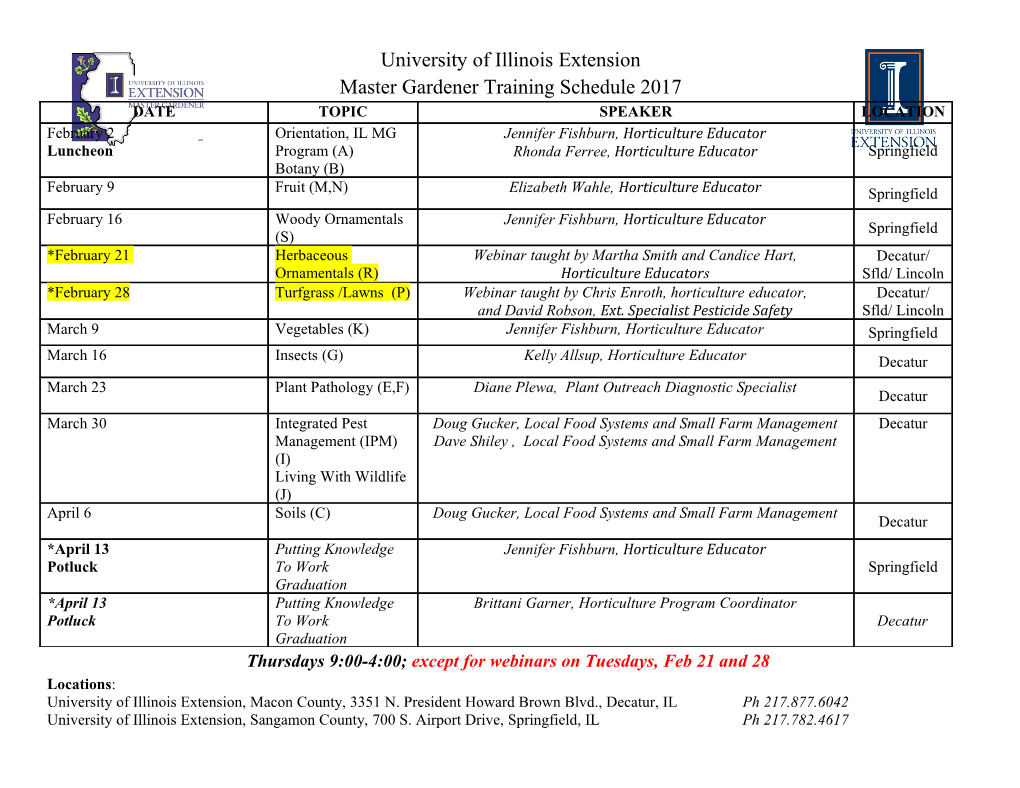
<p> Review</p><p>Alternatives to Cyanide in the Gold Mining Industry: What Prospects for the Future?</p><p>Gavin HilsonA and A.J MonhemiusB*</p><p>A School of City and Regional Planning, Cardiff University, Glamorgan Building, King Edward VII Avenue, Cardiff CF10 3WA, Wales, UK</p><p>B Department of Earth Science and Engineering, Royal School of Mines, Imperial College, London, SW7 2BP</p><p>______</p><p>Abstract</p><p>This paper reviews a series of alternative lixiviant systems for the recovery of gold from ores and concentrates. For over 100 years, cyanide has been the leach reagent of choice in gold mining because of its high gold recoveries, robustness and relatively low costs. The environmental damages resulting from its mismanagement, however, have initiated widespread research aimed at identifying and developing less toxic leaching agents. The most widely researched alternative lixiviants for gold ores are examined in this paper, but it is evident that none of them has yet made any significant inroad into the dominant position of cyanide as the reagent of choice at the vast majority of gold mines worldwide..</p><p>Keywords: cyanide; gold; alternatives; thiourea; thiosulphate; thiocyanate ______</p><p>Introduction</p><p>The debate surrounding the use of cyanide in the mining industry has fuelled considerable investigation into the development of more environmentally benign alternatives. For over 100 years, cyanide has featured prominently as a leach reagent at gold mines because of its high efficiency and relatively low cost. Although Hagen first discovered that gold was soluble in cyanide in 1806, it was not until the work of John Stewart MacArthur in the 1880s that the value of cyanide as a leach reagent for gold ore treatment was finally realized. A chemical metallurgist, MacArthur was granted British Patent No. 14174, Process of Obtaining Gold and Silver from Ores, in 1887. This technology, called “cyanidation”, received immediate industrial success in New Zealand in 1889 and South Africa in 1890. Gold worth US$14 million was recovered by cyanidation over the first five-year period of operation of the process [1], which eventually replaced chlorination processing outright. By 1907, global annual production of gold had doubled because of an increased use of cyanide; it continues to this day to be the primary leaching method in the gold mining industry [2]. </p><p>* Corresponding author Email: [email protected] 1 Today, over one million metric tonnes of cyanide – representing 80% of total production – are used annually by the chemical industry for the production of organic chemicals such as nitrile, nylon, and acrylic plastics. The remaining 20% is used to manufacture sodium cyanide, a solid form of cyanide, of which 90% (i.e. 18% of total production) is used in mining operations around the world for gold recovery purposes [3]. Typically, dilute solutions of sodium cyanide (NaCN), normally in the range of 0.01- 0.05% cyanide (100 to 500 parts per million), are used in tank leaching and heap leaching processes. Cyanide ions (CN-), are the active ingredients in the gold leaching process, which dissolve the gold contained in the ore by complexation (see below), forming a “pregnant solution” from which gold is later extracted. - - - 4Au + 8CN + O2 + 2H2O = 4Au(CN)2 + 4OH A series of recent environmental accidents at various gold mines around the world, however, has precipitated widespread concern over the use of cyanide as a leach reagent. In the majority of these cases, cyanide from processing operations has entered the environment either by leakage through tears and or punctures in protective heap leach liners, or by spillage from overflowing solution ponds or tailings storage areas, [4]. For example, in Guyana, some 2.9 million m3 of cyanide-laced tailings and wastewater was released by a collapse of the tailings dam at the Omai mine in Guyana on the night of August 19th 1995, polluting the Omai River. A disaster similar in scale occurred on January 30th 2000 in Baia Mare, Romania, where the tailings dam of Aurul S.A. was breached, resulting in the discharge of wastewater containing as much as 100 tonnes of cyanide that eventually reached the Danube river. (See Table 1 for additional examples of cyanide spills and resulting impacts). </p><p>Table 1: Examples of recent cyanide-related mine accidents and resulting environmental impacts [6]</p><p>Company/Mine Location and date Impacts Galactic Resources Ltd.’s Colorado, USA, Caused severe environmental problems along Summitville Mine, 1992 a 17-mile stretch of the Alamosa River Pegasus Corporation’s Zortman- Montana, USA, 1997 Severe contamination of groundwater; Landusky Mine substantial wildlife deaths Echo Bay’s McCoy/Cove Gold Nevada, USA, 1989 Eight cyanide leaks over a two year period Mine and 1990 released almost 900 lbs of cyanide into the environment Kumtor Mine Kyrgyzstan, 1998 Almost two tons of sodium cyanide was accidentally released into surface waters Cambior Mining Company’s Omai Guyana, 1995 Released more than 860 million gallons of Mine cyanide-laden tailings into a major river</p><p>Although cyanide is not regarded as a persistent toxin, it is nevertheless a deadly poison in high concentrations, posing a serious health threat to a wide range of ecological entities. In fact, the contention that cyanide is not persistent and does not give rise to chronic health or environmental problems [3] is somewhat misleading. As Moran [5] explains, the CN- ion, which is ubiquitous in nature, tends to react readily with innumerable chemical agents and molecules to form hundreds of different compounds, many of which are lethal to organisms. To determine the persistence and toxicity of the chemical, chemists have traditionally distinguished “weak” from “strong” cyanide complexes. Weak cyanide complexes, often referred to as “weak acid dissociable” or WAD cyanide, break down, releasing free cyanide ions, when the pH is lowered. WAD cyanideincludes cyanide complexes of cadmium, copper, nickel, silver, and zinc, all of which can disassociate in acid conditions and produce environmentally-significant quantities of toxic cyanide ions. Strong cyanide complexes are more stable than WAD cyanide, thus degrading much more</p><p>2 slowly under natural conditions. These include cyanide complexes containing gold, cobalt, and iron, which are more stable in solution [3, 7]. The toxicity of cyanide varies, with free cyanide being the most toxic, and cyanide complexes being less or non-toxic [8].</p><p>In excessive quantities, cyanide can be lethal to humans and to animal species because it binds to the iron-carrying enzymes required for cells to use oxygen, thus inhibiting bodily tissues from extracting oxygen from the blood (Table 2). The body then rapidly exhibits symptoms of oxygen starvation and suffocation. Symptoms of cyanide poisoning include irregular heartbeat, convulsions, chest pains and vomiting [7]. Many species of migratory birds can come to grief at gold mine heap leaching facilities, some dying immediately after consuming cyanide-containing water. Signs of acute poisoning to livestock, including initial muscle tremors, salivation, lacrimation, defecation, urination and laboured breathing, usually occur within 10 minutes; and death can follow quickly [4, 9-11]. In the aquatic environment, young, freshwater fish such as salmonoids are particularly susceptible to cyanide poisoning.</p><p>It is against the background of these environmental impacts that indigenous groups and representatives of the NGO community in particular have lobbied for the mining industry to develop alternative, less toxic, leach reagents. The purpose of this article is to review the most widely-researched alternative leach reagents to cyanide. Several substitutes have been proposed (Table 3) but generally, the non-cyanide lixiviants, thiourea, thiocyanate and thiosulphate are regarded as being the most realistic substitutes. These, along with a number of other possible alternatives, including halides and the recently-developed coal-oil agglomeration process, are reviewed individually in the discussion that follows.</p><p>Table 2: Single dose toxicity of sodium cyanide (in mg NaCN kg-1 body weight), selected species [13]</p><p>Species Oral LD50 (95% confidence limits) Mallard 2.7 Human 3.0 American kestrel 4.0 Coyote 4.1 Black vulture 4.8 Lab rat 5.1 Little brown bat 8.4 Eastern screech owl 8.6 Japanese quail 9.4 Domestic chicken 21 White footed mouse 28</p><p>3 Table 3: Suggested alternatives to cyanide [14]</p><p>Reagent type Concentration pH Basic Research Extent of range range chemistry level commercialisation Ammonia High 8-10 Simple Low Pilot tests, +100 degrees centigrade Ammonia/cyanide Low 9-11 Simple Extensive Applied to Cu/Au ores Ammonium High 8.5-9.5 Complex Extensive Semi commercial thiosulphate Slurry CN-electrolysis Low 9-11 Simple Historical Limited historical Sodium sulphide High 8-10 Simple Low Geological interest only Alpha-hydroxynitriles Moderate 7-8 Fairly simple Fairly None popular Malononitrile Moderate 8-9 Fairly Low None complex Alkali cyanoform Poorly defined 9? Poorly Low None defined Calcium cyanide Poorly defined 9? Poorly Low none defined</p><p>CSTU(1) High 9-10 Complex Very low None. KMnO4 is oxidant Alkaline polysulphides High 8-9 Poorly Low None defined Biocatalyzed Poorly defined 8-10 Poorly Low None biosulphate defined Hypochlorite/chloride High chloride 6-6.5 Well defined Extensive Historical and modern Bromocyanide High 6-7 Poorly Historical Historical defined Iodine High 3-10 Poorly Low None defined Bisulphate/sulphur High 4-5 Fairly simple Low None dioxide Bacteria High 7-10 Fairly Low, None complex growing Natural organic acids High 5-6 Fairly Low None complex DMSO, DMF Poorly defined 7 Poorly Very low None defined Bromine/bromide High 1-3 Well defined Low Historical Thiourea High 1-2 Well defined Fairly Some concentrates popular Thiocyanate Low 1-3 Well defined Low None Aqua regia High Below Well defined Low Analytical and refining 1 Acid ferric chloride High Below Well defined Low Electrolytic Cu slimes 1 Ethylene thiourea High 1-2 Poorly Very low None defined Haber process Poorly defined Proprietary One entity None “Bio-D leachant” Poorly defined Proprietary One entity None High High 6-7 Simple historical Historical temp chlorination </p><p>The Challenges to Developing Alternatives for Cyanide</p><p>The main challenge to developing substitutes for cyanide rests in ensuring that the particular metallurgical processes selected for the extraction of gold matches the characteristics of the ore. Alternative lixiviants or leaching agents should also be [12]: 1) inexpensive and recyclable 2) selective; 3) non-toxic; and</p><p>4 4) compatible with downstream recovery processes.</p><p>Box 1 highlights additional criteria which must be considered for gold lixiviants.</p><p>For over a century, sodium cyanide has been the predominant leach reagent for gold because of its excellent performance with a great variety of ores and its relatively low cost [15]. Moreover, despite being a potentially deadly poison if ingested in high doses, cyanide does not give rise to chronic health or environmental problems if present in low concentrations [3]. It is ubiquitous in nature, produced by a wide range of micro- organisms and over 2500 plant species as part of their normal metabolisms [7]. The low concentrations of cyanide used in gold milling and heap leaching are quickly degradable: cyanide is nowhere near as harmful as other naturally occurring toxins (e.g. tetanus, strychnine) because it is readily transformed by natural physical, chemical, and biological processes, into less or non-toxic forms.</p><p>The primary challenge in devising a suitable substitute for cyanide in gold processing, therefore, lies in developing an equally effective and degradeable leach reagent, which is not a persistent environmental toxin. As gold cyanidation rates are relatively slow, the industry has been searching for faster gold leaching reactions capable of facilitating high metal recovery rates. Although there has been significant research undertaken to develop plausible substitutes, driven mainly by economics, the list of effective options is limited, as most are addressed to refractory ores and are not amenable to simple cyanide leaching [15]. The discussion that follows reviews the most promising alternatives. </p><p>Box 1: Criteria which should be considered for gold lixiviants [16]</p><p>Economic Considerations Capital outlay Extraction costs Availability Detoxification/recycling costs</p><p>Toxicity Emissions Handling Environmental toxicity</p><p>Process applicability Limitations (e.g. ore type, selectivity, control, separation, etc.) Recyclability Detoxifiability Feasibility at a large scale</p><p>Thiourea Leaching of Gold</p><p>Overview</p><p>The use of thiourea (NH2CSNH2) as a gold extraction agent has shown considerable promise. In acidic conditions, thioureadissolves gold, forming a cationic complex;the</p><p>5 reaction is rapid and gold extractions of up to 99% can be achieved [15]. The anodic reaction follows the equation:</p><p>+ - Au + 2 CS(NH2)2 = Au(CS[NH2]2)2 + e</p><p>The reagent, however, has to be used under relatively restricted conditions, as it is fairly stable thermodynamically in acid and neutral mediums, but decomposes rapidly in basic solutions [17]. Leaching is normally carried out in the pH range of 1-2, and its successful application is contingent upon careful optimisation and control of pH, redox potential, thiourea concentration, and leaching time. The critical parameters for thiourea leaching are as follows [18]: pH: 1.4 adjusted accordingly with H2SO4 Redox potential Max 250 mv Min 150 mv Thiourea Conc. 1% Thiourea Consump. 2 kg/t Leach Time 10-15 minutes</p><p>It is claimed in a number of sources [15, 19-20] that the work of the Soviet scientists Plastin and Kozukhova [21-22] was the pioneering research in this field, but the potential of thiourea for gold dissolution was recognized much earlier by Moir [17] who, in 1906, published a paper entitled “Thiocarbamide - a new solvent for gold” [23]. Moir reported the results of experiments in which he dissolved pieces of gold leaf in thiourea (then called thiocarbamide) solutions. He showed that acid conditions were necessary and that the reaction was rapid in the presence of suitable oxidants. At that time, however, cyanide was already established as the reagent of choice for gold ore treatment and so Moir’s discovery lay neglected for over half a century. Thiourea has only received serious consideration as an alternative to cyanide in the past 20 years [12]. It is an attractive option for treating refractory ores and floatation concentrates, although it is hoped that with further research, it can be adopted to treat lower grade ores [18].</p><p>The Thiourea Process and Potential Benefits</p><p>In a comprehensive review, Pyper and Hendrix [24] identified the following about thiourea kinetics: The use of ferric ion in sulphuric acid is the most effective system; The rate of leaching is contingent upon thiourea and oxidant concentrations; The ferric ion ties up thiourea in iron-thiourea complexes; and The rate of gold dissolution is strongly determined by pH.</p><p>Generally, gold can be recovered from thiourea via cementation (using iron powder, or aluminium), or by absorption on activated carbon, or by electrowinning, or by the use of ion-exchange resins. Compared to cyanide, thiourea has certain advantages, including low sensitivity to base metals (Pb, Cu, Zn, As); low sensitivity to residual sulphur in calcines; high gold recovery from pyrite and chalcopyrite concentrates; and satisfactory recovery of gold from carbonaceous (refractory) ores [15]. It also poses less of a threat environmentally [25-26]. On its own, thiourea has a lower toxicity and higher rate of gold and silver dissolution than cyanide [27]. Moreover, if using cyanide to treat refractory sulphide gold-containing ore, which have been pre-oxidised to release the gold, the highly</p><p>6 acidic solutions produced must be neutralized prior to cyanidation, whereas thiourea can be used directly, since gold leaching occurs in acidic solutions [28].</p><p>Despite the proven effectiveness of thiourea as a gold leach reagent [27,29-30], there are still few full-scale operations in existence. Its commercial adoption has been hindered by the following three factors [31]: (1) It is more expensive than cyanide; (2) Its consumption in gold processing is high; and (3) The gold recovery step requires more development.</p><p>Reagent consumption is exceedingly high because thiourea is readily oxidized in solution, and as Prasad [18] implies, the high costs attached to leaching are likely due to the thiourea process still being in an infancy stage. Past attempts to adopt this reagent has led to over- consumption and increased production costs. There is also an emerging concern about its environmental impact. Despite claims that thiourea is less toxic than cyanide, it is a suspected carcinogen, and thus must be treated with caution [12].</p><p>Experimental Work and Commercial Developments</p><p>The effectiveness of thiourea as a substitute for cyanide has been verified through substantial experimental work. As Monhemius [17] reports, in terms of its feasibility as a leach reagent, Shultz originally argued in his work that the addition of sulphur dioxide during thiourea leaching prevents it from degrading chemically, thus resulting in lower consumptions of the reagent[32-33]. The author proposed that the bubbling of sulphur dioxide during the thiourea leaching of precious metals prevents decomposition of formamidine disulphide , which can lead to thecoating of gold surfaces by fine adhesive sulphur, a decomposition product.</p><p>More recently, Abbruzzese et al. [34] carried out further laboratory evaluations to assess the feasibility of thiourea leaching. Gold was recovered from leaching solutions by adsorption onto activated carbon; gold was eluted from the carbon using water/alcohol mixtures, and the final recovery of gold from the elution solutions was accomplished via electrowinning. The authors reported an overall gold yield of 82% with a low consumption of reagents (based upon a gold extraction of 85% at room temperature, and a 99% recovery after the adsorption, desorption and electrowinning phases). More specific studies have been carried out such as that of Gonen et al. [35], who examined the effects of thiourea and oxidant type, pH, agitation time, and particle size on gold recovery from Gumushane- Mastra ore in Turkey. It was determined that with short leaching times and low pH values, the amount of oxidantand the consumption of thiourea decreased. Gold extractions of 75% were achieved using a particle size of 150-mesh in a mixing time of five hours.</p><p>Miroslav et al. [36] examined the feasibility of thiourea extraction of gold from copper slimes in Kovohutny Krompachy, Slovenia. The authors reported that thiourea extracted some 80% of gold from the residues. Orgui and Atalay [37] reported similar results. In an attempt to extract gold from Kaymaz gold ore (4gAu/t) using thiourea and iron (III) sulphate as an oxidizing agent, at room temperature, it was possible to obtain a gold extraction of 80% in six hours. The process has also proven to be a successful non-toxic solvent for gold in Russia [38].</p><p>Thiourea leaches gold more rapidly than cyanide, and is less toxic; forms cationic complexes; and can be utilized on ores refractory to cyanideand in heap and in situ</p><p>7 leaching [39]. However, as previously explained, research gaps and costs have hindered its wide-scale adoption as a leach reagent in the gold mining industry. Costs can be exorbitant because of the quantity of reagent required. As Presad et al. [18] explain, the high consumption of thiourea and low rate of dissolution is attributable to an inhibiting coating of sulphur on the surface of the gold particles formed because of thiourea degradation. It has, however, been proposed that adding sulphur dioxide to leaching solutions would control the redox couple between the thiourea and its first decomposition product, formamidine sulphide, thereby keeping the formation of sulphur to an absolute minimum.</p><p>The most significant commercial development involving thiourea made to date in the Western world has been at the New England Antimony Mines in New South Wales, Australia, which was commissioned in 1982. As la Brooy [31] explains, because of thiourea’s tolerance of stibnite, the company was able to recover 50-80% of the gold from a stibnite concentrate using a thiourea leach, followed by gold recovery onto activated carbon. More recently, Lacoste-Bouchet et al. [40] used thiourea to leach gold from ore containing 0.4% Cu and 5.6g/t Au obtained from the Val d’Or Mine in Quebec. Gold extractions of 89% were obtained using thiourea at a rate of 3.9 g/t.</p><p>Thiocyanate</p><p>In 1906, White [41] first demonstrated that thiocyanate dissolved gold effectively in the presence of a suitable oxidizing agent; however work with this reagent remained stagnant until Fleming re-opened the field in 1986 [42]. As Barbosa and Monhemius explain [43], the leaching of gold with thiocyanate, if performed between pH 1 and 2, enables the use of iron (III) as an oxidizing agent. During the dissolution of gold by iron (III)-thiocyanate, FeIII is reduced to FeII while oxidizing SCN-, producing several intermediate species, - including (SCN)3 and (SCN)2, which can both oxidise and complex gold. In aqueous solutions, acidified thiocyanate can dissolve gold to form both Au(I) and Au(II) complexes [44].</p><p>In terms of relevant research, Barbosa-Filho and Monhemius [45] describe aspects of the chemistry and thermodynamics of thiocyanate, and Broadhurst and du Perez [46] describe the speciation of the gold-thiocyanate system. In subsequent experimental work undertaken by Barbosa-Filho and Monhemius [47-8] withsolutions of FeIII-thiocyanate, which reduced from FeIII to FeII with the oxidation of SCN-, it was shown that the auto- - reduction reaction produced both (SNC)3 and (SNC)2, which were fundamental in the mechanism of the thiocyanate dissolution of gold. The authors concluded that thiocyanate is an “effective lixiviant for gold in acidic conditions, yielding dissolution rates that are comparable to those obtained with thiourea while offering the advantage of much greater stability against oxidative decomposition”. Kholmogorov et al. [49] recovered over 95% of gold from weak acidic solutions, recovering thiocyanate complexes on carbon adsorbents and ion exchangers [49]. Further research was performed at a laboratory scale by Monhemius and Ball, who used iodide-catalyzed thiocyanate solutions to leach gold ores derived from the Dominican Republic [50]. These researchers concluded that the thiocyanate system used gave results comparable to that of a cyanide system, and exhibited considerably greater effectiveness than thiourea. </p><p>Thiosulphate Leaching</p><p>2- Thiosulphate (S2O3 ), a chemical used widely in photography and in the pharmaceutical industries, has also been proposed as a substitute for cyanide. As Yannopoulis [23]</p><p>8 explains, gold dissolves slowly in alkaline thiosulphate. The rate of dissolution is influenced by the concentrations of thiosulphate and dissolved oxygen, the process temperature, and can be enhanced by adding copper ions [44]. Gold forms a stable anionic complex with thiosulphate according to the following reaction:</p><p>2- 3- - 2Au + 0.5 O2 + 4S2O3 + H2O 2Au (S2O3) 2 + 2OH</p><p>Although the gold thiosulphate complex is fairly stable once it has formed, alkaline conditions are necessary to prevent thiosulphate decomposition by acid [31]. As Aylmore [51] explains, the thiosulphate leaching process is catalysed by copper and has several advantages over the conventional cyanidation process, in spite of its slow rate. Acceptable gold leaching rates using thiosulphate are achieved in the presence of ammonia, together with copper [52]. In recent yearsthe reagent has been considered seriously as a potential substitute for cyanide because it generally causes fewer environmental impacts: thiosulphate leaching exhibits less interference from foreign cations and poses fewer pollution concerns [53-54].</p><p>The use of thiosulphate as a leach reagent in gold processing was first proposed during the early-1900s. As Aylmore and Muir [55] explain, in a procedure known as the “Von Patera Process”, gold and silver ores, first subjected to a chloridizing roast, were leached with thiosulphate. Before World War II, silver-rich sulphide ores in South America and the ore at Mexico’s LeColorado Mine were leached commercially with thiosulphate following a chloridizing roast [56-57]. Improvements were made to the procedure during the 1980s, most notably through the addition of copper ions and stabilization of thiosulphate by addition of SO2 or bisulphite [18].</p><p>Several promising experimental investigations [58-60] have since been undertaken. Most of this work has been carried out on complex ores containing copper, carbonaceous ores, or ores containing high concentrations of lead, zinc or manganese. For example, Yen et al. [61] used a mildly refractory gold ore containing pyrite and chalcopyrite to determine the feasibility of thiosulphate as an alternative heap leaching technology. The authors report that a gold extraction of 72% was achieved over a period of 50 days. The US Bureau of Mines used statistical experimental methods to determine thiosulphate leaching feasibility. Gold extractions of up to 62% were achieved from the leaching of low-grade carbonaceous ores [62].</p><p>A number of the more recent research efforts have targeted the development of pre- treatment strategies in order to improve recovery with thiosulphate. Balaz et al. [63] investigated the use of a mechanically-activated flotation concentrate from a complex copper-lead-zinc ore from Banska Hodrusa, Slovakia. Mechanical activation of the concentrate was achieved by ultra-fine grinding and proved to have a beneficial influence on the rate of extraction and recovery of gold using ammonium thiosulphate in the presence of copper ions. The authors report that 90% gold recovery was achieved in only a few minutes, compared to the 54% extraction achieved under equivalent conditions for untreated concentrate. Liu et al. [64] reported that sponge gold of 99.92% purity was produced by ammonium thiosulphate leaching, although it was found that the addition of copper sulphate not only accelerated the rate of gold leaching, but also increased thiosulphate consumption. Panayotov [65] reported similar results, indicating that a gold recovery of 91% can be achieved using thiosulphate, if the concentrate is pre-oxidised. In commercial practice, thiosulphate has been used successfully by Newmont and Consolidated Empire Gold Inc. in heap leaching of gold ore.</p><p>9 The principal problem with thiosulphate leaching is the high consumption of reagent during extraction [54]. Moreover, the process is generally slow, although acceptable leaching rates can be achieved in the presence of ammonia using copper (II) as an oxidant [51, 53]. Nevertheless, high reagent consumption renders most thiosulphate systems uneconomical overall, in spite of their potential environmental benefits. At present, no simple and affordable method for recovering gold from thiosulphate leach solutions exists [10]. </p><p>Coal-oil gold agglomeration</p><p>The coal-oil gold agglomeration (CGA) method has been identified as an alternative to cyanide for large-scale operations. More recently, it has also been promoted as an alternative to mercury amalgamation in the small-scale (artisanal) gold mining industry. Although the ability of oil to recover gold was known in the early-1900s, as Calvez et al. [66] explain, it was not until BP Australia patented the CGA process in 1986 that it began gaining recognition in the public domain as an alternative to cyanide in the gold mining industry. Batmen Engineering International Ltd. and Charlton Mineral Associates Pty Ltd. have also patented variations of the CGA method.</p><p>As described by Kotze and Petersen [67], the CGA process is based upon the recovery of hydrophobic/oleophilic free gold particles from ore slurries; gold grains ranging from one to 100 microns can be recovered with ease. Oil serves as a bridge between coal and gold particles, whilst coal acts as a carrier and facilitates effective separation of the gold-bearing agglomerates. The CGA method has proved to be effective if used to process liberated/free gold particles, such as those contained within alluvial deposits and some process tailings. </p><p>Considerable experimental work has been undertaken which suggests that CGA can be an effective gold ore processing alternative. For example, findings by Bellamy et al. [68], who tested ore samples collected from a New Zealand beach, show that the CGA method is a quicker, cleaner and more effective method than convention gold processing techniques, including cyanidation. Bonney [69] conducted laboratory analysis on mixtures of silica and gold powder and pilot tests on gold tailings; the laboratory results showing that under optimum conditions, gold recoveries of 95% could be achieved, whilst pilot test results gave recoveries of. 62-75%</p><p>Additional experimental work has been carried out to further validate the CGA method. For example, Marciano et al. [70] used diesel oil, kerosene and vegetable oil as agglomerating liquids to process gold-containing materials, achieving 90% gold recovery. Calvez et al. [66] performed tests using synthetic goldbearing samples to evaluate the underlying principles by which gold is recovered by coal-oil agglomerates, including oil- coal ratios, agglomerate-ore ratios, pH and coal particle size. The results show that gold recovery increases with decreasing coal particle size, an increased agglomerate-ore ratio, and a decreased oil-coal ratio. Moses and Petersen [71] also performed batch tests on synthetic (7gAu/t) ore, demonstrating that increased oil viscosity led to the formation of stronger agglomerates and hence, greater gold recovery. Kotze and Petersen [67] also achieved high gold recoveries (85%) in batch tests on artificial gold slurry mixtures. </p><p>Although promising experimental work has been carried out using the CGA method, it can only be used to process free gold particles. It therefore has limited potential at the large- scale, but as explained previously, it could prove to be a viable alternative to amalgamation in small-scale mining. 10 Halides</p><p>The use of halide (fluorine, chlorine, bromine, iodine and astatine) systems for gold dissolution pre-dates cyanidation [31]. With the exceptions of fluorine and astatine, all halogens have been tested and/or used for the extraction of gold [23]. Prior to the inception of cyanide, chlorine was extensively used as a leaching reagent for gold recovery from calcines, ores and concentrates. Chlorine can be generated in slurries and solutions via electrolysis of NaCl solution or by addition of MnO2 to hydrochloric acid [15]:</p><p>MnO2 + 4HCl MnCl 2 + 2H2O +Cl2</p><p>Gold is leached rapidly by chlorine at low pH:</p><p>2Au + 3Cl 2 2AuCl3</p><p>Chlorination rates are favoured by low pH, high chloride and chlorine levels, increased temperatures, and high ore surface areas [15, 31]. Although gold dissolution with chlorine is substantially faster than with cyanide, low concentrations of sulphides or other reactive - components in the ore can make reagent consumption excessive and can reduce AuCl4 back to metallic gold.</p><p>Several investigations have been undertaken to determine the effectiveness of chlorine [72- - 76]. Historically, AuCl4 was recovered from chloride leach solutions as metallic gold by natural reduction on carbon or charcoal, after which the “loaded” carbon was burnt to yield the gold. Today, the gold chloride complex is recovered on activated carbon as metallic gold, which generally leads to the loss of gold to tailings, due to attrition of fine gold particles from the surface of the activated carbon particles. Chloride gold extraction is more difficult to apply than cyanide extraction, however, for a number of reasons [4]: special stainless steel and rubber-lined equipment is required to withstand the highly corrosive acidic and oxidizing conditions; chlorine gas is highly poisonous and must be contained both to allow optimum utilization of the gas and to avoid any health risk; and although chlorination was used for pre-treating refractory and carbonaceous ores in several plants in the United States in the 1980s, few pilot or demonstration studies have since been reported on the use of these systems [78-79].</p><p>Bromine was first identified as a potential solvent for gold in 1846; its rate of leaching gold is enhanced in the presence of a protonic cation [15]. However, despite long being recognized as a powerful gold extractant, it is only recently that its application has been seriously considered. Bromine offers a number of advantages, including rapid extraction, non-toxicity, and adaptability to a wide range of pH values.</p><p>Although the high dissolution rates give it a definite advantage over cyanide, there is often high reagent consumption with bromine use [18], and because it can combine with other elemental species to form toxic compounds, the costs of construction materials to withstand the severe conditions and resultant health impacts can be exorbitant [4]. However, as Tran et al. [72] explain, the bromide-bromine system is receiving increasing attention, particularly since the Great Lakes Corporation patented its bromine-based gold leaching process, which is based upon a proprietary dibromo-dimethyl hydantoin compound. 11 Gold leaching rates in iodide solutions are also much faster than cyanidation. Despite the fact that gold iodide complexes are the most stable in aqueous solutions (among halogens), iodine continues to be under-utilised and under-researched as a gold lixiviant. This is largely because of its cost [15]. </p><p>Presently, halide systems have limited potential as substitutes for cyanide, which itself had replaced gold leaching agents such as chlorine in the first place. As La Brooy [31] explains, halide systems are currently limited to analytical applications, where the capacity for simultaneous sulphide destruction and speed of dissolution are more important than reagent consumption. Moreover, gold halide complexes are generally unstable and critics remain sceptical about their widespread use because of the significant chemical and process control required during processing to maintain gold in solution [72]</p><p>Conclusions</p><p>This paper has reviewed the most promising alternative gold leaching agents to cyanide. Although considerable research has been undertaken at a laboratory scale, most, if not all, of the alternatives identified have proven to have limitations that hinder their widespread adoption in the gold mining industry. Thiourea, for example, despite being a proven technology and suitable for refractory ores, is associated with high detoxification costs, has limited recyclability, and features process parameters that are difficult to control. Thiosulphate offers the same advantages, but is highly unstable and also has high detoxification costs and limited recyclability. Thiocyanate, whilst being more stable than the former two reagents, is under researched with considerable obstacles to be overcome before it could be considered as a viable alternative to cyanide. The CGA process, developed and patented by BP during the mid-1980s, is only suitable for processing free gold particles, and is therefore more likely to become a substitute for small-scale mercury amalgamation than cyanidation. Finally, halides, which have been utilized in this context in the past, are difficult to handle and control, and are also expensive. </p><p>It is concluded that, in spite of the vociferous opposition to the use of cyanide in the gold mining industry by sections of the environmental movement, unless further research and development makes one or more of the alterative lixiviants economically competitive, cyanide will continue to be the only practical leach reagent in large-scale gold extraction processes. It should be realised, however, that much of the opposition to the use of cyanide in the mining industry is based on a relatively small number of well-publicised pollution incidents, most of which were caused either by poor design or faulty operation of gold extraction processes. Cyanide has been used for over a century at gold mines all over the world and the evidence is overwhelming that in well-designed and properly managed mining operations, cyanide can be safely used as a gold leaching reagent without significant risk to human life or the environment.</p><p>Acknowledgements</p><p>12 References</p><p>[1] Young, C.A. 2001. Cyanide: Just the Facts, in Cyanide: Social, Industrial and Economic Aspects, pp. 99-114, Minerals and Materials Society, New Orleans. [2] Habashi, F. 1987. One hundred years of cyanidation. CIM Bulletin 80(905): 108-114. [3] Logsdon, M.J., Hagelstein, K., Mudder, T.I. 1999. The Management of Cyanide in Gold Extraction. ICME Publications, Ottawa. [4] Korte, G., Coulston, F. 1998. From Single-Substance Evaluation to Ecological Process Concept: The Dilemma of Processing Gold with Cyanide. Ecotoxicology and Environmental Safety 32: 96-101. [5] Moran, R. 2001. More Cyanide Uncertainties: Lessons From the Baia Mare, Romania, Spill – Water Quality and Politics. MPC Issue Paper #3, Mineral Policy Center, Washington. [6] Moran, R. 1998. Cyanide Uncertainties: Observations on the Chemistry, Toxicity, and Analysis of Cyanide in Mining-Related Waters. MPC Issue Paper No. 1, Mineral Policy Center, Washington. [7] EPA. 1995. Environmental Best Practice in Mining: Cyanide Management. Environmental Protection Agency, Australia. [8] Mosher, J.B., Figueroa, L. 1996. Biological Oxidation of Cyanide: A Viable Treatment Option for the Minerals Processing Industry? Minerals Engineering 9(5): 573-581. [9] Clayton, C.A., Goldberg, M.M., Potter, B.B. 1997. Design and analysis of an experiment for assessing cyanide in gold mining wastes. Chemometrics and Intelligent Laboratory Systems 36: 181-193. [10] Eisler, R., Clark Jr., D.R., Wiemeyer, S.N., Henny, C.J. 1999. Sodium cyanide Hazards to Fish and Other Wildlife from Gold Mining Operations, pp. 55-67, in The Environmental Impacts of Mining Activities (ed. J. Asuze), Springer Verlag, New York. [11] Haghighi-Podeh, M.R., Siyahati-Ardakani, G. 2000. Fate and toxic effects of cyanide on aerobic treatment systems. Water Science and Technology 42(3-4): 125-130. [12] Mine Action. 2000. Cyanide Alternatives: Alternatives for Cyanide in the Gold Mining Industry. Great Basin Mine Watch, Reno. [13] Miller, G.C., Pritsos, C.A. 2001. Unresolved Problems With the Use of Cyanide in Open Pit Precious Metals Mining in Cyanide: Social, Industrial and Economic Aspects, pp. 73-81, Minerals and Materials Society, New Orleans. [14] McNulty, T. 2001. Cyanide substitutes. Mining Magazine 184(5): 256-261. [15] Yannopoulos, J.C. 1991. The Extractive Metallurgy of Gold. Van Nostrand Reinhold, USA. [16] Gos, S., Rubo, The Relevance of Alternative Lixiviants with Regard to Technical Aspects, Work Safety and Environmental Safety, Paper prepared for CyPlus, Germany [17] Monhemius, A.J. 1987. Recent advances in the treatment of refractory gold ores. Paper presented at II Meeting of the Southern Hemisphere on Mineral Technology, Rio de Janeiro. [18] Presad, M.S., Mensah-Biney, R., Pizarro, R.S. 1991. Modern Trends in Gold Processing – an Overview. Minerals Engineering 4(12): 1257-1277. [19] Groenwald, T. 1976. The dissolution of gold in thiourea. Hydrometallurgy 1: 277- 290. [20] Groenwald, T. 1977. Potential applications of thiourea in the processing of gold. Journal of the South African Institute of Mining and Metallurgy 77: 217-223. [21] Plaskin, I.N., Kozhukhova, M. 1941. The solubility of gold and silver in thiourea. Dok. Ak. Nauk. SSR 31: 671-674. 13 [22] Plaskin, I.N., Kozhukhova, M. 1960. Dissolution of gold and silver in solutions of thiourea. Sb. Nauch. Tr. Ins. Tsvt. Met. 33: 107. [23] Moir, J. 1906.Thiocarbamide – a new solvent for gold. Journal of the Chem. Met. Mining Soc. S. Africa. May: 332-336. [24] Pyper, R.A., Hendrix, J.L. 1981. Extraction of gold from finely disseminated gold ores by use of acidic thiourea solution, pp. 57-75, in Extractive Metallurgy 81, Institution of Mining an Metallurgy, London. [25] Ubaldini, S., Fornari, P., Massidda, R., Abbruzzese, C. 1998. An innovative thiourea gold leaching process. Hydrometallurgy 48: 113-124. [26] Juarez, C.M., Dutra, A.J.B. 2000. Gold Electrowinning from Thiourea Solutions. Minerals Engineering 13(10-11): 1083-1096. [27] Lee, H.Y., Kim, S.G., Oh, J.K. 1997. Cementation behaviour of gold and silver onto Zn, Al, and Fe Powders from Acid Thiourea Solutions. Canadian Metallurgical Quarterly 36(3): 149-155. [28] Deng, T.L., Liao, M.X., Wang, M.H., Chen, Y.M., Belzile, N. 2001. Enhancement of gold extraction from bioxidation residues using an acidic sodium sulphite-thiourea system. Minerals Engineering 14(2): 263-268. [29] Yao, S.Z., He, F.J., Nie, L.H. 1992. Piezoelectric determination of traces of thiourea. Analytica Chimica Acta 268: 311-314. [30] Tremblay, L., Deschenes, Ghali, E., McMullen, J., Lanouette, M. International Journal of Mineral Processing 48: 225-244. [31] la Brooy, S.R., Linge, H.G., Walker, G.S. 1994. Review of Gold Extraction from Ores. Minerals Engineering 7(10): 1213-1241. [32] Schultz, R.G. 1984. New aspects of thiourea leaching of precious metals. Journal of Metals 36(6): 62-65. [33] Schultz, R.G. 1986. Thiourea leaching of precious metals. Erzmetall 39(2): 57-59. [34] Abbruzzese, C., Fornari, P., Massidda, R., Ubaldini, S. 1997. A complete scheme of treatment of gold-bearing ores for gold recovery by thiourea leaching, pp 357-365, in Proceedings of the XX International Mineral Processing Congress (eds. H. Hoberg and von Blottnitz), Germany. [35] Gonen, N., Kekec, K., Kizilkaya, B., Yildirim, M. 1998. Leaching of Gumushane- Mstra ore with thiourea, pp. 561-565, in Proceedings of the 7th International Mineral Processing Symposium (eds. Atak et al.), Turkey. [36] Miroslav, S., Magdalena, S., Jarmila, H. 1997. Thiourea in gold leaching from copper slimes, pp. 142-147, in Metallurgy East-West ’97 (ed. J. Schmiedi et al.), Slovakia. [37] Orgui, S., Atalay, U. 2000. Gold extraction from Kaymaz gold ore by thiourea leaching, pp. 22-28, in Proceedings of the XXI International Mineral Processing Congress (ed. P Massacci), Rome. [38] Abramov, A.A. 1998. Technology of gold recovery from ores in Russia, pp 503-508, in Proceedings of the 7th International Mineral Processing Symposium (ed. Atak et al.), Turkey, A.A. Balkema. [39] Swaminathan, C., Pyke, P., Johnston, R.F. 1993. Reagent Trends in the Gold Extraction Industry. Minerals Engineering 6(1): 1-16. [40] Lacoste-Bouchet, P., Deschenes, G., Ghali, E. 1998. Thiourea leaching of a copper- gold ore using statistic design. Hydrometallurgy 47: 189-203. [41] White, H.A. 1905. The solubility of gold in thiosulphates and thiocyanates. Journal of the Chemical, Metallurgical and Mining Society of South Africa 6: 109-111. [42] Barbosa-Filho, O., Monhemius, A.J. 1994. Iodine-thiocyanate leaching system for gold, in Hydrometallurgy ’94, pp. 425-440, Institution of Mining and Metallurgy, UK.</p><p>14 [43] Fleming, C.A. 1986. A process for simultaneous recovery of gold and uranium from South African ores, in Gold 100 – Proceeding of the International Conference on Gold. Volume 2: Extractive Metallurgy of Gold, pp. 301-309, South African Institution of Mining and Metallurgy, Johannesburg. [44] Kuzugudenli, O.E., Kantar, C. 1999. Alternatives to gold recovery by cyanide leaching. Erc. Univ. Fen Bil. Derg. 15(1-2): 119-127. [45] Barbosa-Filho, O., Monhemius, A.J. 1994. Leaching of gold in thiocyanate solutions – Part 1: chemistry and thermodynamics. Transactions of the Institution of Mining and Metallurgy 103: C105-110. [46] Broadhurst, J.L., du Preez, J.G.H. 1993. A thermodynamic study of the dissolution of gold in the acidic aqueous thiocyanate medium using iron(III) sulphate as an oxidant. Hydrometallurgy 32: 317-344. [47] Barbosa-Filho, O., Monhemius, A.J. 1994. Leaching of gold in thiocyanate solutions – Part 2: redox processes in iron(III)-thiocyanate solutions. Transactions of the Institution of Mining and Metallurgy 103: C111-116. [48] Barbosa-Filho, O., Monhemius, A.J. 1994. Leaching of gold in thiocyanate solutions – Part 3: rates and mechanism of gold dissolution. Transactions of the Institution of Mining and Metallurgy 103: C117-125. [49] Kholmogorov, A.G., Kononova, O.N., Pashkov, G.L., Kononov, Y.S. 2002. Thiocyanate solutions in gold technology. Hydrometallurgy 64: 43-48. [50] Monhemius A.J., Ball S.P. 1995. Leaching of Dominican gold ores in iodide- catalysed thiocyanate solutions. Transactions of the Institution of Mining and Metallurgy 104: C117-124. [51] Aylmore, M.G. 2001. Treatment of a Refractory Gold-Copper Sulphide Concentrate by Copper Ammoniacal Thiosulfate Leaching. Minerals Engineering 14(6): 615-637. [52] Breuer, P.L., Jeffrey, M.I. 2000. Thiosulfate Leaching Kinetics of Gold in the Presence of Copper and Ammonia. Minerals Engineering 13(10-11): 1071-1081. [53] Abbruzzese, C., Fornari, P., Massidda, R., Vegliò, F., Ubaldini, S. 1995. Thiosulphate leaching for gold hydrometallurgy. Hydrometallurgy 39: 265-276. [54] Feng, D., Van Deventer, J.S.J. 2002. Leaching behaviour of sulphides in ammoniacal thiosulphate systems. Hydrometallurgy 63: 189-200. [55] Alymore, M.G., Muir, D.M. 2001. Thiosulfate Leaching of Gold – A Review. Minerals Engineering 14(2): 135-174. [56] Flett, D.S., Derry, R., Wilson, J.C. Chemical study on the thiosulphate leaching of sulfide. Transactions of IMM, Part C 92: 282-293. [57] Von Michaelis, H. Thiosulfate leaching of gold and silver. Randol Phase IV Report, Randol International, Golden, Colorado [58] Gelves, G.A., Arias, V.A., Pedraza, J.E. 1996. Recovering of refractory gold using ammonium thiosulphate solutions, pp. 477-487, in Clean Technology for the mining industry (ed. M.A. Sanchez and S.H. Castro), University of Concepcion. [59] Wan, R.Y. 1997. Importance of solution chemistry for thiosulphate leaching of gold, pp. 159-162, in World Gold ’97, Australian IMM, Victoria. [60] Breuer, P.L., Jeffrey, M.I., Choo, W.L. 2001. Fundamental Aspects of the Gold Thiosulphate Leaching Process, pp. 455-468, in Cyanide: Social, Industrial and Economic Aspects, pp. 501-511, Minerals and Materials Society, New Orleans. [61] Yen, W.T., Guo, H., Deschenes, G. 1999. Development in percolation leaching with ammonium thiosulphate for gold extraction of a mild refractory ore, pp. 441-455, in Proceedings of a symposium held in San Diego, California (ed. B. Mishra), Minerals, Metals and Materials Society, Pennsylvania. [62] Langhans, J.W., Blake, B.D. 1996. Gold extraction from low grade carbonaceous ore using thiosulphate, pp. 85-96, in Practical aspects of international management and </p><p>15 processing (ed. G.E. McClelland et al.), Society for Mining, Metallurgy and Exploration, Colorado. [63] Balaz, P. Ficeriova, J., Boldizarova, E., Haber, M., Jelen, S., Kammel, R. 2000. Thiosulphate leaching of gold from a mechanochemically pretreated complex sulphide concentrate, pp. A6-74 – A6-81, in Proceedings of the XXI International Mineal Processing Congress (ed. P. Massacci), Rome. [64] Liu, K., Shibayama, A., Suzuki, T., Yan, W., Fujita, T. 2001. Gold leaching by using ammonium thiosulphate solution. Journal of Min. Mater. Process. Inst. Japan 117(3): 221-225. [65] Panayotov, V.T. 1996. A technology for thiosulphate leaching of Au and Ag from pyrite concentrates, pp. 563-565, in Proceedings of the 6th international mineral processing symposium (ed. M. Kemal et al.), A.A. Balkema, Rotterdam. [66] Calvez, J.P.S., Kim, M.J., Wong, P.L.M., Tran, T. 1998. Use of Coal-Oil Agglomerates for Particulate Fold Recovery. Minerals Engineering 11(9): 803-812. [67] Kotze, W., Petersen, F.W. 2000. Free gold recovery by coal-oil agglomeration. Journal of the South African Institute of Mining and Metallurgy 100(1-2): 57-62. [68] Bellamy, S.R., House, C.I.T., Veal, I.G. 1989. Recovery of fine gold by coal gold agglomeration, in World Gold ’89, Australia. [69] Bonney, C.F. 1992. Coal-gold agglomeration – a novel approach to gold recovery, pp. 2301-2308, in Innovations in Gold and Silver Recovery, Randol, Chapter 16. [70] Marciano, A., Costa, L.S.N., Lins, F.F. 1994. Utilization of Coal-Oil Agglomerates to Recover Gold Particles. Minerals Engineering 7(11): 1401-1409. [71] Moses, L.B., Petersen, F.W. 2000. Flotation as a Separation Technique in the Coal Gold Agglomeration Process. Minerals Engineering 13(3): 255-264. [72] Tran, T., Lee, K., Fernando, K. 2001. Halide as an alternative lixiviant for gold processing – an update, pp. 501-508, in Cyanide: Social, Industrial and Economic Aspects, Minerals and Materials Society, New Orleans. [72] Ramadorai, G. 1993. Use of chlorine in the processing of gold ores, pp. 265-279, in Precious Metals 1993 (ed. R.K. Mishra). International Precious Metals Institute, Pennsylvania. [73] Tran, T., Davis, A. 1992. Fundamental aspects on the leaching of gold in halide media, pp. 99-113, in EPD Congress 1992 (ed. P.A. Warrendale), Minerals, Metals and Materials Society, USA. [74] Tan, T., Davis, A., Song, J. 1992. Extraction of gold in halide media, pp. 323-327, in Extractive Metallurgy of Gold and Base Metals, Australian Institute of Mining and Metallurgy, Victoria. [75] Huff, R.V., Boughman, D.R. 1994. Chloride process for precious metals recovery, pp. 657-664, in In Situ Recovery of Minerals II (ed. S.A. Swan and K.R. Coyne), New York. [76] Ximing, L., Jiajun, K., Xinhui, M., Bin, L. 1992. Chlorine leaching of gold-bearing sulphide concentrate and its calcine. Hydrometallurgy 29: 205-215. [77] Marsden, J., House, I. 1992. The Chemistry of Gold Extraction, Ellis Horwood Ltd., England. [78] Jena, P.K., Brocchi, E.A. 1997. Metal Extraction Through Chlorine Metallurgy. Mineral Processing and Extractive Metallurgy Review 16: 211-217.</p><p>16</p>
Details
-
File Typepdf
-
Upload Time-
-
Content LanguagesEnglish
-
Upload UserAnonymous/Not logged-in
-
File Pages16 Page
-
File Size-