV launched from the surface, which dock on one side of the ERV. The whole assembly then inserts into the transfer orbit, at which point the spent propulsion system can be used for the tether counterweight. However, the MLV stage is attached such that the CTV is between it and the ERV, which makes things awkward for the separation of the CTV at Earth arrival. Therefore, we have to somehow get the MLV to the other side of the ERV before we deploy the tether. Doing so is very simple; we simply undock the MLV, spin the ERV-CTV assembly 180 degrees, then redock the MLV to the ERV. Although easily done, this does require maneuvering on the part of both ERV and MLV.</p><p>3-1-7 E R V R e t u r n T r a j e c t o r y - M a r s t o E a r t h 1</p><p>M a r s L a u n c h 0 . 5</p><p>0 ) U A (</p><p>E a r t h</p><p>Y A r r i v a l - 0 . 5</p><p>- 1</p><p>- 1 . 5 - 2 - 1 . 5 - 1 - 0 . 5 0 0 . 5 1 X ( A U )</p><p>Fig. 3.1.3: Mars-to-Earth trajectory of Earth Return Vehicle</p><p>Table 3.1.3 Specifics of Mars-Earth ERV trajectory Launch ΔV to LMO 3.21 km/s Transit Insertion ΔV 2.08 km/s Launch V∞ 2.60 km/s Earth Arrival V∞ 4.22 km/s Earth Atmospheric Entry Velocity 11.79 km/s Earth Atmospheric Entry Flight Path Angle 9.5o Launch Date 26 December 2015 TOF to Earth 230 days Earth Arrival Date 12 August 2016</p><p>3-1-8 3.1.6 Communication Availability Both manned and unmanned missions require the ability to communicate with Earth, preferably with clarity. One thing that can interfere with interplanetary communications is the Sun, either because of a blackout, where the Sun lies between the Earth and the spacecraft, or a whiteout, where the Sun lies directly behind either spacecraft or Earth, as seen from the other. Either situation results in communication loss, and in signal degradation for a few degrees to either side of the event. For the duration of this mission, there are only three such occurrences, all between Earth and Mars. The transit orbits for all three vehicles, including the free- return abort, are such that we can maintain communication with Earth at all times. The dates of comm loss are shown in Table 3.1.4.</p><p>Table 3.1.4 Dates of Communication Loss 27 Mar - 05 Apr 2013 Blackout (Sun between Mars – Earth) 26 Apr - 04 May 2014 Whiteout (Sun behind Earth) 26 May - 04 Jun 2015 Blackout (Sun between Mars – Earth)</p><p>3.1.7 NTR Disposal Since we are separating the Hab module from the NTR before we get to Mars, the NTR, which we are using for a counterweight, will follow the free-return trajectory and eventually crash on Earth, unless we alter its course. To do this, we use the ΔV created by the severing of the tether to alter the gravity assist at Mars, then apply a propulsive ΔV of about 160 m/s 30 days later, to alter the heliocentric orbit to one that will not approach any planet. A similar maneuver, of about ~240 m/s, produces the same effect in the case of an abort, where we cut the tether approaching Earth. The ERA does not have a tether, so the NTR is simply separated from it after it enters Mars orbit, and remains in orbit after the ERV leaves. The ERV itself, returning to Earth, does not have an NTR, but uses the spent MLV stage as a counterweight. This separates from the CTV upon Earth arrival, and burns up on re-entry, so does not need to be disposed of.</p><p>3-1-9 References 1) Sauer, Carl, “Preliminary Draft of User’s Guide to MIDAS,” Advanced Projects Group, Jet Propulsion Laboratory, March 1991.</p><p>2) Hoffman, Steven J. and Kaplan, David L., “Human Exploration of Mars: The Reference Mission of the NASA Mars Exploration Study Team,” http://exploration.jsc.nasa.gov/marsref/contents.html, July 1997.</p><p>3) Okutsu, Masataka and Longuski, James M., “Mars Free Returns Via Gravity Assist from Venus,” AIAA Journal, ????.</p><p>3-1-10</p>
Details
-
File Typepdf
-
Upload Time-
-
Content LanguagesEnglish
-
Upload UserAnonymous/Not logged-in
-
File Pages10 Page
-
File Size-