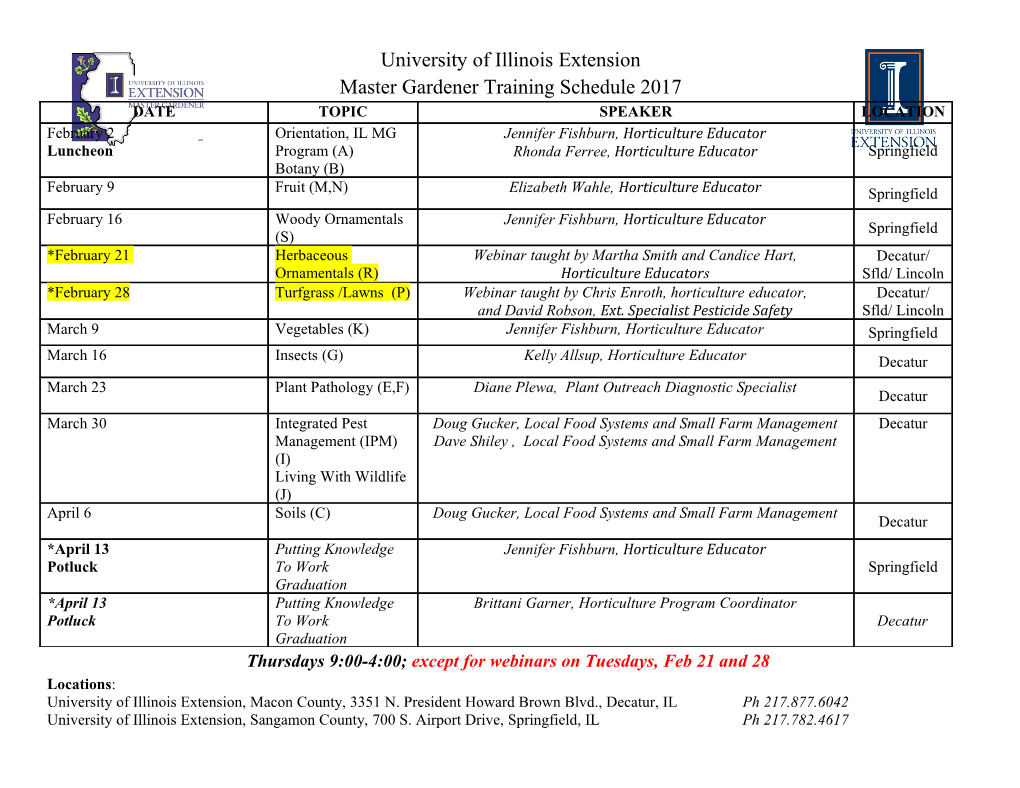
<p> Supplementary Methods and Results</p><p>Supplementary Methods</p><p>Sample isolation and NK cell activation</p><p>DNA and RNA from NKCL cases, NK-cell lines, normal NK cells and tonsil were isolated using AllPrep DNA/RNA mini kit (Qiagen Inc., Valencia, CA). </p><p>Primary human NK cells were isolated from peripheral blood lymphocytes (PBL) using a human NK cell isolation kit (Miltenyi Biotec, Auburn, CA) as described previously (1). The purity of NK cells was evaluated by CD56-APC (BD Biosciences, San Jose CA) and CD3-PE </p><p>(BD Biosciences) double staining according to manufacturer’s recommendations, and samples with >95% CD56+CD3- cells were used in the experiments. Resting NK cells were cultured in the presence of 100 IU of IL2 for 1, 2 or 3 days to obtain activated NK cells. NK cell activation by coculturing PBLs with engineered K562 cells for 15 days was performed as described previously (1). DNA was isolated from normal tonsil tissue as a control in the MSCC analysis without cellular fractionation.</p><p>GEP analysis</p><p>GEP was performed on malignant tumor samples or normal NK cells (resting NK cells, NK cells activated with IL2 for 1 or 3 days, NK cells activated with engineered K562 cells for 15 days) using HG U133Plus2 chips (Affymetrix Inc., Santa Clara, CA) according to the manufacturer’s recommendations. The raw CEL files were uploaded into BRB-array tools </p><p>(version 4.2.1). The Robust Multiarray Average (RMA) method was used to normalize the CEL gene expression values. Genes whose expression did not differ by at least 1.5 fold from the median in at least 20% of the cases were excluded from the analysis. Normalization and filtering of the microarrays from cases (n=7) or cell lines (n=2) with methylation analysis were performed</p><p>1 along with additional arrays which include NK cell lines (n=6), NK-LGLL cases (n=5), NKCL cases (n=25), IL2-activated NK cells (n=4), and IL12-activated CD4+ (n=5) and CD8+ T (n=5) cells. The GEPs of most of these additional samples have been reported in previous studies (2, </p><p>3). The microarray data is available in the Gene Expression Omnibus database of NCBI through the accession number GSE19067. 18 of 25 NKCL cases are historical cases without MSCC data; they were used here only for normalization and filtering of the microarrays. </p><p>Reduced representation bisulfite sequencing (RRBS)</p><p>RRBS experiment was performed in the City of Hope Integrative Genomics Core Facility as described previously (4). The procedure is briefly as follows: Genomic DNA from NK92 and </p><p>KHYG1 cell lines and 3 day IL2-activated normal NK cells were digested with MspI (NEB, </p><p>Ipswich, MA), a restriction enzyme that cleaves both methylated and unmethylated CCGG sites. </p><p>After digestion, Illumina TruSeq DNA LT sample prep kit was used for end repair. A tailing and ligation were performed using Illumina TruSeq DNA library prep kit. EZ DNA Methylation </p><p>(Zymoresearch, Irvine, CA ) kit was then used for bisulfite conversion. Bisulfite converted DNA was amplified with PfuTurbo Cx Hotstart DNA Polymerase (Agilent, Santa Clara, CA).Paired- end 100bp sequencing was performed with Illumina Hiseq2500 platform. The data quality was checked with FastQC tool. </p><p>Raw reads were aligned to hg19 using Bismark program (5), and bedGRAPH files were generated. These files were then used as input for Bi-Seq, a package in Bioconductor (6). Default parameters were applied to identify significantly differentially methylated regions (DMR) between each NK-cell line and normal NK-cell sample (NKd3). The RRBS sequencing data will be submitted to SRA database of PubMed</p><p>Pyrosequencing</p><p>2 Bisulfite treatment was carried out using 1 µg of sample genomic DNA and the EZ DNA </p><p>Methylation-Direct kit (Zymo Research, Orange, CA). This process deaminates unmethylated cytosine residues to uracil leaving methylated cytosine residues unchanged. To perform PCR reactions, 42 ng of bisulfite-modified DNA was used as template. The PCR reactions were performed in a total volume of 25 µl for 35 cycles using 1.0U of FastStart Taq DNA Polymerase</p><p>(Roche Inc., Indianapolis, IN) , MgCl2 solution (3.5 mM), dNTP’s (0.2 mM), forward primer </p><p>(0.24 uM), reverse primer (0.18 µM), with denaturation at 95 ºC for 30 seconds, annealing for 45 seconds, and extension at 72 ºC for 1 minute. For a positive (high methylation level) control, human lymphocyte genomic DNA (Roche Inc., Indianapolis, IN) was methylated using M. SssI</p><p>(CpG) methylase kit (New England Biolabs, Ipswich, MA). Methylated DNA (1000 ng) was treated with sodium bisulfite as described above. Sodium bisulfite treated Roche human lymphocyte genomic DNA (1000 ng) served as a negative (low methylation level) control. All </p><p>PCR products were separated by electrophoresis on 0.8% agarose gel, stained with ethidium bromide, and visualized for appropriate and pure product before proceeding with all analyses using a Bio-Rad Laboratories (Hercules, CA) Gel-Doc UV illuminator. Methylation percentage of each CpG was determined using a Pyromark Q24 pyrosequence (Qiagen,Valencia, CA) according to manufacturer’s recommendations. The primers used for pyrosequencing are as follows: BIM-1 forward: 5’-TTTGTTTTTTTAAATGTTTGATTTTGA-3’; BIM-1 reverse: 5’-</p><p>ACCCRATCCACACRAATCCCCACCTCC-3’; BIM-1 sequencing: 5’-</p><p>TAAGGTAAATATTTTTAAATT-3’; BIM-2 forward: 5’-</p><p>GTTTTTGTTTGTTTTGTAGGAGTTTTG-3’; BIM-2 reverse: 5’-</p><p>CAACCCTAAAACAACAAATCCCAAAAAAC-3’; BIM-2 sequencing: 5’-</p><p>AGGTTTTTTTTTGATTATTTGG-3’; DAPK1-1 forward: 5’-</p><p>3 TATTATTTATTTGTTTTATTAGAATGTAGG-3’; DAPK1-1 reverse: 5’-</p><p>ACATTACTAAACTACCCTCTAACTAAATTCT-3’,DAPK1-1 sequencing: 5’- </p><p>TTGTTTTATTAGAATGTAGGTT-3’; DAPK1-2 forward: 5’- </p><p>YGTGGGTGTGGGGYGAGTGGGTGTGTG-3’; DAPK1-2 reverse: 5’-</p><p>ACTCCRAAACAACCTCTCRACTCCTTAC-3’; DAPK1-2 sequencing: 5’-</p><p>GAGTGGGTGTGTGYGGGGTG-3’; ASNS -1 forward: 5’- </p><p>ATGTTTTAGGGAATTAGGATAGAAAGGT-3’; ASNS-1 reverse: 5’-</p><p>TCAACCCRCCACATCACCCTAACCTACTT-3’; ASNS-1 sequencing: 5’-</p><p>TTTTTTYGTTAGTTGTTAGGT-3’; ASNS -2 forward: 5’-</p><p>GGTTGAGGTTAGGGATGTGGATAGTTT-3’; ASNS -2 reverse: 5’-</p><p>TCCCTAATTATCAAAACAACAAATAACCT-3’; ASNS sequencing: 5’-</p><p>TTATATYGATTTGGTTTTTGTA-3’.</p><p>Quantitative methylation specific PCR (q-MSP)</p><p>1 µl of bisulfite-treated DNA was used for methylation-specific q-PCR (MSP) as described earlier (1) to assess promoter methylation in NKCL cases (n=8) and NK-cell lines (n=7). M.SssI- methylated human genomic DNA (D5015, Zymo Research, Irvine, CA) and human non- methylated DNA (D5014-1, Zymo Research, Irvine, CA) were used as the positive and negative controls, respectively. 3-day IL2-activated peripheral blood NK cells were used as the normal standard for comparison. The primers used for the q-MSP analysis were designed using </p><p>Methprimer (7) and are as follows: BIM-qMSP-M forward: 5’-</p><p>GAAGATGCGTAGTTTGTTGATTC-3’; BIM-qMSP-M reverse: 5’-</p><p>CTATACCCTAAATTTCTAAACCGCT-3’; BIM-qMSP-U forward: 5’-</p><p>AGGAAGATGTGTAGTTTGTTGATTTG-3’; BIM-qMSP-U reverse: 5’-</p><p>4 CTATACCCTAAATTTCTAAACCACT-3’; ASNS-qMSP-M forward: 5’-</p><p>TTTTTTCGGAGTAGTTTTAGGGTAC-3’; ASNS-qMSP-M reverse: 5’-</p><p>ATCGATATAAACGCCAACGAC-3’; ASNS-qMSP-U forward: 5’-</p><p>TTTTTTTTGGAGTAGTTTTAGGGTATG-3’; ASNS-qMSP-U reverse: 5’-</p><p>CCAAATCAATATAAACACCAACAAC-3’; DAPK1-qMSP-U forward*: 5’-</p><p>GGAGGATAGTTGGATTGAGTTAATGTT-3’; DAPK1-qMSP-U reverse*: 5’-</p><p>CAAATCCCTCCCAAACACCAA-3’; SOCS6-q-MSP-U forward: 5’-</p><p>GGATAGGGATTGTAGTTGTTAAATTTG-3’; SOCS6-q-MSP-U reverse: 5’-</p><p>TTTTCCTTTAATACTTTCCCTCTAACA-3’; ZFHX3-q-MSP-U forward: 5’-</p><p>TGGGAATAAAGTTTAGGTTTTTTTG-3’; ZFHX3-q-MSP-U reverse: 5’-</p><p>AACCTCAAACTCCTTTTAATAACCAA-3’; TET2-q-MSP-M forward *: 5’-</p><p>CGAGAGtTTGGGCGGtC-3’; TET2-q-MSP-M reverse *: 5’-</p><p>GTCTaTTCTCATCACTCAaCGaaaCCG-3’; CD300A-q-MSP-M forward: 5’-</p><p>GGGTTTTTTATTAGGAGGAGATTC-3’; CD300A-q-MSP-M reverse: 5’-</p><p>TAATTAAAACTACAAATTCTTCGAC-3’; IL12Rb2-q-MSP-M forward: 5’-</p><p>CGTTTTTAGAGTTGAATTGAGAAGC-3’; IL12Rb2-q-MSP-M reverse: 5’-</p><p>AAAACTAACGACGAATAAAAATCGA-3’; TLE1-q-MSP-U forward: 5’-</p><p>AAGTTGGAAAGTTAAGTAGAAGTGG-3’; TLE1-q-MSP-U reverse: 5’-</p><p>CACTAATATAACCCTAAAAACCAAA-3’.</p><p>*: DAPK1 q-MSP-U and TET2-q-MSP-M primer sequences were reported previously in </p><p>Calmon et. al.Cancer Genetics and cytogenetics 2007 (PMID: 17284367) and in Abdel-Vahab et. al. Blood 2009 (PMID: 19420352), respectively.</p><p>5 q-RT-PCR</p><p> q-RT-PCR was performed as described previously (8). Briefly, RNA was isolated using the </p><p>RNeasy kit (Qiagen Inc., Gaithersburg, MD). cDNA was generated with Superscript II (Life </p><p>Technologies, Grand Island, NY) using a combination of oligo-dT and random hexamer primers.</p><p>The q-RT-PCR primers were designed across exon-exon junctions. q-PCR was performed using </p><p>DyNAmo HS SYBR Green qPCR Kit (Thermo Scientific Inc) with DNA Engine Opticon 2 or </p><p>CFX Connect (Bio-Rad, Hercules, CA) real time thermocyclers. Melting curves were analyzed to ensure amplification specificity. The housekeeping gene RPL13A was used to calibrate gene expression. q-RT-PCR primers used in this study are as follows: BIM forward: 5’-</p><p>GTGTGCACCACATGAGCA-3’; BIM reverse: 5’-GCCAACAGCCTGGTCTTTAATA-3’; </p><p>TET2 forward: 5’- TTCCTCCCTGGAGAACAGCTCAAA-3’; TET2 reverse: 5’- </p><p>TGCTGCATGACTGGTCCTGAAAGT-3’; TLE1 forward: 5’- </p><p>AGATAACCTCCTCAATGCTTGG-3’; TLE1 reverse: 5’-</p><p>ATCATCCACAGAGATGTCACAG-3’; IL12Rβ2 forward: 5’- </p><p>ACCAAGCATTTGCATTGCTATC-3’; IL12Rβ2 reverse: 5’-TCTATCAGGAGCCTGTCCAA-</p><p>3’; ASNS forward: 5’-AGAAGCCTTCAGTGATGGAATAA-3’; ASNS reverse: 5’-</p><p>GGAAATTTCTGGGCTGCATTT-3’; DAPK1 forward: 5’-TGTCGCTGCAGCAGTTT-3’; </p><p>DAPK1 reverse: 5’-CGTCCTGAACTGTTTCACTTTG-3’; SOCS6 forward: 5’-</p><p>GCCAGATGTGGAAGGACATAC-3’; SOCS6 reverse: 5’-GCAGCCGAGACCTTGAATAA-</p><p>3’; ZFHX3 forward: 5’-CCCTTAACCTTCCTACAGCATATC-3’; ZFHX3 reverse: 5’-</p><p>ACCCATCAAGTTCGGCTTAG-3’; CD300A forward: 5’-AATCTGGAGCTGCTGATGTG-</p><p>3’; CD300A reverse: 5’-CACCGAGGCATAGTGAAGTT-3’; GAPDH forward: 5’-</p><p>AGGTGGTCTCCTCTGACTTCAACA-3’; GAPDH reverse: 5’-</p><p>6 CCCTGTTGCTGTAGCCAAATTCGT-3’; RPL13A forward: 5’-</p><p>ACCGTCTCAAGGTGTTTGACG-3’; RPL13A reverse: 5’-GTACTTCCAGCCAACCTCGTG-</p><p>3’.</p><p>Western Blotting </p><p>Western blotting was performed as described previously (2, 8). Briefly, whole cell extracts from equal numbers of cells from normal NK cells and NK cell lines (n=6) were resolved on a </p><p>10% SDS-PAGE gel and transferred to a PVDF membrane. ECL Plus kit (GE Healthcare Bio-</p><p>Science, NJ, USA) was used to visualize the expression. BIM (Cell Signaling Technology, </p><p>Danvers, MA) antibody was used to detect BIM expression, and α-tubulin (Sigma Aldrich, St </p><p>Louis, MO) was used as the loading control.</p><p>Detection of phospho-STAT3 expression was performed as described previously (2) with the following modifications. RIPA buffer supplemented with a protease inhibitor cocktail (Sigma</p><p>Aldrich, St Louis, MO) and phosphatase inhibitor cocktails 2 and 3 (Sigma Aldrich, St Louis, </p><p>MO) were used to prepare the whole cell lysate. 20 ug protein/sample was used for Western blot.</p><p>BSA (Sigma-Aldrich, St Louis, MO) was used instead of non-fat dry milk for blocking. Primary antibodies against phospho-STAT3 (Cell Signaling, Danvers, MA) and α-Tubulin (Sigma-</p><p>Aldrich) were used.</p><p>Expression of BIM-EL, SOCS6 and ASNS in NK cell lines The BIM-EL (extra-long alternatively spliced form), SOCS6 or ASNS coding sequence </p><p>(NCBI accession numbers: NM_138621.4 NM_004232.3 and NM_133436, respectively) was </p><p>PCR-amplified with the high-fidelity PfuUltra II Fusion HS DNA Polymerase (Agilent </p><p>Technologies, Palo Alto, CA) from 48h IL2-activated human NK cell cDNA and cloned into the pMIG expression plasmid upstream of the internal ribosomal entry site (IRES) using NotI and </p><p>7 SalI sites. The constructs were validated by diagnostic restriction mapping and Sanger sequencing of the inserts. </p><p>Retroviral transduction of NK-cell lines was performed as previously described (1) with the following modifications: 4 µg of pMIG, pMIG-BIM-EL, pMIG-SOCS6 or pMIG-ASNS was cotransfected with 4 µg of the packaging construct PCL-Ampho into the 293T cell line. </p><p>Transduction was performed once rather than twice. KAI3 cells were cultured in the presence of </p><p>20% FBS to increase transduction efficiency. Transduction efficiency was determined with flow cytometry by quantifying the percentage of GFP+ cells 2 days post-transduction.</p><p>Apoptosis assay</p><p>Apoptosis of retrovirally transduced cells was evaluated on GFP+ gated cells by flow cytometry after staining the cells with annexin V-PE (Apoptosis Detection Kit; BD Biosciences) according to the manufacturer’s instructions. </p><p>Transfection of 293T cells with BIM-EL for western blot</p><p>70-80% confluent 293T cells seeded in 10cm plates were transfected with 4µg pMIG or 4µg pMIG-BIM-EL vector using Turbofect in vitro transfection reagent (Thermoscientific, Waltham, </p><p>MA) according to the manufacturer’s recommendations. Cells were harvested 48h after transfection. </p><p>Additional statistic methods</p><p>Perl scripts that had a random number generator and that picked each sequence only once (9) were used to randomly select 16,000,000 sequences from the total number of sequences aligned to the genome. For sites with both forward and backward mappable reads, the cut counts were summed. To compensate, cut counts were doubled at sites with mappable reads only in one direction (23.9% of sites; 12.3% of sites at promoters). HpaII cut sites in the data set were </p><p>8 reannotated to show their relationship to the nearest TSS of all RefSeq genes, excluding processed RNAs, such as miRNAs (host genes were retained). Their presence in a gene body or in a CGI (using the UCSC web browser annotation at genome.ucsc.edu) was also noted as was the chromatin structure encompassing the site in hESCs, especially noting the “poised” chromatin structure. Each site was assigned uniquely to the closest TSS. Sites within 1 kb of the </p><p>TSS for each gene (or within 1 kb of any TSS for genes with more than one promoter) were considered to be in the promoter. A promoter was considered CGI-associated if at least one promoter site was in a CGI. Similarly, a promoter was considered “poised” if at least one promoter site was assigned the poised state. </p><p>Compared to normal samples, most NK tumors and both NK cell lines showed an overall decrease in cut counts at promoter regions compared to gene bodies and extragenic regions. To distinguish the relative degree of promoter hypermethylation compared to hypomethylation elsewhere, cut counts were renormalized based on the assumption that a subset of HpaII sites are likely never to be methylated in any of the samples. A set of highly expressed genes were derived based on the sample value among normal and malignant NK samples with the lowest expression. The top 20% of genes was determined and of these, the bottom half in SD gene expression was taken. Genes poised in hESCs or not associated with a CGI were removed, leaving 1083 genes. All cuts within 500 bp of the TSS for these genes were then summed after removing sites overlapping a common (≥1% frequency) single-nucleotide polymorphism (SNP) </p><p>(dbSNP138, UCSC browser) or with >100 cuts in a normal NK sample. Cut counts were then normalized to the mean of the summed counts of the 3 normal NK replicates. With one exception</p><p>(case #10, 1.77), normalization factors were close to 1 (range: 0.91 to 1.41) and were not sensitive to minor changes in the criteria for generating the gene list for normalization. After </p><p>9 normalization, outlying cut counts >101 were truncated to 101. For each gene region, the average cuts per site were determined, after excluding common SNPs. Any averages <0.5 were raised to </p><p>0.5, and average cuts were then log2 transformed. </p><p>For each gene promoter, the S.D. (MSCC.SD) was calculated from two cell lines (KHYG1 and NK92) and nine tumor samples (cases #3, 9, and 12 were excluded). The S.D. for gene expression (GEP.SD) was calculated using the seven cases and three cell lines (KHYG1, NK92, and NKYS) with GEP data along with the four normal NK samples. The correlation coefficient between MSCC cuts and GEP (MSCC.GEP) was evaluated using the seven cases and two cell lines (KHYG1 and NK92) with both types of data along with the mean value for normal NK cells. The number of malignant samples (of seven cases and three cell lines) with decreased gene expression (n.GEP.down) less than the minimum of the four normal NK samples was calculated </p><p>(n.GEP.down). </p><p>For genes with ≥5 promoter sites with nonzero cuts in the normal NK cells, MSCC was considered decreased if a one-sided paired Wilcoxon test was significant at p≤0.05 comparing the cut-counts of the malignant sample to the mean normal NK value. For the remaining genes, </p><p>MSCC was considered decreased if the MSCC was less than normal NK by at least 1.15 log2 units (a value set so that one quarter of all genes with GEP data would be decreased in at least three malignant samples). The number of malignant samples with decreased MSCC </p><p>(n.MSCC.down) was calculated. </p><p>Genes that are hypermethylated in NK tumor cells, are never expressed significantly in NK cells, but are expressed in the nontumor component of a sample may show a strong correlation between cut-counts and expression only because both MSCC cuts and expression derive from a varying proportion of nontumor cells. To try to remove genes expressed in nontumor cells but </p><p>10 not in NK cells, we set an expression filter requiring either that the maximum normal NK expression fall into the upper half among genes or that the median expression in tumor samples be less than the maximum expression among normal NK cells or the three cell lines with GEP data. </p><p>Of the two filters for variability in MSCC and expression, MSCC-SD requires that MSCC.SD be in the upper half among genes, and GEP-SD requires that GEP.SD be in the top quarter among genes. MSCC-down and GEP-down require that n.MSCC.down and n.GEP.down be in three or more malignant samples (out of 11 and 10, respectively). </p><p>We considered a wide range of methods to filter for genes in NK tumors that are silenced by abnormal promoter methylation. Most of the same genes of interest appear on the resulting list even if the filters are somewhat altered; however, more “interesting genes” are captured by using a large number of weak filters than by using a smaller number of more stringent filters. As shown in Fig. 2, the filters successively increased the average RMSCC.GEP, suggesting that they were successfully removing “noise”. </p><p>To identify additional interesting genes that share statistical similarities to those on the final lists, we generated a linear predictor based on the parameters described above. First, we performed ANOVA comparing the correlation coefficient MSCC.GEP with GEP.SD, </p><p>MSCC.SD, n.MSCC.down and n.GEP.down (with the expression filter). The variance explained by m.MSCC.down was increased by a log2 transformation (log2(n-1), with values of 0 and 1 transformed to -8 and -2). </p><p>Response: R MSCC,GEP [f] (f = filtered) Df Sum Sq Mean Sq F value Pr(>F) gep.sd[f] 1 13.11 13.105 111.98 5.0E-26 *** mscc.sd[f] 1 15.78 15.779 134.82 5.7E-31 *** n.GEP.down[f] 1 12.02 12.024 102.74 5.0E-24 *** log.n.MSCC.down[f] 1 26.25 26.245 224.25 3.8E-50 *** Residuals 9906 1159.35 0.117 </p><p>11 Because the 22 genes in the 174-gene list that are most clearly TSGs can be considered the most likely genes to be silenced by hypermethylation, we generated a linear predictor by sequentially linearly combining GEP.SD with the remaining four parameters, minimizing the mean rank of the 22 TSGs are each step. The final predictor was 0.628145*gep.mscc+0.063495* log.n.MSCC.down + 0.047607*mscc.sd+ 0.24826*gep.sd+ 0.012496*n.GEP.down. This yielded a mean rank for the 22 TSG of 123.1 in the 9971 filtered genes. This ranking was used to determine which “near miss” genes to include in Table S3.</p><p>Supplementary Results</p><p>Low-expression genes are more hypermethylated than high-expression genes</p><p>Dividing the 7079 genes with GEP data and ≥5 evaluable promoter MSCC sites into expressed genes (top 75% normal NK expression) and low-expression genes (bottom 25%) and comparing tumor samples to normal NK cells, the low-expression genes were on average more hypermethylated than expressed genes, with an average decrease in median log2 MSCC counts of 0.42, versus 0.21 for expressed genes (p= 1.6x10-86, Wilcoxon test). They were also significantly decreased (p≤0.05 one-sided paired Wilcoxon test) in more malignant samples: in </p><p>3.17 malignant samples (of 11), versus 1.58 for normally expressed genes (p=5.2x10-119, </p><p>Wilcoxon).</p><p>Known tumor suppressor genes are enriched among genes silenced by promoter methylation.</p><p>We compared our lists of recurrently methylated and silenced genes to a curated list of 716 </p><p>TSGs (10). Of the 468 of these for which we have expression data, 12 (DAPK1, DDX3X, DOK2, </p><p>HPGD, IRF8, NAPEPLD, PTCH1, PTPN6, PYCARD, TET2, TP53INP1, and ZFHX3) were in </p><p>12 our list of 95 genes, representing a 3.3-fold enrichment (p=7.3x 10-4 significance, Fisher exact test). An additional 10 (CTBP2, GAS1, HIC1, ITGAV, LXN, MAF, PAWR, PLK2, RASSF8, and </p><p>ZBTB16) were in our 174-gene list, a 3.4-fold enrichment (p=5.2x 10-6 significance, Fisher exact test). </p><p>References 1. Kucuk C, Hu X, Iqbal J, Gaulard P, Klinkebiel D, Cornish A, et al. HACE1 is a tumor suppressor gene candidate in natural killer cell neoplasms. The American journal of pathology. 2013;182:49-55. 2. Iqbal J, Kucuk C, Deleeuw RJ, Srivastava G, Tam W, Geng H, et al. Genomic analyses reveal global functional alterations that promote tumor growth and novel tumor suppressor genes in natural killer-cell malignancies. Leukemia. 2009;23:1139-51. 3. Iqbal J, Weisenburger DD, Chowdhury A, Tsai MY, Srivastava G, Greiner TC, et al. Natural killer cell lymphoma shares strikingly similar molecular features with a group of non- hepatosplenic gammadelta T-cell lymphoma and is highly sensitive to a novel aurora kinase A inhibitor in vitro. Leukemia. 2011;25:348-58. 4. Gu H, Smith ZD, Bock C, Boyle P, Gnirke A, Meissner A. Preparation of reduced representation bisulfite sequencing libraries for genome-scale DNA methylation profiling. Nature protocols. 2011;6:468-81. 5. Krueger F, Andrews SR. Bismark: a flexible aligner and methylation caller for Bisulfite- Seq applications. Bioinformatics. 2011;27:1571-2. 6. Hebestreit K, Dugas M, Klein HU. Detection of significantly differentially methylated regions in targeted bisulfite sequencing data. Bioinformatics. 2013;29:1647-53. 7. Li LC, Dahiya R. MethPrimer: designing primers for methylation PCRs. Bioinformatics. 2002;18:1427-31. 8. Kucuk C, Iqbal J, Hu X, Gaulard P, De Leval L, Srivastava G, et al. PRDM1 is a tumor suppressor gene in natural killer cell malignancies. Proc Natl Acad Sci U S A. 2011;108:20119- 24. 9. Hlady RA, Novakova S, Opavska J, Klinkebiel D, Peters SL, Bies J, et al. Loss of Dnmt3b function upregulates the tumor modifier Ment and accelerates mouse lymphomagenesis. J Clin Invest. 2012;122:163-77. 10. Zhao M, Sun J, Zhao Z. TSGene: a web resource for tumor suppressor genes. Nucleic Acids Res. 2013;41:D970-6.</p><p>13</p>
Details
-
File Typepdf
-
Upload Time-
-
Content LanguagesEnglish
-
Upload UserAnonymous/Not logged-in
-
File Pages13 Page
-
File Size-