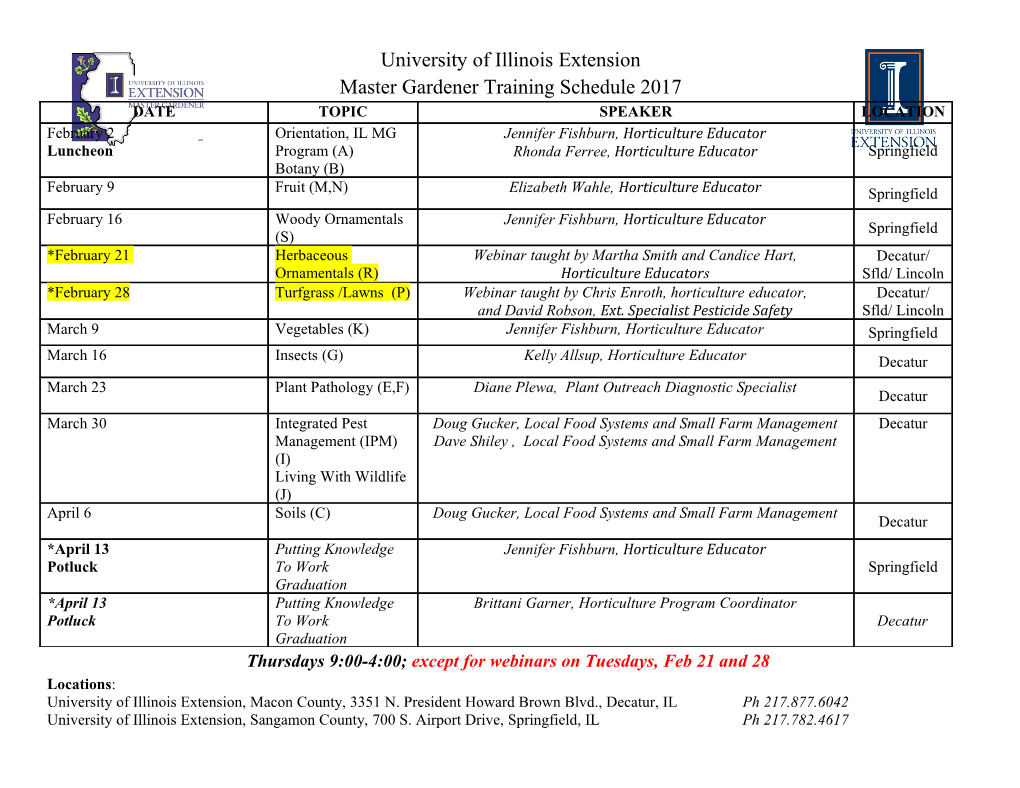
<p>ASEN5116 Spacecraft Life Support Systems</p><p>FINAL TRADE STUDY REPORT</p><p>ECLSS System for the NASA Mars Design Reference Mission</p><p>December 16, 2002</p><p>By Earth Replications, Inc.</p><p>Contributing Engineers: Vanessa Aponte ERI Steve Chappell Markus Czupalla Nancy Kungsakawin Nicholee Page Jesse Riggert Tommy Romano</p><p>ASEN5116 Final Report Spacecraft Life Support Systems TABLE OF CONTENTS 1.0 INTRODUCTION...... 1 2.0 SYSTEM & MISSION REQUIREMENTS...... 1 2.1 Mission Overview...... 1 2.2 System Overview & Requirements Summary...... 1 2.3 Metabolic Load Basis...... 2 2.4 Baseline System Architecture...... 3 3.0 SYSTEM & MISSION ASSUMPTIONS...... 6 4.0 TRADE STUDY PROCESS & PHILOSOPHY...... 6 4.1 Identification of Requirements...... 7 4.2 Selection of Trade Variables...... 7 4.3 Determination of Weighting Factors...... 8 4.4 Specification Sheets...... 8 4.5 Data Analysis...... 8 4.6 Results...... 9 5.0 ATMOSPHERE MANAGEMENT...... 10 5.1 Baseline Functional Requirements...... 10 5.2 Baseline Architecture...... 10 5.3 Subsystem Assumptions...... 11 5.4 Candidate Technologies...... 11 5.5 Subsystem Technology Trade Studies & Design Decisions...... 13 5.5.1 C02 Reduction & Removal, O2 Provision, N2 Provision...... 14 5.5.2 Trace Contaminant Control...... 15 5.5.3 Temperature & Humidity Control...... 15 5.5.4 Ventilation...... 16 5.5.5 Monitoring & Control...... 16 5.5.6 Fire Detection & Suppression...... 16 5.5.7 Results...... 17 5.5.8 Conclusions and Recommendations...... 19 5.6 Atmosphere Management Subsystem References...... 19 6.0 WATER MANAGEMENT...... 21 6.1 Water Management...... 21 6.2 Water Monitoring...... 22 6.2.1 Flow Chart for Water Monitoring...... 25 6.3 Water Generation...... 26 6.4 Water Processing...... 27 6.4.1 Fire Detection & Suppression...... 27 6.4.2 Baseline Architecture...... 28 6.4.3 Potable and Hygiene Water Processing...... 29 6.4.3.1 Candidate Technologies...... 29 6.4.3.2 Infeasible Technologies...... 29 6.4.3.3 Technology Trade Study...... 29 6.4.4 Urine Processing...... 30 6.4.4.1 Candidate Technologies...... 30 6.4.4.2 Infeasible Technologies...... 30 6.4.4.3 Technology Trade Study...... 30</p><p>Earth Replications, Inc. Page i ASEN5116 Final Report Spacecraft Life Support Systems</p><p>6.4.5 Closed Loop Architecture...... 31 6.5 Conclusions and Recommendations...... 33 6.6 Water Management Subsystem References...... 33 7.0 WASTE MANAGEMENT...... 34 7.1 Baseline Functional Requirements...... 34 7.2 Baseline Architecture...... 34 7.3 Subsystem Assumptions...... 35 7.4 Subsystem Technology Trade Studies...... 35 7.4.1 Candidate Technologies...... 36 7.5 Results...... 39 7.5.1 Batch and Continuous Incineration (BI, CI)...... 40 7.5.2 Electrochemical Oxidation (EO)...... 41 7.5.3 Gasification (GAS)...... 41 7.5.4 Supercritical Water Oxidation (SCWO)...... 41 7.5.5 Pyrolysis (PYRO)...... 41 7.5.6 Plasma Arc Oxidation (PAO)...... 41 7.5.7 Trade Study Process...... 42 7.6 The Integrated System...... 43 7.7 Failure Analysis...... 45 7.8 Conclusions and Recommendations...... 45 7.9 Waste Management Subsystem References...... 46 8.0 FOOD SUPPLY...... 48 8.1 Baseline Functional Requirements...... 48 8.2 Baseline Architecture...... 50 8.3 Subsystem Assumptions...... 52 8.4 Candidate Technologies...... 52 8.5 Subsystem Technology Trade Study...... 53 8.5.1 Results...... 54 8.6 Food Supply Subsystem References...... 55 9.0 SYSTEM INTEGRATE DESIGN RESULTS...... 57 9.1 System Architecture...... 57 9.2 Mass Balance Results...... 60 9.3 Equivalent System Mass Results...... 60 10.0 SYSTEM CONCLUSIONS & RECOMMENDATIONS...... 63 11.0 APPENDIX A: REQUIREMENTS...... 64 11.1 Atmosphere Management...... 69 11.2 Water Management...... 74 11.3 Waste Management...... 75 11.4 Food Supply...... 76 12.0 SUBSYSTEM PHYSICAL/CHEMICAL PROCESS FLOW SCHEMATICS...... 77 12.1 Atmosphere Management...... 77 12.2 Water Management...... 109 12.3 Waste Processing...... 123</p><p>Earth Replications, Inc. Page ii ASEN5116 Final Report Spacecraft Life Support Systems</p><p>TABLE OF FIGURES Figure 2-1 Human Input / Output Diagram (NASA Tech 109927, 1994)...... 3 Figure 2-2 Open Loop Subsystem Mass, Volume and Power Totals...... 4 Figure 2-3 Open Loop System Diagram...... 5 Figure 4-1 Trade Study Process...... 7 Figure 5-1 Open Loop AM Subsystem...... 11 Figure 5-2 AM Subsystem Diagram Transit...... 17 Figure 5-3 AM Subsystem Parameters...... 18 Figure 5-4 AM Subsystem Diagram - Mars Surface...... 18 Figure 6-1 The Order of Monitoring Devices...... 26 Figure 6-2 Open Loop Diagram w/ Flow Rates in kg/day for 6 Crewmembers...... 28 Figure 6-3 Closed Loop Water Management Flow Diagram...... 31 Figure 7-1 WP Open Loop Diagram...... 35 Figure 8-1 Food Subsystem Baseline Open Loop Design...... 50 Figure 8-2 Food Supply Comparison Graph...... 53 Figure 8-3 Closed Loop Design for Food Supply Subsystem...... 54 Figure 9-1 System Block Diagram – Transit...... 57 Figure 9-2 System Block Diagram - Surface...... 58 Figure 9-3. Final Design Subsystem Mass, Power and Volume...... 60</p><p>Earth Replications, Inc. Page iii ASEN5116 Final Report Spacecraft Life Support Systems</p><p>1.0 INTRODUCTION This trade study report was written for the Fall 2002 semester Spacecraft Life Support Design course at the University of Colorado Department of Aerospace Engineering Sciences. It is a preliminary analysis of current life support system technologies and the design of an integrated system. It was based on the NASA Design Reference Mission (DRM) for Mars, Addendum 3.0. This report is intended to summarize the results of the analysis and design activities. The responsibilities and personnel involved in this study are shown in Table 1-1</p><p>Table 1-1 Personnel and Responsbilities Responsibility Personnel Program Manager Tommy Romano Systems Engineer Steve Chappell Atmosphere Management (AM) Steve Chappell & Markus Czupalla Food Supply (FS) Tommy Romano Waste Processing (WP) Vanessa Aponte & Nicholee Page Water Management (WM) Nancy Kungsakawin & Jesse Riggert</p><p>2.0 SYSTEM & MISSION REQUIREMENTS 2.1 Mission Overview The mission for which the ECLSS is designed is based on the NASA Design Reference Mission (DRM) for Mars. The mission is a multistage mission that will send 6 astronauts to live on the surface of Mars for an extended period. The overall mission requires the use of several vehicles that must contain an ECLSS: an Earth Return Vehicle, a Crew Transit/Surface Habitat, and a Crew Ascent Vehicle. The Crew Transit/Surface Habitat will be used to transport the crew of 6 from Earth to the Mars surface. After the Mars surface stay, the Crew Ascent Vehicle will be used to leave the surface of Mars and rendezvous with the Earth Return Vehicle already in orbit about Mars. Once the crew is on-board the Earth Return Vehicle, it will perform the transfer and bring the crew back to Earth. The DRM also identifies overall mission parameters and constraints, such as the need for the systems to be able to operate without re-supply for the duration of the mission. For further details, a full listing of mission requirements is given in Appendix A. 2.2 System Overview & Requirements Summary The ECLSS system design encompasses the life support system on board the Crew Transit/Surface Habitat. As specified by the DRM, the life support system onboard the Earth Return Vehicle will be a duplicate of the Crew Transit/Surface Habitat that arrives in orbit around Mars prior to the departure of the Crew Transit/Surface Habitat with the crew onboard.</p><p>The ECLSS is broken down into four subsystems:</p><p>Earth Replications, Inc. Page 1 ASEN5116 Final Report Spacecraft Life Support Systems</p><p> Atmosphere Management (AM), Food Supply (FS), Waste Processing (WP), and Water Management (WM).</p><p>These four subsystems work together in an integrated system that provides all of the necessary functions of the ECLSS. Specific functional and derived requirements for each subsystem are provided in the subsystem sections as well as in Appendix A. The main system-level driving requirements are: Provide life support functions for a crew of 6; 180 worst-case transit time between Earth and Mars, 600 day worst-case surface stay on Mars, Perform the entire mission assuming no resupply from Earth, Take advantage of ISRU when possible, Target life support system mass of 4661 kg + consumable weight, Target life support system power usage of 12.1 kW, Operate during launch, transit, descent, and surface g-loads, Provide 2 levels of backup redundancy, Do not rely on biological systems for life support functions, Provide as much loop closure as possible, Reliability, maintainability, and safety.</p><p>These driving requirements were primary considerations in the overall design. Other derived requirements were placed on the system and subsystems to define the system further. These details can be found in the subsystem sections and in Appendix A. 2.3 Metabolic Load Basis Figure 2-1 shows the metabolic input and output of each crew member. These numbers were based on chemical compositions of the input and output constituents. Values used in the following sections are based on these baseline values in calculations requiring consumables and processables.</p><p>Earth Replications, Inc. Page 2 ASEN5116 Final Report Spacecraft Life Support Systems</p><p>Inputs Outputs</p><p>Total For Total For Total Six Six Total Safety kg/per- People People kg/per- Safety Dry Food kg/per-day Factor day (kg) (kg) day Factor kg/per-day Solid Wastes Protein C4H5ON C2H6O2N2 Urine Carbon 0.0770 1.1 0.0847 0.5082 0.1056 0.0176 1.1 0.0160 Carbon Hydrogen 0.0081 1.1 0.0089 0.0535 0.0264 0.0044 1.1 0.0040 Hydrogen Oxygen 0.0257 1.1 0.0283 0.1696 0.1406 0.02343 1.1 0.0213 Oxygen Nitrogen 0.0225 1.1 0.0248 0.1485 0.1234 0.02057 1.1 0.0187 Nitrogen</p><p>Carbohydrates C6H12O6 C42H69O13N5 Feces Carbon 0.1489 1.1 0.1638 0.9827 0.1168 0.01947 1.1 0.0177 Carbon Hydrogen 0.0250 1.1 0.0275 0.1650 0.0158 0.00264 1.1 0.0024 Hydrogen Oxygen 0.1984 1.1 0.2182 1.3094 0.0482 0.00803 1.1 0.0073 Oxygen</p><p>HUMAN 0.0158 0.00264 1.1 0.0024 Nirogen</p><p>Fat C16H32O2 </p><p>Carbon 0.0858 1.1 0.0944 0.5663 C13H28O13N2 Sweat Hydrogen 0.0144 1.1 0.0158 0.0950 0.0488 0.00814 1.1 0.0074 Carbon Oxygen 0.0143 1.1 0.0157 0.0944 0.0092 0.00154 1.1 0.0014 Hydrogen 0.0653 0.01089 1.1 0.0099 Oxygen</p><p>Liquids (H2O) 0.0086 0.00143 1.1 0.0013 Nitrogen Potable </p><p>Hydrogen 0.1802 1.1 0.1982 1.1893 Liquid (H20) Oxygen 1.4298 1.1 1.5728 9.4367 Urine Food Prep 1.1174 0.18623 1.1 0.1693 Hydgrogen Hydrogen 0.0884 1.1 0.0972 0.5834 8.8704 1.4784 1.1 1.3440 Oxygen Oxygen 0.7016 1.1 0.7718 4.6306 </p><p>Food Content Feces Hydrogen 0.1287 1.1 0.1416 0.8494 0.0673 0.01122 1.1 0.0102 Hydrogen Oxygen 1.0213 1.1 1.1234 6.7406 0.5320 0.08866 1.1 0.0806 Oxygen</p><p>Gases Air Sweat & Perspiration Oxygen 0.8359 1.1 0.9195 5.5169 1.6988 0.28314 1.1 0.2574 Hydrogen 13.4831 2.24719 1.1 2.0429 Oxygen</p><p>Hygiene H2O 9 1.1 9.9000 59.4000 </p><p>Laundry H2O 12.5 1.1 13.7500 82.5000 Gases 11846 kJ/per-day CO2 Carbon Dioxide Total weight per day 174.9396 kg Heat Load 71076 kJ/6per-day 1.7860 0.29766 1.1 0.2706 Carbon Total weight per year 63852.95 kg 25942740 kJ/6per-year 4.7579 0.79299 1.1 0.7209 Oxygen</p><p>59.4000 9.9 1.1 9 Hygiene H2O</p><p>82.5000 13.75 1.1 12.5 Laundry H2O</p><p>Mass Balance 0.0020 174.9376 kg Total weight per day 63852.23 kg Total weight per year Figure 2-1 Human Input / Output Diagram (NASA Tech 109927, 1994) 2.4 Baseline System Architecture The baseline open loop ESM was calculated with the information provided in Figure 2-2. This figure show the breakdown of each subsystem and its’ components and the values that were obtain or estimated for Mass, Power and Volume. The additional weight column provides inclusion of consumables, such as LiOH canisters, piping and storage tank components.</p><p>Earth Replications, Inc. Page 3 ASEN5116 Final Report Spacecraft Life Support Systems</p><p>OPEN LOOP ESM Atmosphere Add. Total Total Total Crew Weight Weight Power Power Volume Volume Time Component # Weight (kg) (kg) (kg) (kW) (kw) (m3) (m3) (hrs/day) Oxygen Consumable 1 4303.21 0.00 4303.21 0.00 0.00 0.00 0.00 0.00 Oxygen Buffer Tank 1 1463.09 0.00 1463.09 0.60 0.60 0.00 0.00 0.00 CO2 Removal 1 5104.24 0.00 5104.24 0.00 0.00 0.00 0.00 0.67 LiOH 1 5548.09 1109.62 6657.71 0.00 0.00 0.00 0.00 0.00 Nitrogren Consumable 1 1288.00 0.00 1288.00 0.00 0.00 0.00 0.00 0.00 Nitrogen Buffer Tank 1 695.52 0.00 695.52 0.60 0.60 0.00 0.00 0.00 Totals 19511.77 1.20 0.00 0.67</p><p>Water Add. Total Total Total Crew Weight Weight Power Power Volume Volume Time Component # Weight (kg) (kg) (kg) (kW) (kw) (m3) (m3) (hrs/day) Potable Water 1 128957.40 0.00 128957.40 0.00 0.00 0.00 0.00 0.50 Water Tank 1 43845.52 0.00 43845.52 0.00 0.00 0.00 0.00 0.00 Totals 172802.92 0.00 0.00 0.50</p><p>Waste Add. Total Total Total Crew Weight Weight Power Power Volume Volume Time Component # Weight (kg) (kg) (kg) (kW) (kw) (m3) (m3) (hrs/day) Toilet assembly 1 16.00 19.20 35.20 0.22 0.22 0.08 0.08 0.08 Septic tank 1 14.50 2.90 17.40 0.00 0.00 0.10 0.10 0.00 Compactor 1 15.10 10.84 25.94 0.11 0.11 0.36 0.36 0.00 Non-processables Storage 1 50.00 0.00 50.00 0.00 0.00 2.00 2.00 0.08 Totals 128.54 0.33 2.53 0.17</p><p>Food Add. Total Total Total Crew Weight Weight Power Power Volume Volume Time Component # Weight (kg) (kg) (kg) (kW) (kw) (m3) (m3) (hrs/day) Dehydrated Food 1 3192.27 420.00 3612.27 0.00 0.00 37.00 37.00 3.50 Microwave 2 70.00 14.00 154.00 1.80 3.60 0.15 0.30 0.00 Totals 3766.27 3.60 37.30 3.50</p><p>Grand Totals 196209.51 5.13 39.83 4.83</p><p>Figure 2-2 Open Loop Subsystem Mass, Volume and Power Totals</p><p>By using the Equations (1), the total system ESM was calculated to be 196,663 kg.</p><p>ESM total TotalMass 87 * Power 1.54*CrewTime (1)</p><p>This mass was used as the baseline of comparison for the developed system to see the improvements made as more loop closure is obtained. A diagram depicting the baseline open-loop system is shown in Figure 2-3.</p><p>Earth Replications, Inc. Page 4 ASEN5116 Final Report Spacecraft Life Support Systems</p><p>Food</p><p>Storage Processing Non- Waste Biological Chemical Dump 0.614 Biological Preparation Stabilization</p><p>Spent LiOH 13.678 Storage 5.214 16.897 Waste Water 167.669</p><p>6.544 Solid Human Waste Atmosphere 5.517 0.724</p><p>CO2 Reduction</p><p>O2 Storage M 10.626 M o 82.5 Laundry o Water n N Storage n</p><p>2 i i t Pressure t o</p><p>59.4 Hygiene o r Control r Storage i Storage i n Ventilation Potable n g g Monitoring All values are in kg/day for 6 FDS THC TCC crew members</p><p>Figure 2-3 Open Loop System Diagram</p><p>Earth Replications, Inc. Page 5 ASEN5116 Final Report Spacecraft Life Support Systems</p><p>3.0 SYSTEM & MISSION ASSUMPTIONS Several assumptions were made at the system level that affected the overall design. The main assumptions were: Mission Duration: The DRM listed several different values for the length of the transit phase, ranging from 120 days to 180 days. Additionally, the surface stay was listed as from 500-600 days. For this design, worst case was assumed for the transit and the surface stay, 120 days and 600 days, respectively. Safety Buffer: Since a detailed analysis on appropriate safety buffers for each technology was not performed, a 10% safety buffer was assumed for all subsystems. In-Situ Resource Utilization (ISRU): The DRM details the contents of an ISRU plant that will be taken to Mars on board the Cargo Lander. The ISRU plant contains equipment to produce resources from the Martian atmosphere. It was assumed that this plant is in place and that it will be interfaced with the Crew Transit/Surface Habitat upon landing. Duplicate Systems: It was assumed that the Crew Transit/Surface Habitat and the Earth Return Vehicle have duplicate life support systems and that each system only has to handle a one-way transfer of 180 days. 4.0 TRADE STUDY PROCESS & PHILOSOPHY The trade study process used in the selection of the technologies for the ECLSS consists of several steps. The steps of the process are depicted in Figure 4-4 and explained in the remainder of this section.</p><p>Earth Replications, Inc. Page 6 ASEN5116 Final Report Spacecraft Life Support Systems</p><p>Requirements</p><p>Technology Research</p><p>Spec Spec Sheets Sheets •Mass •Power •Volume Trade Trade •TRL Matrix Matrix •Op. Temp. •Op. Pressure •Heat Integrate System</p><p>Assess System</p><p>Final System</p><p>Figure 4-4 Trade Study Process 4.1Identification of Requirements The requirements for the subsystems were first identified. The high level mission and system requirements were derived mainly from the NASA DRM for Mars. The high- level mission and system requirements were flowed down and allocated to the subsystems where applicable. Additional requirements for the subsystems have been derived from analysis of existing systems and systems undergoing research and development. 4.2Selection of Trade Variables The trade variables used in the subsystem’s trade studies are based on the subsystem’s requirements and typical assessment criteria for manned space systems. Qualitative and quantitative criteria were taken into account via the identified trade variables. The trade variables are a subset of the overall specification data collected for each candidate technology. Table 4-2 shows a general table of trade variables that are later modified based upon the subsystem and specific trade.</p><p>Earth Replications, Inc. Page 7 ASEN5116 Final Report Spacecraft Life Support Systems</p><p>Table 4-2 General Trade Study Variables Trade Variables Component Weight (kg) Additional Weight (kg) Power (kW) Volume (m3) Heat Generated (kW) Operating Temperature (K) Operating Pressure (kPa) Designed Efficiency (%) TRL (1-9) Reliability Maintainability Gravity Dependence Crew Time - Operations Safety Lifetime Cost Prospective Improvements</p><p>4.3Determination of Weighting Factors Weighting factors were applied to the trade variables to be used in the technology candidate selection process. These served to set the relative importance of a particular trade variable in relation to the others. Assignment of weighting factors allowed for comparison of all necessary trade variables without less important trade variables overpowering more important trade variables. The weighting criteria for trade variables were assigned by each subsystem for its trade studies. 4.4Specification Sheets Specification sheets were developed for each candidate technology containing all parameters and trade variables where data is available. The specification sheets served as the repository for the raw trade study data from which data was extracted for input to the trade matrices. See Appendix B for all of the specifications sheets for candidate technologies considered in the design. 4.5Data Analysis Trade study variables from the specification sheet for each candidate technology were extracted and input into a trade matrix for each required function. The trade matrices assessed how well each candidate technology will meet the optimal trade variables. Weighting factors were applied to adjust the overall importance of particular trade variables. Additionally, an uncertainty percentage was applied to each input value for the trade variables so that the sensitivity to each variable could be more readily assessed. The uncertainty percentage allowed maximum, nominal, and minimum values to be computed to assess the impact of the uncertainty on the trade matrix outcome. Additionally, the trade variable input values were scored based upon a linear normalization; i.e. the scores for each value ranged from 0.0-1.0. The highest score </p><p>Earth Replications, Inc. Page 8 ASEN5116 Final Report Spacecraft Life Support Systems achieved was intended to be the winner. Scoring and normalization for each trade variable is shown in Table 4-3. Table 4-3 Scoring & Normalization Scheme Trade Variables Scoring/Normalization Component Weight (kg) 1 for lowest & 0 for highest Additional Weight (kg) 1 for lowest & 0 for highest Power (kW) 1 for lowest & 0 for highest Volume (m3) 1 for lowest & 0 for highest Heat Generated (kW) 1 for lowest & 0 for highest Operating Temperature (K) 1 for lowest & 0 for highest Operating Pressure (kPa) 1 for lowest & 0 for highest Designed Efficiency (%) 1 for highest & 0 for lowest TRL (1-9) 1 for highest & 0 for lowest Reliability 1 for highest & 0 for lowest Maintainability 1 for highest & 0 for lowest Gravity Dependence 1 for ‘no’ & 0 for ‘yes’ Crew Time - Operations 1 for lowest & 0 for highest Safety 1 for highest & 0 for lowest Lifetime Cost 1 for lowest & 0 for highest Prospective Improvements 1 for highest & 0 for lowest</p><p>4.6Results The results of the trade studies took into account the data analysis of the trade variables using the trade matrices. Additionally, other program and mission level considerations affected the overall decision. A description of the selected technologies is supplied as well as rationale for the selections in the subsystem sections of this document.</p><p>Earth Replications, Inc. Page 9 ASEN5116 Final Report Spacecraft Life Support Systems</p><p>5.0 ATMOSPHERE MANAGEMENT The Atmosphere Management (AM) subsystem is designed to continuously control and regenerate the module atmosphere. It monitors the status of the atmosphere for gas composition as well as any contaminants deemed harmful. Additionally, it serves as a warning mechanism through the detection and suppression of on-board fires. The following sections will go over the requirements, process, and results of the design of the AM subsystem. 5.1 Baseline Functional Requirements The atmosphere management functions of the ECLSS are provided by the AM subsystem and include the following:</p><p> CO2 Removal</p><p> CO2 Reduction</p><p> O2 Provision</p><p> N2 Provision Atmosphere Monitoring and Control Gas Storage Ventilation Trace Contaminant Control Temperature and Humidity Control Fire Detection and Suppression The AM subsystem interfaces with the other subsystems of the ECLSS as necessary to provide the overall functions required for life support. For additional details on the requirements of the AM subsystem see Appendix A. 5.2 Baseline Architecture The baseline architecture of the AM subsystem is based upon a completely open loop design. Figure 5-5 shows the architecture. It includes processing of CO2 by LiOH only. This produces 13.6 kg/day of spent LiOH for 6 people. N2 and O2 provision is covered completely by cryogenic storage. The remainder of the functions of the AM subsystem is supplied by existing systems in use on ISS or STS (e.g. trace contaminant control system, condensing heat exchanger, etc.). This system was used as the basis of comparison as the system was modified to a closed-loop configuration.</p><p>Earth Replications, Inc. Page 10 Food</p><p>Storage Processing Non- Waste Biological Chemical Dump 0.614 Biological Preparation Stabilization</p><p>Spent LiOH ASEN5116 13.678 Final Report Storage Spacecraft Life Support Systems 5.214 16.897 Waste Water 167.669 Spent LiOH = 13.6 kg/day 6.544 Solid Waste 5.517 Human Atmosphere 5.517 0.724</p><p>CO2 Reduction 6.5 kg/day CO2</p><p>O2 Storage 5.5 kg/day O2 M 10.626 M o 82.5 Laundry o Water n N Storage n</p><p>2 i i t Pressure t o 59.4 Hygiene o r Control r Storage i Storage i n Ventilation Potable n g g Monitoring All values are in kg/day for 6 FDS THC TCC crew members</p><p>Figure 5-5 Open Loop AM Subsystem 5.3 Subsystem Assumptions The following assumptions were significant in the design of the AM subsystem: ISRU via extra life support equipment on the precursor cargo mission is available. This assumption significantly reduced the size and weight of the AM subsystem by designing them for the 180-day transfer (with safety buffer). The ISRU equipment on the precursor cargo mission will provide EVA gas makeup. This allows for much smaller N2 and O2 stores on the transfer vehicle. Thermal control equipment to support the cabin air temperature and humidity control system is available as part of the other vehicle systems and is not included in the life support system</p><p>5.4 Candidate Technologies A list of candidate technologies was established to satisfy the requirements for the AM subsystem. The candidate technologies are identified in Table 5-4 and are mapped to the function for which they were investigated to provide; in bold are the technologies that were analyzed more in depth for this design. Many technologies were eliminated from the study based on the lack of information, low technology readiness level, and other design factors. The following sections go over further trade studies and design decisions that were used to narrow the technology options.</p><p>Earth Replications, Inc. Page 11 ASEN5116 Final Report Spacecraft Life Support Systems</p><p>Table 5-4 Candidate Technologies for Atmosphere Management Atmosphere Management Candidate Technologies Function</p><p>CO2 Removal 2-Bed Molecular Sieve20</p><p> 4-Bed Molecular Sieve20</p><p> Electrochemical Depolarization Concentration (EDC) 20</p><p> Air Polarized Concentration (APC) 20</p><p> Solid Amine Water Desorption (SAWD) 20</p><p> LiOH20</p><p> Temperature Swing Adsorption6,5,21,22</p><p> Osmotic Membranes20</p><p> Electroactive Carriers20</p><p> Metal Oxides20</p><p> Carbonate20</p><p> Ion-Exchange Electrodialysis20</p><p> Sodasorb20</p><p> Superoxides20</p><p> Solid Oxide Fuel Cells8,15,3</p><p> Pressure Swing Adsorption1</p><p>CO2 Reduction Bosch20</p><p> Sabatier20</p><p> Advanced Carbon Formation Reactor20</p><p> Solid Oxide Electrolysis23</p><p> CO2 Electrolysis20</p><p> Superoxides20</p><p> Iron Direct Reduction1</p><p> Reverse Water Gas Shift Reaction with Microreactors25</p><p></p><p>O2 Provision High Pressure Storage Tanks20</p><p> Cryogenic Storage Tanks20</p><p> Solid Polymer Water Electrolysis (SPWE) 20</p><p> Solid Oxide Electrolysis23</p><p> H20 Electrolysis20</p><p> Static Feed Water Electrolysis (SFWE) 20</p><p> Water Vapor Electolysis20</p><p> CO2 Electrolysis20</p><p> O2 from Chemical Compounds20</p><p> Superoxides20</p><p> Artificial Gill20</p><p> Water Splitting by Visible Light9</p><p>N2 Provision High Pressure Tanks20</p><p> Cryogenic Tanks20</p><p> Thermal Catalytic Dissociation of Hydrazine20</p><p> Dissociation of Ammonia20</p><p> Modular Pressure Swing Adsorption10</p><p> Vacuum Pressure Swing Adsorption11</p><p>Atmosphere Monitoring & Mass Spectrometer/Gas Chromatograph20</p><p>Control Ion Trap Mass Spectroscopy/ Mass Spectroscopy20</p><p> Fourier Transform IR Spectroscopy20</p><p> CFU Analyzer20</p><p>Ventilation Air Diffusers and Intakes20</p><p>Earth Replications, Inc. Page 12 ASEN5116 Final Report Spacecraft Life Support Systems</p><p>Atmosphere Management Candidate Technologies Function</p><p>Trace Contaminant Control Particulate Filters20</p><p> Activated Charcoal20</p><p> Chemiabsorbant Beds20</p><p> Catalytic Burners20</p><p> Reactive Bed Plasma (RBP) 20</p><p> Super Critical Water Oxidation20</p><p>Temperature & Humidity Condensing Heat Exchange (CHX) 20 Control</p><p>Fire Detection & Suppression Obscuration Smoke Detector20</p><p> Scattering Smoke Detector20</p><p> UV/Visible/IR Flame Detector20</p><p> CO2 Fire Suppression System20</p><p> N2 Fire Suppression System20</p><p> Halon Fire Suppression System20</p><p> Condensation Nuclei Counter20</p><p> Ionization Smoke Detector20</p><p>5.5 Subsystem Technology Trade Studies & Design Decisions The following sections go over the trade studies and design decisions that led to the selection of the technologies that make up the AM subsystem. The general trade study process described in earlier sections. For weighting criteria within the trade studies, different values were used for each function, but in general the following guidelines were followed by the AM subsystem: Mass was given a high weighting factor mainly due to its importance in launch costs; Reliability was given a high weighting factor due to the long-term, isolation aspects of the mission; TRL was given a high weighting factor due to its relevancy in tested systems; Power was given a mid-range weighting factor due to the general abundance of power from solar and nuclear sources during the mission; Volume was given a mid-range weighting factor due to the limited volume of the transfer vehicle; Medium to low weighting factors were generally assigned to operating temperature, operating pressure, prospective improvements, and maintainability. Additionally, certainty factors were applied to each value in the trade matrices with the following general guidelines: 100% Number confirmed through multiple references 75% Number from reference or based on reasonable assumptions 50% Number based on reference with questionable assumptions 25% Number based only on assumptions 0% Slightly educated guess</p><p>Earth Replications, Inc. Page 13 ASEN5116 Final Report Spacecraft Life Support Systems</p><p>These values were used to look at the sensitivity of our results based on the certainty of the input data. For further information regarding each technology and to see associated trade matrices, see Appendix B.</p><p>5.5.1 C02 Reduction & Removal, O2 Provision, N2 Provision</p><p>The design of the C02 reduction, C02 removal, O2 provision, and N2 provision systems were addressed together and were iterated upon independent from the remaining systems in the AM subsystem. Initially, the winners from the trade matrices were chosen and integrated together, adding buffer tanks, piping, etc., to achieve a baseline for the AM subsystem. Although this system was fully functional, the initial weight was estimated at 4227 kg for these functions of the AM subsystem. It was decided to investigate other options to attempt to reduce the overall mass. The options considered and implemented were: The solid oxide electrolysis system was chosen even though it did not win in the trade matrix comparison for CO2 reduction or O2 provision. However, the solid oxide electrolysis system can perform both functions. Therefore, the scores from both trade matrices were added together, showing solid oxide electrolysis to win. It is a low mass system compared with the alternatives and it eliminates the need for a CH4 storage tank (as is necessary with the Sabatier reactor). Additionally, it reduces the necessary size of the H2 tank due to the decrease in losses of H2 in CH4. The disadvantage of solid oxide electrolysis is that its power requirements are about 3 times as much as the other two candidates combined, but the benefits are still worth the change. A main assumption was to use ISRU for the provision of buffer gases. This is the reason why the storage tanks for the buffer gases are only designed for the transfer of 180 days and not for 780 days, as in the first system. The two buffer gases needed are O2 and N2. While the N2 buffer is the main source to allow for pressure maintenance, the O2 buffer takes care of the reaction inefficiencies. </p><p> The N2 buffer is sized as follows: The N2 needed to account for the whole transfer leakage of 26 kg (transfer both ways) (BVAD 1999 [page. 21]). The tank weight is 0.524 kg tank/kg gas. Therefore, 13.7 kg was arrived at for the tank weight. The volume was computed using the density of liquid Nitrogen, thus the total N2 buffer tank weight is 39.7 kg. Without being able to close the N2 loop with, for example, ammonia dissociation, ISRU is the only way to stay within reasonable mass limits. The reason is that on the surface multiple EVA´s will be performed. This accounts for a large gas loss (1280 kg due to BVAD 1999 page. 21). This gas loss is too large to be able to bring all the necessary gas from Earth. </p><p> The O2 buffer is sized as follows: A 10% loss of O2 due to reaction inefficiencies with the crew needing 5.5 kg O2 per day was assumed. With a transit of 180 days, this means 100 kg of O2 is needed. Using pressurized tanks, 0.364 kg tank/kg gas has to be added. Therefore, we arrived at 36.4 kg of additional weight for the tank. The volume is computed using the density of gaseous O2.</p><p>Earth Replications, Inc. Page 14 ASEN5116 Final Report Spacecraft Life Support Systems</p><p> Both N2 and O2 will be provided for the transfer only. On the surface of Mars, those buffer gases will be provided directly by utilizing the Martian Atmosphere. From this atmosphere, CO2 and an N2/Ar mixture can be obtained by using the Temperature Swing Adsorbtion technique (K.R. Sridhar “Utilization of Martian Atmosphere Constituents by Temperature-Swing Adsorbtion”). The CO2 will then be taken apart by the Sabatier reactor which already is in place (DRM Addendum). The H2 for the Sabatier reaction will be brought from Earth in the Cargo vehicle (DRM Addendum) until H2O sources have been located on Mars. The penalty of ISRU is that the facilities to extract gas from the Martian atmosphere needs to be added. In particular, this is the Temperature-Swing Adsorbtion plant. The weight is 72 kg, which is a small price to pay for reducing the system mass by the factor of 10. In addition, this TSA system is able to act as a backup for CO2 concentration during transit. The only thing to consider when choosing that option is that the cooling system has to be designed such that it can simulate the Martian temperature change during night and day. This task remains to be solved when designing the transfer vehicle. Even if this should turn out to be too difficult to achieve, the 72 kg is a price which one clearly would be willing to pay. 5.5.2 Trace Contaminant Control The trade study to pick the technology to be used for trace contaminant control considered a few options: Super-Critical Water Oxidation (SCWO), Reactive Bed Plasma (RBP), and a conventional Trace Contaminant Control Subsystem (TCCS). The conventional TCCS consists of activated charcoal beds, a catalytic oxidizer, a lithium hydroxide bed, a fan system, flow meters, and an electronic control interface. This type of system is in use on the ISS and is well understood, reliable, and lightweight. SCWO and RBP technologies are both universal waste-processing systems that use air as part of their processing. Since the air is subject to the same processing as the waste, the trace contaminants in the air can be destroyed and the air returned to the cabin. Therefore, they do not meet the requirement to provide continuous trace contaminant control.</p><p>The conventional TCCS was chosen to provide trace contaminant control for the life support system mainly due to its light weight, high TRL, and overall effectiveness. Additionally, HEPA filters are used in conjunction with the TCCS to provide high- efficiency particulate control as air is drawn into the ventilation system within the cabin. See Appendix B for more details on the technologies. 5.5.3 Temperature & Humidity Control Technologies to perform temperature and humidity control are limited. The most utilized and effective technology for temperature and humidity control consists mainly of a Condensing Heat eXchanger (CHX). The CHX works in conjunction with fan systems, temperature controls, check valves, water separators, and damper valves. The CHX system was chosen for temperature and humidity control for the life support system. See Appendix B for more details on the technology.</p><p>Earth Replications, Inc. Page 15 ASEN5116 Final Report Spacecraft Life Support Systems</p><p>5.5.4 Ventilation Ventilation within the crew cabin is generally performed with various fan, ducting, and valve systems. It is necessary to move air with a portion of the cabin as well as between different floors or modules of the overall cabin. Additionally, it is necessary to be able to control ventilation within specific areas for the purposes of fine-tuning the ventilation speed as well as to be able to stop the circulation of smoke in the case of fire. A system similar to the ISS was selected that uses intercabin fans, intracabin fans, and a valve control system to achieve the necessary ventilation. See Appendix B for more details on the chosen technologies. 5.5.5 Monitoring & Control The monitoring technologies involved in the trade study were: conventional ISS Major Constituent Analyzer (MCA), Gas Chromatograph/ Mass Spectrometer (GC/MS), and Fourier-Transform Infrared Spectrometer. The conventional MCA is based on ISS technologies that include a mass spectrometer for constituent analysis. Recent research and development of GC/MS technology has produced a miniature, space-rated version that performs well and is very lightweight. Mass was the major driver that caused the mini-GC/MS to be chosen for the monitoring technology.</p><p>Pressure control technology consists of pressure monitoring sensors and valve systems that can adjust the overall cabin pressure and partial pressures of major constituents. This technology is quite developed and there is little variation in methods from one system to another. A system similar to the ISS pressure control system was chosen for this life support system. See Appendix B for details on the technology. 5.5.6 Fire Detection & Suppression Technologies for smoke and fire detection include: scattering smoke detectors, ionization smoke detectors, condensation nuclei counters, obscuration smoke detectors, and UV/IR flame detectors. Scattering and obscuration smoke detectors are quite simple technologies that work well. Condensation nuclei counters and ionization smoke detectors are somewhat more complex and bit heavier than their counterparts. UV/IR flame detectors function to supplement the smoke detectors in warning the crew of an on- board fire. A system of scattering smoke detectors in UV/IR flame detectors was chosen for the life support system based on heritage use in the ISS.</p><p>Fire suppression will be handled in the life support system via the use of Portable Fire Extinguishers (PFEs). PFEs are small, pressurized tanks that can be operated by the crew to put out a cabin fire. They can be filled with CO2, N2, or Halon. Since Halon is toxic in large concentrations, it was not chosen as the fire suppressant. CO2 was chosen over N2 for fire suppression since there is an effective way to remove the excess CO2 from the environment via the CO2 removal system after the fire is put out. For more details on the technologies, see Appendix B. 5.5.7 Results The AM subsystem trade process led to the selection of several interacting technologies to fulfill the necessary functions of atmosphere management. The chosen technologies and their interactions are depicted in Figure 5-6 and Figure 5-8. The figures represent </p><p>Earth Replications, Inc. Page 16 ASEN5116 Final Report Spacecraft Life Support Systems two different configurations and operating schemes: one for the Earth-Mars transit phase, and one for the Mars surface stay.</p><p>ATMOSPHERE MANAGEMENT Pressure Control N2</p><p>CO2 C SOE CO To WP 2 2 BMS G G a a C s s O Low CO2 Air</p><p>2 S S r C C i e e c</p><p>O HEPA O h p p TCCS 2 2 A a , a , Filter</p><p>H H i r r r 2 a 2 a , , N</p><p>Humidity t t N N</p><p>H GC/MS</p><p> o FDS o H H 3 Control r , r</p><p>3 3 N , ,</p><p>O C C x H H ,</p><p>Air 4 S 4 , , O</p><p>N N x O O x x , ,</p><p>S S HUMAN O O O 2 H2, NH3, O2 x x CH4, NOx, SOx x CO2</p><p>From WP To WP Vent</p><p>Figure 5-6 AM Subsystem Diagram Transit</p><p>Figure 5-6 shows the configuration of the AM subsystem for the Earth-Mars transit phase. During this phase, the AM subsystem utilizes a 2-bed molecular sieve system for removal of CO2 from the cabin air. The removed CO2 is processed by the solid-oxide electrolysis system, which separates the CO2 into C and O2, sending the C to the WP subsystem and the O2 into use in the cabin air. Additionally, the cabin air is circulated via the ventilation system through HEPA filters and is processed by the Trace Contaminant Control System (TCCS). The gas chromatograph/mass spectrometer sensors insure that the major and minor constituents of the atmosphere are within acceptable limits. Additionally, the temperature and humidity of the cabin air is kept within requirements by the condensing heat exchanger and associated equipment. Overall pressure control is monitored and adjusted by valve systems to the storage containers for N2, O2, etc. The entire crew cabin area is monitored by scattering smoke detectors for early detection of fires. In case of a fire, throughout the cabin are CO2 portable fire extinguishers and portable breathing apparatus. Finally, exhaust gasses from the WP subsystem are routed to the AM subsystem where they are separated into those substances usable by the AM subsystem (i.e.CO2) and those that are currently not (e.g. NH3, CH4, etc.). Those that are not usable by AM may be temporarily stored or vented immediately. Care is taken in the AM subsystem to insure that there is no danger of the unusable gases from the WP subsystem contaminating the crew cabin. Figure 5-7 shows the mass, power, volume, etc. of the chosen components for the AM subsystem in the transit configuration. </p><p>Earth Replications, Inc. Page 17 ASEN5116 Final Report Spacecraft Life Support Systems</p><p>Add. Total Total Total Crew Weight Weight Weight Power Power Volume Volume Time Component # (kg) (kg) (kg) (kW) (kw) (m3) (m3) (hrs/day) Portable Fire Extinguisher (PFE) 3 15.10 0.00 45.30 0.00 0.00 0.04 0.12 0.00 Scattering Smoke Detector 8 1.50 0.00 12.00 0.00 0.02 0.04 0.32 0.00 Portable Breathing Apparatus 12 2.80 0.00 33.60 0.00 0.00 0.31 3.72 0.00 UV/IR Flame Detector 3 3.70 0.00 11.10 0.10 0.30 0.00 0.01 0.00 Pressure Control 1 74.00 0.00 74.00 0.10 0.10 0.41 0.41 0.00 Pressure Control Sensors 2 2.00 0.00 4.00 0.01 0.01 0.00 0.03 0.00 Gas Chromatograph/Mass Spectrometer (mini) 3 1.80 0.00 5.40 0.02 0.05 0.03 0.09 0.00 Trace Contaminant Control Subassembly (TCCS) 1 78.20 163.00 241.20 0.18 0.18 0.50 0.50 0.01 HEPA Filters 15 2.00 260.00 290.00 0.00 0.00 0.10 1.50 0.01 Common Cabin Air Assembly 2 112.00 0.00 224.00 0.47 0.94 0.40 0.80 0.00 Intermodule Ventilation Fan 2 4.76 0.00 9.52 0.55 1.10 0.01 0.02 0.00 Intermodule Ventilation Valve 12 5.10 0.00 61.20 0.01 0.07 0.01 0.12 0.00 Cabin Fan Assembly 1 26.90 0.00 26.90 0.41 0.41 0.15 0.15 0.00 2 Bed Molecular Sieve 2 96.00 28.80 220.80 0.50 0.50 0.60 1.20 0.00 Solide Oxide Electrolysis 2 220.00 44.00 484.00 6.50 6.50 0.80 1.60 0.00 Oxygen Buffer Tank 1 100.00 34.00 134.00 0.00 0.00 0.45 0.45 0.00 Nitrogen Buffer Tank 1 26.00 18.90 44.90 0.00 0.00 0.25 0.25 0.00 Totals 1921.92 10.16 11.28 0.02 Figure 5-7 AM Subsystem Parameters</p><p>ATMOSPHERE MANAGEMENT Pressure Control CH 4 N2 CH4 N2 CO2 , H2 Mars Sabatier Atmosphere CO2 TSA G G a a C s s O Low CO2 Air</p><p>2 S S r C C i e e c O O HEPA p h p TCCS 2 2 a a A , , Filter</p><p>H H H i r r 2 r a a 2 2 H2O , , N t</p><p> t Humidity N N H o o GC/MS FDS H H 3 Control r r ,</p><p>3 3 N , ,</p><p>O C C x H H ,</p><p>Air 4 4 S , , O</p><p>N N x O O x x , ,</p><p>SPWE S S HUMAN O2 O O O2 x x NH3, NOx SO, CO2 ISRU Plant</p><p>From Pyrolysis To Pyrolysis Vent</p><p>Figure 5-8 AM Subsystem Diagram - Mars Surface Figure 5-8 shows the AM subsystem as it is configured for use on the Mars surface. The major difference is the use of equipment from the ISRU plant that has been pre-landed on the surface via the cargolander. The equipment used from the ISRU plant consists of a Sabatier reactor and a solid polymer water electrolysis system. These in conjunction with a temperature swing absorption system perform the O2 and CO2 revitalization while on the surface. The temperature swing absorption system functions through utilization of the changes in temperature on the surface of Mars during day-night cycle. It removes the CO2 from the cabin air and outputs it to the Sabatier reactor. The Sabatier reactor produces CH4 for use as a propellant and H2O, which is sent to the solid polymer water electrolysis system. This system produces H2 and O2, the former being fed back to the </p><p>Earth Replications, Inc. Page 18 ASEN5116 Final Report Spacecraft Life Support Systems</p><p>Sabatier reactor and the later being used in the crew cabin air. The remainder of the AM subsystem performs the same on the Mars surface as it does in Earth-Mars transit. 5.5.8 Conclusions and Recommendations The result of the design and trade study process for the AM subsystem is a result of both the internal trade study process as well as system integration synergies with other subsystems and the ISRU plant on the Mars surface. The technologies chosen are those that best fit within the mission parameters of the Mars Design Reference Mission. The AM subsystem could benefit from further research into a few technologies: Advanced Carbon Formation Reactor (ACFR), Water Vapor Electrolysis (WVE), and Ammonia Dissociation. Specifically, Ammonia Dissociation would allow more loop-closure for N2 and H2, thus assuring less need to take replacement supplies on-board.</p><p>5.6 Atmosphere Management Subsystem References</p><p>1. Benkmann, C., Hoellriegelskreuth, "CO2 Removal by Pressure Swing Adsorption for Iron Direct Reduction Plants", Linde AG Reports on Science and Technology, ACHEMA 2000 Special Issue No. 62 2000 ISSN 0942-5268. 2. Chutjian, A., et al, "A Miniature Quadrupole Mass Spectrometer Array and GC For Space Flight: Astronaut EVA and Cabin-Air Monitoring", Warrendale, PA: Society for Automotive Engineers, 2000. International Conference on Environmental Systems, Paper No. 2000-01-2300. 3. Clemens, T., Haines, M., Heidug, W., "Optimized CO2 Avoidance Through Integration of Enhanced Oil and Gas Recovery with Solid Oxide Fuel Cells", F3-3 (2002) SPE 77348, Annual SPE Conference. 4. Common Cabin Air Assembly Description, http://www.hsssi.com/Applications/SpaceHabitat/CCAA.html. 5. Finn, J.E., “Solid State Compressor for Space Station Oxygen Recovery”, Overview in 2000 Annual Report, Astrobiology Technology Branch, Space Sciences Division. 6. Finn, J.E., Sridhar, K.R., McKay, C.P., “Utilization of Martian Atmosphere Constituents by Temperature-Swing Adsorption”. 7. Hanford, A. J. and Drysdale, A. E. (1999) “Advanced Life Support Technology Research and Development Metric – Initial Draft,” LMSMSS33045, Lockheed Martin Space Mission Systems and Services, Houston, Texas, 13 January 1999. 8. Horneck, G., DLR, "Advanced Life Support Systems and Human Exploratory Missions", 2nd Workshop on Advanced Life Support, ESTEC, 17-18 April 2002. 9. http://www.aist.go.jp/aist_e/new_research/20011206_1/20011206_1.html, "Water Split into Hydrogen and Oxygen Using Visible Light". 10. http://www.carbotech.de/AN/e/technologie3.htm, Modular Pressure Swing Adsorption (MPSA). 11. http://www.carbotech.de/AN/e/technologie3.htm, Vacuum Pressure Swing Adsorption (VPSA). 12. Human Spaceflight: Mission Analysis and Design, Larson & Pranke, 2000. 13. In-House Life Support Technology Review Databook, FY 91, Volume 5, NASA Ames Research Center, Advanced Life Support Division, Doc. No. 90-SAS-R-003, Rev.2, 1991 (http://joni.arc.nasa.gov/Database/index.shtml).</p><p>Earth Replications, Inc. Page 19 ASEN5116 Final Report Spacecraft Life Support Systems</p><p>14. International Space Station Cabin Fan Assembly Description, http://www.hsssi.com/Applications/SpaceHabitat/CFA.html. 15. Lygre, A., Matteo Cé, Vik, A., Byrknes, J., "Solid Oxide Fuel Cell Power Plants with Integrated CO2 Capture", 2nd Nordic Minisymposium on Carbon Dioxide Capture and Storage, Göteborg, October 26, 2001. 16. Marthe, P., et al, Crew Transfer/Return Vehicle Study Phase 3 Report, University of Alabama, April 2000 (http://www.eb.uah.edu/ipt/files/2000/IPT2000_Phase3_Final_Report_Team1.pdf) 17. Section 2.2.3.3, 2.3.3.1, 3.2.3.3, 3.3, 3.3.3.1, & 3.4, International Space Station Environmental Control & Life Support System Training Manual, TD9706. 18. Section 3, Shuttle Environmental Control & Life Support System Training Manual, TD415B. 19. Solid Waste Processing and Resource Recovery Workshop Report – Volume I, NASA Johnson Space Center, Crew & Thermal Systems Division, Doc. No. CTSD- ADV-474, Rev. A, 2002. 20. Spacecraft Life Support and Biospherics, Eckart, 1996. 21. Sridhar, K.R., Gottman, M., Baird, R.S., “AIAA 99-2413 2001 Mars In-Situ Oxygen Production Flight Demonstration”, 35th AIAA/ASME/SAE/ASEE Joint Propulsion Conference and Exhibit, Los Angeles, CA, June 1999. 22. Sridhar, K.R., Gottman, M., Baird, R.S., “AIAA 2000-1068 “Update on the Oxygen Generator System for the 2001 Mars Surveyor Mission”, 38th Aerospace Sciences Meeting and Exhibit, Reno, NV, January 1999. 23. Sridhar, K.R, Vaniman, B.T., "Oxygen Production on Mars Using Solid Oxide Electrolysis", SAE Technical Paper Series 951737, 25th International Conference on Environmental Systems, San Diego, California, USA July 10-13, 1995. 24. STS Humidity Control Heat Exchanger Assembly Description, http://www.hsssi.com/Applications/SpaceVehicles/multipurphx.html. 25. VanderWiel, D.P., Zilka-Marco, J.L., Wang Y., Tonkovich, A.Y., Wegeng, R.S., "Carbon Dioxide Conversions in Microreactors", Pacific Northwest National Laboratory, P.O. Box 999, MSIN K8-93, Richland, WA 99352. 26. Whitiker, Alana; Overview of ISS US Fire Detection and Suppression Subsystem; June, 2001.</p><p>Earth Replications, Inc. Page 20 ASEN5116 Final Report Spacecraft Life Support Systems</p><p>6.0 WATER MANAGEMENT 6.1 Water Management The requirements for water management are defined in Table 6-5. Table 6-5. Water Quality Requirement: Maximum Contaminant Levels Quality Parameters Potable Hygiene Physical Parameter Total Solids (mg/l) 100 500 Color, True (Pt/Co units) 15 15 Taste (TTN) 3 N/A Odor (TON) 3 3 Particulates (max size - microns) 40 40 pH 6.0-8.5 5.0-8.5 Turbidity (NTU) 1 1 Dissolved Gas (free @ 37 C No Detectable Gas N/A Free Gas (@ STP) No Detectable Gas No Detectable Gas Inorganic Constituents (mg/l) Ammonia 0.5 0.5 Arsenic 0.01 0.01 Barium 1.0 1.0 Cadmium 0.005 0.005 Calcium 30 30 Chlorine (total - include chloride) 200 200 Chromium 0.05 0.05 Copper 1.0 1.0 Iodine (total - include organic iodine) 15 15 Iron 0.3 0.3 Lead 0.05 0.05 Magnesium 50 50 Manganese 0.05 0.05 Mercury 0.002 0.002 Nickel 0.05 0.05</p><p>Nitrate (NO3-N) 10 10 Potassium 340 340 Selenium 0.01 0.01 Silver 0.05 0.05 Sulfate 250 250 Sulfide 0.05 0.05 Zinc 5.0 5.0 Bactericide (mg/l) Residual Iodine (minimum) 0.5 0.5 Residual Iodine (maximum) 4.0 6.0 Aesthetics (mg/l) Cations 30 N/A Anions 30 N/A</p><p>CO2 15 N/A Micobial Bacteria (CFU/100ml) Total Count 1 1 Anaerobes 1 1</p><p>Earth Replications, Inc. Page 21 ASEN5116 Final Report Spacecraft Life Support Systems</p><p>Quality Parameters Potable Hygiene Coliform 1 1 Virus (PFU/100 ml) 1 1 Yeast & Mold (CFU/ 100ml) 1 1 Organic Parameters (ug/l) Total Acids 500 500 Cyanide 200 200 Halogenated Hydrocarbons 10 10 Total Phenols 1 1 Total Alcohols 500 500 Total Organic Carbon (TOC) 500 10,000 Uncharacterized TOC (UTOC) 100 1,000</p><p>6.2 Water Monitoring Water Monitoring is a major subsystem within the water management. Therefore, any technology that has the Technology Readiness Level (TRL) of less than 7 is assumed to be unacceptable for the Life Support System (LSS) and not studied in this section. The TRL of 7 means that the system prototype has been demonstrated in space environment. However, if the technology is not gravity dependent, the acceptable TRL is said to be 5. At this TRL level, the component and/or the breadboard have been validated in relevant environment and shows promising evidences that it would also work in space. With this in mind, the first tread study would be to do the simple pass/fail elimination due to the TRL level. Table 6-6. List of Technologies & Associated TRLs Technologies TRL</p><p>Fiber Optic Sensor for Water11 (for turbidity, color, pH, iodine, metals, ions, NOC, VOC, semi- 4 volatiles and hardness level)</p><p>Electronic Nose11 (for taste, metals, NOC and odor) 6</p><p>Ion Mobility Spectrometry (IMS)11 (monitor iodine level) 2-4</p><p>Ion Specific Electrodes (ISE)11 (for conductivity level) 2-8</p><p>Capillary Electrophoresis (CE)11 (for metals level) 3-4</p><p>Liquid Chromatography (LC)11 (for metals, ions level) 2-3</p><p>Ion Coupled Plasma (ICP)11 (for metals, ions and silver) 3</p><p>Solid Phase Extraction-Mass Spectrometry (SPE-MS)11 (for NOC, VOC and semi-volatiles) 2-4</p><p>Supercritical Fluid Chromatography (SFC)11 (for NOC, VOC and semi-volatiles) 1-2</p><p>Liquid Chromatography-Mass Spectrometry (LC-MS)11 (for NOC, VOC and semi-volatiles) 3</p><p>Fourier Transform Infrared (FTIR)11 (for NOC, VOC and semi-volatiles) 4</p><p>Total Organic Carbon-Infrared (TOC-IR)11 (for TOC/COD) 4</p><p>Total Organic Carbon (TOC) - conductivity11 6</p><p>Total Organic Carbon (TOC) - reagentless11 2</p><p>Total Organic Carbon Analyzer14 3</p><p>Gas Chromatography (GC)11 (for NOC and semi-colatiles) 4</p><p>Voltametry11 (for metals and silver) 3</p><p>UV-visible Spectrophotometer (laser)11 (for turbidity, color, pH, iodine, metals, ions, NOC, 2 VOC, semi-volatiles and hardness level) </p><p>Surface Acoustic Wave (SAW) Detector11 4</p><p>Test Kits11 (for pH, chem-strips for specific compounds) 9</p><p>Conductivity11 8-9</p><p>Test Kits11 (for pH, chem-strips for specific compounds) 5-9</p><p>Earth Replications, Inc. Page 22 ASEN5116 Final Report Spacecraft Life Support Systems</p><p>Therefore the technologies that will be studied are the following:</p><p>1. Electronic Nose11 (for taste, metals, NOC and odor) [TRL 6]11</p><p>Figure 6-1. Electronic Nose Equipment</p><p>2. Ion Specific Electrodes (ISE)11 (for conductivity level) [TRL 2-8]11</p><p>Figure 6-2. Ion Specific Electrode</p><p>3. Total Organic Carbon (TOC) - conductivity11 [TRL 6]11</p><p>Earth Replications, Inc. Page 23 ASEN5116 Final Report Spacecraft Life Support Systems</p><p>Figure 6-3. Total Organic Carbon - Conductivity Diagram</p><p>Figure 6-4. Actual Total Organic Carbon device</p><p>4. Conductivity11 [TRL 8-9]11</p><p>Figure 6-5. Sample of Conductivity device</p><p>5. Test Kits11 (for pH, chem-strips for specific compounds) [TRL 5-9]11</p><p>Table 6-7.Water Sample Requirement for Off-Line MonitoringFigure 6(initial-6. Sample On-Orbit Test Kits Operations)devices Location Sample Volume Frequency</p><p>Earth Replications, Inc. Page 24 ASEN5116 Final Report Spacecraft Life Support Systems</p><p>ECLSS Storage Tank 500 ml/day Every day Random (Tank or Use Port) 500 ml/day Every 2 days Avg. Total Volume 5,250 ml/week Avg. No. Sample 10.5 times/week</p><p>Table 6-8.Water Sample Requirement for Off-Line Monitoring (Mature Operations)6 Location Sample Volume Frequency ECLSS Storage Tank 110 ml/day 6 days/week 500 ml/day 1 days/week Random (Tank or Use Port) 110 ml/day 3 days/week 500 ml/days 1 days/week Avg. Total Volume 1,990 ml/week Avg. No. Sample 11.0 times/week</p><p>6.2.1 Flow Chart for Water Monitoring Since each technology can detect different contaminates in water, the trade study cannot be conducted to compare them to one another. The combination of all the technologies is considered to be the best arrangement. The repetition of the monitoring follows for the redundancy of the subsystem. </p><p>ISE Conductivity pH, Iodine, TOC/COD, hardness </p><p>TOC TOC/COD </p><p>Conductivity Gross quality indicator </p><p>Electronic Nose Odor, taste </p><p>Test Kits Conductivity, pH, Iodine, TOC/COD, Hardness </p><p>Figure 6-9The Order of Monitoring Devices 6.3 Water Generation Water Generation unit is an excellent system to enclose on Mars LSS since not 100% of water can be reclaimed in the total system even on the high efficiency technology. This indicates that the crews are constantly losing water on the LSS. All the technologies in </p><p>Earth Replications, Inc. Page 25 ASEN5116 Final Report Spacecraft Life Support Systems water generation are in the conceptual level. Even though the crews should not depend on the water generation using in-situ resources, the possibility of the technologies to be fully developed by the time human exploration on Mars exists is highly achievable. The water generation until is a back up subsystem, which is included mainly for the purpose of experimentation. This is an excellent time to test the technology that is necessary in the future. It was not meant to be a main subsystem and will not have a major effect on the optimum design of the LSS. Please note that the buffer size of water on the LSS is sufficient for the crews without this water generation unit. The list of water generation subsystem is as follow. </p><p> Zirconia Electrolysis Cell Unit6 [TRL 1-2]</p><p> Water Vapor electrolysis11 [TRL 4]</p><p> Cyanobacteria11 [TRL 6]</p><p> Sabatier Reaction7,8,9 [TRL 6]</p><p>Not all of the technologies have TRL associated with them. Therefore, there is not much information on each technology other than the formula that has CO2 as one of the input and H2O as one of the output. There is no reason to omit any technology until future study has been done and more data is available. Therefore a trade study is not done in this situation. The formulae for all the technologies are as shown.</p><p>1. Zirconia Electrolysis Cell Unit: Operate at 800 to 1000 C</p><p>2 CO2 2 CO + O2</p><p>O2 +2H2 2 H2O</p><p>2. Water Vapor Electrolysis: has TRL up to 4 Also use Zirconia Electrolysis Cell Unit</p><p>3. Cyanobacteria: has TRL up to 6 Depends on biological and might be gravity dependent </p><p>4. Sabatier Reaction: TRL up to 6</p><p>4 H2 + CO2 CH4 + 2 H2O</p><p>Since Sabatier reaction is already being used in the Atmosphere Management Subsystem on the surface of Mars, this technology made the most sense to be used for water generation subsystem as well. This selection is proven to reduce the mass of the whole system. </p><p>6.4 Water Processing 6.4.1 Fire Detection & Suppression</p><p>Earth Replications, Inc. Page 26 ASEN5116 Final Report Spacecraft Life Support Systems</p><p>The baseline water management requirements are to provide potable and hygiene water to the crew for the duration of the mission. As outlined in the human mass balance section, 3.905 kg/CM/day of potable water and 23.65 kg/CM/day of hygiene water must be provided and meet the water quality requirements outlined in section 1.0. Detailed requirements for the LSS can be found in Appendix A.</p><p>6.4.2 Baseline Architecture</p><p>Waste Management </p><p>2.5 Laundry </p><p>Huma 9.4 Hygiene Storage n 18.216 Potable </p><p>0.214 </p><p>Food Management </p><p>. </p><p>Figure 6-10 Open Loop Diagram w/ Flow Rates in kg/day for 6 Crewmembers</p><p>Table 6-9 WM Parameters Mass Power Volume (kg) (kW) (m3) 180 Days 29759.4 29.8 500 Days 82665.0 82.7 Total 112424.4 112.4 Water Storage 22484.9 0.02 5.62 Total 134909.3 0.02 118.02</p><p>Figure 6-10 shows the open loop block diagram for the water management subsystem. The only components needed to operate the system include storage and delivery. A 10% increase in the stored water quantity is included to provide a safety buffer. The storage </p><p>Earth Replications, Inc. Page 27 ASEN5116 Final Report Spacecraft Life Support Systems</p><p> mass was assumed to be 20% of the stored mass. Table 6-9 includes a summary of the total subsystem mass for 180 day transit and 500 day Martian stay. This mass does not include any dependence on ISRU because it is unknown if the water production rates would match the 165.33 kg required per day. </p><p>6.4.3 Potable and Hygiene Water Processing Water processing technologies were broken down into two main categories, Potable and Hygiene processing and urine processing. This section discusses potable and hygiene water processing.</p><p>6.4.3.1 Candidate Technologies</p><p>Table 6-10 Hygiene & Potable Water Treatment Candidate Technologies WM Function Candidate Technologies</p><p>Hygiene & Potable Water MIR Technology (Condensate)10</p><p>Treatment Reverse Osmosis (RO)1,13</p><p> Multifiltration (MF)1,13</p><p> Electrodialysis1</p><p> Oil and Water Seperation2</p><p> Rock/Plant/Microbial Filtering System3,4 </p><p> Thermoelectric Integrated Membrane Evaporation (TIMES)1</p><p> Granular Activated Carbon (GAC)5</p><p> Aqueous Phase Catalytic Oxidation Subsystem (APCOS)</p><p> Ultrafiltrtion10</p><p> MilliQ Absorbtion Beds</p><p> Pasteurization</p><p> Ionic Silver1</p><p> Regenerable Microbial Check Valve (Iodine)12,15</p><p> UV-visible Spectrophotometer (laser)11 </p><p>6.4.3.2 Infeasible Technologies Due to the significant list of candidate technologies, a series of selection criteria needed to be used to rule out undeveloped technologies. TRL levels less than 6 and complete lack of information were the primary criteria used to reduce the number of candidate technologies. Further elimination of technologies was performed during the formation of the spec sheets due to key information (such as mass or power) missing.</p><p>6.4.3.3 Technology Trade Study The potable and hygiene water processing consisted of numerous technologies, each consisting of unique pre and post treatment processes. This required the development of </p><p>Earth Replications, Inc. Page 28 ASEN5116 Final Report Spacecraft Life Support Systems</p><p> systems of different technologies to be traded between. Hygiene and potable water will be processed with the same system. Consumable data is primarily described for complete processing to potable quality water, therefore the data is not available for a unique hygiene water processing trade. The following systems were traded:</p><p> o UltraFiltration/Reverse Osmosis + APCOS o UltraFiltration/Reverse Osmosis + MilliQ Absorbtion Beds o Multifiltration</p><p>The UF/RO and MilliQ Absorption Beds won the trade. Multifiltrations downside was its large consumable mass while the APCOS was significantly pentalized due to the unknown oxygen consumption for the oxidation process. </p><p>Microbial control was separated from this trade study due to its need in any system chosen. Due to lack of information, the Iodine Microbial Check Valve was chosen. Iodine removal beds are also required before potable use to eliminate long-term effects of Iodine consumption. Detailed trade sheets can be found in Appendix B.</p><p>6.4.4 Urine Processing 6.4.4.1 Candidate Technologies</p><p>Table 1-7. Urine Treatment Candidate Technologies. Water Management Function Candidate Technologies</p><p>Urine Treatment MIR Technology (evaporation, steam condensation, sorption, </p><p> electrolysis)10</p><p> Vapor Compression Distillation (VCD)1,13</p><p> Vapor Phase CatalyticAmmonia Removal (VAPCAR)1</p><p> Air Evaporation (AES)1</p><p> Aqueous Phase Catalytic Oxidation Post-Treatment System (APCOS)10,13</p><p> Super Critical Water or Wet Oxidation (SCWO)1</p><p> Incineration (oxidation)11 </p><p> Pyrolysis11</p><p> Aerobic Slurry11</p><p> Aerobic Solid Processing (composting)11 </p><p> Anerobic Solid Processing11</p><p> Aquaculture (fish)11 </p><p> Electrochemical Oxidation11 </p><p>6.4.4.2 Infeasible Technologies Again this large list of technologies was reduced through a few selection criteria. The primary selection factor in urine treatment was the elimination of biological systems following the DRM requirements. TRL levels below 6 and lack of information rules out many of the other candidates.</p><p>Earth Replications, Inc. Page 29 ASEN5116 Final Report Spacecraft Life Support Systems</p><p>6.4.4.3 Technology Trade Study Due to the severe elimination of technologies by initial selection criteria, only two were left. The urine distillation trade was performed between the following technologies:</p><p>-Air Evaporation System (AES) -Vapor Compression Distillation (VCD)</p><p>Vapor Compression Distillation won the trade study with the Air Evaporation System in second. The primary reason the AES places second was due to the high power consumption of 1 kW. This is approximately 10% of the allotted power for the spacecraft dedicated to one system. A simple trade between carrying makeup water in the VCD only system versus using an AES to process the brine water on a low duty cycle resulted in a significant mass savings of approximately 175 kg as well as providing a very simple redundant system in the case of a VCD failure.</p><p>6.4.5 Closed Loop Architecture</p><p>Pretreatment Urine HUMAN Urine Ozone Pretreated VCD AES Sulfuric Acid Urine B B r Hygiene H O r i 2 i n n e Storage Tank e Brine </p><p>H H H O 2 2 2 O O Ultra Filtration Iodine Removal Bed RO</p><p>ISE Monitoring Iodine testing Monitoring MCV Milli Q Iodine Waste Water (Hygiene, AM, WP)</p><p>Potable Input H2O Water Management</p><p>Figure 6-11 Closed Loop Water Management Flow Diagram</p><p>Figure 6-11 shows the integration of the water management subsystem components. The urine treated by the VCD must be pretreated with Ozone (a commercial oxidizer) and sulfuric acid to prevent the release of ammonia during the distillation process. Brine water from the reverse osmosis filter is also passed through the VCD to reclaim more water. The water flow through the VCD can be arranged in numerous forms and is not </p><p>Earth Replications, Inc. Page 30 ASEN5116 Final Report Spacecraft Life Support Systems completely shown on this diagram. Brine water from the VCD can be passed back through itself to maximize the solid concentration of the brine. The remaining brine water is stored and periodically processed by the AES. Current AES testing shows a slightly reduced quality of water from the AES, so this water is reprocessed through the VCD to ensure complete processing of the urine. </p><p>Product water from the VCD is combined with the remaining wastewater from the craft including that from hygiene, condensate and Pyrolysis recovery. The water stream then flows through an Ultra filtration unit which consists of mechanical filtration media. This increases the lifetime and efficiency of the following reverse osmosis filter. As stated earlier, the brine water produced in processed through the VCD. The product water then flows through the Milli-Q absorption bed which consists of activated carbon and a proprietary organic carbon scavenger media to reduce the TOC to acceptable potable water quality standards. At this time, Iodine is then added to the water stream for microbial control. Monitoring then checks the PH, conductivity, TOC and Iodine levels before the water is stored. Hygiene water is used directly from this source. Potable water must first be passed through the iodine removal bed to reduce the iodine level to acceptable amounts. Online iodine monitoring then ensures this level. </p><p>The water buffer capacity of this system is 324.28 kg. This was calculated for the transit trip only due to the ISRU having the capacity to provide the small amounts of makeup water required. The Earth Return Vehicle would require a larger storage capacity to provide for the potential on orbit stay in addition to the return trip. The only water loss within the closed water system takes place in the Pyrolysis waste processing unit used to process feces. This 90% efficient system creates a loss of 10.8 kg of water during transit. With the near 100% design efficiency of the system, and no additional information on losses or buffer size, a value of 1% of the total processed water for transit was used in addition to the expected loss of 10.8 kg from Pyrolysis. Adding in our 10% safety factor of consumables results in 324.28 kg. In an event of total water system failure, this allows for 14 days under normal potable water use without any hygiene use. This should be plenty of time to allow for repairs of the system. Table 6-11 Water Management Subsystem Mass Breakdown Mass (kg) Hardware 588.212 Water Buffer 324.28 180 Day Consumables 54.5 500 Day Consumables 142.2 Total 1109.192</p><p>Table 6-11 shows the breakdown of mass between consumables, buffer and hardware. The total mass includes all consumables for the transit/habitation module, while depending on ISRU for water makeup on the surface. If the cargo lander brought the consumables for the surface stay, the mass would be reduced to 966.99 kg. The Earth Return vehicle water subsystem mass would be larger due to the increased buffer size for the potentially long, on orbit stay.</p><p>Earth Replications, Inc. Page 31 ASEN5116 Final Report Spacecraft Life Support Systems</p><p>6.5 Conclusions and Recommendations Current technology provides a substantial choice of operational subsystems within water management. Detailed testing has been performed on several occasions and provides excellent information on physical/chemical and biological systems. Many promising technologies in development or in use have been developed in the private sector and almost all information is proprietary. While these technologies could provide the optimum solution to our design requirements, they had to be eliminated or severely penalized within the trade study due to the lack of detailed information.</p><p>Buffer size is one subject that is not discussed within literature. Only one reference was found which mentioned buffer size. This is a very critical design feature that will significantly affect the mass and safety of the system. Further research or testing is needed on this subject. 6.6 Water Management Subsystem References 1. Spacecraft Life Support and Biospherics, Eckart, 1996. 2. http://techlink.msu.montana.edu/articles/spacedwellers.html, "Water for Space Dwellers" 3. http://techtran.msfc.nasa.gov/Patents/(21).html, "Combined Air and Water Pollution Control System" 4. http://science.nasa.gov/headlines/y2001/ast09apr_1.htm, "Leafy Green Astronauts" 5. http://www.admin.mtu.edu/urel/breaking/2000/water.html, "MTU Engineering Earn Landmark Award for Water Treatment Design Approach" 6. http://www.lpi.usra.edu/meetings/isru97/PDF/MINH.PDF, " Production of Oxygen Carbon Dioxide Using Zirconia Electrolysis Cells" 7. http://www.sff.net/people/Geoffrey.Landis/propellant.html, "Making Rocket Propellant on Mars" 8. http://www.isso.uh.edu/publications/A9900/html/mini/mini-richardson.htm, "Improving Sabatier Reactions for In-Situ Resource Utilization on Mars Missions" 9. http://perso.wanadoo.fr/salotti/Zubrincarburant.doc, "Report on the Construction and Operation of a Mars In-Situ Propellant Production Unit" 10. http://www.colorado.edu/ASEN/asen5116/Lect-16.htm, "Lecture 16" 11. http://research.hq.nasa.gov/code_u/matrix/index, "Technology Readiness Level (TRL) and Equivalent System Mass (ESM)" 12. http://lsda.jsc.nasa.gov/readingroom/4.2WaterChem.pdf, “Water Chemistry Monitoring” 13. http://advlifesupport.jsc.nasa.gov/ehti2/bkgrd.html, “Lunar-Mars Life Support Test Project Phase II” 14. http://lsda.jsc.nasa.gov/scripts/cf/hardw.cfm?hardware_id=827&string=TOC, “Total Organic Carbon (TOC) Analyzer” 15. http://www.urc.cc/rmcv.htm, “URC: Regenerable Microbial Check Valve” 16. Advanced Life Support Program: Requirements Definition and Design Considerations JSC 38571, National Aeronautics and Space Administration 1998</p><p>Earth Replications, Inc. Page 32 ASEN5116 Final Report Spacecraft Life Support Systems</p><p>7.0 WASTE MANAGEMENT 7.1 Baseline Functional Requirements The waste model for the WP Subsystem is based on the data found in the Baseline Values and Assumptions Document (Hanford et al. 2002) for a six-person crew. The functions of the subsystem include collecting, processing, storing and/or dumping waste, when necessary. Table 7-12 presents a summary of all the different sources of waste within the life support system. Packaging material constitutes the largest waste mass. Even though this life support system does not depend on any bioregenerative sources, inedible plant biomass is taken into account in the mass budget to account for this future possibility. Table 7-12. Waste Model for a Six-Person Crew (Verostko et al. 2002, Handford et al. 2002) Subsystem or Interface Waste Component Weight (kg/day) WP, WM Dry Human Waste: feces, urine, 0.720 shower/hand wash and sweat. FS Inedible Plant Biomass 1.691 AM, WM Filters 0.326 Trash: clothes/towels, pads/tampons, 0.556 menstrual solids and paper. Packaging Material: snack packaging, food containers, plastic bags, food 7.908 In General remains, frozen, refrigerated, ambient, beverage and straws. Paper: wipes, tissues and general waste. 1.164 Tape: masking, conduit and duct. 0.246 Miscellaneous: Teflon and PVC. 0.069 Total 12.680</p><p>7.2 Baseline Architecture The WP open-loop mass flow diagram is shown as Figure 7-12. This diagram illustrates the baseline architecture for the system at its fundamental level with no waste processing. In this mode of operation, the only processes taking place are chemical stabilization of decomposable matter such as biological waste, and storage of materials. This mass will drastically decrease as the waste product output is incorporated as consumables into the input streams for the WM and AM subsystems.</p><p>Earth Replications, Inc. Page 33 ASEN5116 Final Report Spacecraft Life Support Systems</p><p>Waste Non-Biological 14.292</p><p>Biological Chemical Dump 168.393 Stabilization</p><p>All values are in kg/day Storage for 6 crew members Figure 7-12 WP Open Loop Diagram</p><p>7.3 Subsystem Assumptions The following assumptions have been made when designing the WP Subsystem: A collection system for human wastes such as urine and feces has already been designed (urinal and commode); therefore, no further development will be explored within this document. Urine and feces are assumed to be collected individually; therefore, there is no need for separation within the WP Subsystem. The Water Management Subsystem will treat urine effluent. The WP Subsystem will not rely on any biological treatment. No metals will be processed. The budget for developing and building technologies is not constrained.</p><p>7.4 Subsystem Technology Trade Studies The trade variables used to compare the technologies in the WP Subsystem is shown in Table 7-13. Out of all these variables, mass, TRLs, and the inherent process variables such as pressure, temperature and volume were given a higher weight in the trade matrix. Numerical values were assigned to qualities such as reliability and safety (1=low, 2=med, 3=high) to be able to account for those variables in the selection process. Table 7-13. Waste Processing Trade Variables</p><p>Selection Criteria Unit/Value Component Mass Kg Additional Mass Kg Power kW Volume m3 Operating Temperature K Operating Pressure kPa TRL 1-9 Reliability 1-3*</p><p>Earth Replications, Inc. Page 34 ASEN5116 Final Report Spacecraft Life Support Systems</p><p>Selection Criteria Unit/Value Maintainability 1-3* Gravity Dependence yes, no Safety 1-3* Prospective Improvements 1-3* *(low, med, high)</p><p>7.4.1 Candidate Technologies A wide variety of technologies were considered to establish the infrastructure of the WP Subsystem. Given the assumptions for the WP design, biological treatments were mentioned but not compared against physicochemical technologies, due to the increased challenges involved in maintaining plants and bacterial cultures alive. Physicochemical processes have the advantage of rapidly converting waste to products, most of which can be used to complete other functions within the system. The candidate technologies are characterized in – Table 7-16, mapped to the function that they help provide.</p><p>Earth Replications, Inc. Page 35 ASEN5116 Final Report Spacecraft Life Support Systems</p><p>Table 7-14. Preprocessing Technologies for Waste Management Waste Management Candidate Technologies (Refs. 2,6,7) Function Vacuum Waste Collection and Transport Bulk Compaction Dry Size Reduction and Particle Size Control Drying (Forced Air Thermal Convection, Forced Air, Collection and stabilization of Thermal Vacuum, and Freeze Vacuum) waste Pneumatic Transport of Dry Material Screw Conveyor Slurry Pumping</p><p> Solid/Liquid Blending; Slurrying (50-95% H2O) Solid/Solid Blending Storage Wet Size Reduction and Particle Size Control</p><p>Table 7-15. Biological Processing Technologies for Waste Management Waste Management Candidate Technologies (Refs. 2,5,6,7) Function Aerobic/Anaerobic Waste Processing Activated Sludge Composting Aerobic Completely Mixed (Slurry) Biological Reactor Plant Nutrient Extraction Variant – 7 Day Residence Time (No Curing Stage/Biofilter) Biological treatment of waste Plant Nutrient Extraction Variant – 21 Day Residence Time Fixed-Film Bioreactor High-Solids Leach Bed Anaerobic Digestion using SBAC (Sequential Batch Anaerobic Composting) Paper and Biomass to Products Single Cell Protein Production and Crop Nutrient Recovery</p><p>Earth Replications, Inc. Page 36 ASEN5116 Final Report Spacecraft Life Support Systems</p><p>Table 7-16. Physicochemical Technologies for Waste Management Waste Management Candidate Technologies (Refs. 1,2,3,6,7) Function Super Critical Wet Oxidation Wet Oxidation Batch Incineration Continuous Incineration Biologically decomposable Pyrolysis solids (feces, waste with Gasification bound water, solids from Electrochemical Oxidation urine, sweat and hygiene Molten Carbonate Oxidation water, clothes) Starved Air Combustion </p><p> Proteolysis</p><p> Acid Hydrolysis</p><p> Plasma Arc Oxidation</p><p> Peroxide Oxidation</p><p> Space Shuttle Waste Management System Waste Management – Water Systems </p><p> Batch Incineration</p><p> Continuous Incineration</p><p>Non decomposable solids Electrochemical Oxidation </p><p>(spare parts, plastic, metal, Molten Carbonate Oxidation filters) Super Critical Wet Oxidation</p><p> Dumping</p><p> Storage</p><p> Batch Incineration</p><p> Continuous Incineration</p><p>Non decomposable solids Plasma Arc Oxidation</p><p>(products from experiments, Pyrolysis medicine) Molten Carbonate Oxidation</p><p> Dumping</p><p> Storage</p><p>7.5 Results A total of seven (7) technologies were selected from all the initial candidates, using TRL, versatility and prospective potential as cut-offs. Versatility was determined by the number of functions and the capability of the technologies to process a variety of wastes. Prospective potential was determined by the number of years and cost for the technology to achieve a TRL of 5 (Verostko et al. 2002). The chosen technologies were: Electrochemical Oxidation, Gasification, Supercritical Water Oxidation, Incineration (Batch and Continuous), Plasma Arc Oxidation and Pyrolysis. All of these have a TRL of 3 or above, with the exception of Gasification (TRL 2), which was deemed worthy of consideration because of all of its advantages and potential for future development.</p><p>Earth Replications, Inc. Page 37 ASEN5116 Final Report Spacecraft Life Support Systems</p><p>A brief description of each candidate technology and a summary of their main traits follows in Table 7-17. Process diagrams as well as specification sheets can be found in Appendix B.</p><p>Table 7-17 Summary of Assessed Physicochemical Technologies Prospective Technology Advantages Disadvantages TRL Improvement Batch -Treats all wastes -Flue gas contaminants High 3 Incineration -High conversion -High T $ to TRL 5= $1M</p><p>Continuous -High TRL -Flue gas contaminants 5 Uncertain Incineration -High conversion -High T Electrochemica -Pretreatment required -Low T & P Medium l -Complex waste 4 -Treats all wastes $ to TRL 5= $5M Oxidation effluent -Minimum NOx, SO 2 -Low TRL High Gasification -Many useful 2 -High T $ to TRL 5= $600k - $1M products -Treats all wastes -High T & p Medium SCWO 4 -Clean products -Solids plugging $ to TRL 5= $1 - 5M</p><p>Plasma Arc -Treats combustibles -Doesn’t treat metals Medium 4 Oxidation -Fast reaction -High power & T $ to TRL 5= $8 - $13M -Treats many wastes -Doesn't treat metals High Pyrolysis -Many useful 3 -CO production $ to TRL 5= $500k products</p><p>7.5.1 Batch and Continuous Incineration (BI, CI)</p><p>Incineration is a process that is capable of converting almost all waste into CO2, H2O, and inorganic ash. Pretreatment is necessary to dry and prepare the waste for the feed system. Waste is heated at ambient pressure to 813 K using pure O2 as the oxidizing agent. The incineration process consists of a combustor and a contaminant clean-up system. Produced contaminants (CO, HCl, SOx, NOx, and trace hydrocarbons) are removed by catalytic systems and absorption beds. In the continuous mode the system is fed at a constant feed rate, holds a constant temperature and composition, and produces a continuous constant composition output. Under batch operation, a pack of material is fed at a specified interval of time. </p><p>7.5.2 Electrochemical Oxidation (EO) In this process, soluble organic materials can undergo oxidative degradation at a low temperature and pressure. This method does not produce NOx, SOx, or CO and it </p><p>Earth Replications, Inc. Page 38 ASEN5116 Final Report Spacecraft Life Support Systems requires less clean up than thermal systems. The breakdown occurs by the reaction that takes place at the interface between the anode and the electrolyte-waste solution. H2 is produced as a by-product at the cathode, which can be used to feed a fuel cell to produce electricity. This system can be easily scaled down to fit the waste requirements of a crew of 6 (Verostko et al. 2002). Some of the drawbacks that this process presents are that the feed needs to be pretreated and turned into slurry; some of the gaseous emissions include chlorine; and it consumes high amounts of acid and alkaline solutions.</p><p>7.5.3 Gasification (GAS) In the gasification process, any carbon-containing material can be converted into synthesis gas and energy, through the reaction between the feed and steam in the presence of O2 in a high temperature and pressure gasifier. The waste is fed in dry or in slurry form to the gasifier. Once in the gasifier this material reacts with steam and CO2 at a high temperature and temperature in an oxygen starved environment. This produces a synthesis or “syn” gas made up of mostly of CO and H2. The high temperature in the gasifier changes the inorganic material into stable “slag”. Post treatment processing refines the raw gases and collects trace contaminants. This system can convert nearly 100% of all waste into useful end products of gas and energy. Gasification has a current TRL of 2, but shows future potential.</p><p>7.5.4 Supercritical Water Oxidation (SCWO) SCWO uses water in its supercritical state to break down toxic organic compounds into harmless oxidation by-products such as carbon dioxide and water. It consumes oxygen and power at a high temperature and pressure and has an aqueous stream output that carries all the inorganic salt residues. It produces water and carbon dioxide that is clean enough to be reused without processing. Safety concerns exist because the system operates at a high temperature and pressure. Precautions such as venting, monitoring, pressure relief must be taken to assure proper reactions.</p><p>7.5.5 Pyrolysis (PYRO) From the Greek “puro” (fire, heat) and “lusis” (destruction), Pyrolysis is a process that breaks down waste by heating at high temperature in the absence of O2. It is able to function both in batch and continuous mode, and has the advantage of having a product- rich waste stream that can be used by other subsystems. This technology will produce a char residue that can be gasified, combusted or activated to be used as an adsorbent (Verostko et al. 2002). Other by-products of this process include H2O, CO2, H2, CH4 and NH3. 7.5.6 Plasma Arc Oxidation (PAO) PAO completely decomposes waste materials in an oxygen-starved, extremely high temperature environment. A plasma arc torch provides a plasma gas at 2000-3000 Celsius. This extreme heat and oxygen-starved environment results in Pyrolysis, or heat destruction. The output of this system is combustible gas and an inert slag, whose characteristics will vary, depending on the type of input waste. This process is extremely</p><p>Earth Replications, Inc. Page 39 ASEN5116 Final Report Spacecraft Life Support Systems efficient and creates a volume reduction of almost 250:1. This system has not been tested in a microgravity environment and concerns exist regarding heat transfer characteristics. Reducing the size of this system for a human spacecraft environment may be a problem.</p><p>7.5.7 Trade Study Process Once the technologies made the first cut, they were divided into two groups according to the type of waste they could process: technologies that could break down virtually all waste (EO, GAS, and SCWO), and technologies that could break down everything but metals (BI, CI, PAO, and PYRO). Gasification obtained the highest score in the ‘all waste’ category. This technology was included in the trade matrix for the ‘all waste/no metals’ category as well, since it was comparable to their functionality. Again, Gasification won within this category, leaving Pyrolysis as the runner-up technology.</p><p>The final step in the trade study was to contrast the strengths and weaknesses of those two remaining technologies and weight their functionality with respect to the other subsystems’ needs and capabilities. For comparison purposes, includes Plasma Arc Oxidation along with the two top technologies that resulted from the trade study. Even when the three technologies have similar traits, Pyrolysis has a richer product stream and less consumables than the other two processes. Plasma Arc Oxidation has the highest TRL [4], but is nearly 20 times heavier than the other two systems. Even when the prospects for future improvement are high for this technology, scale down is foreseen to be very difficult and the cost to TRL 5 is the highest of all the technologies (see Table 7- 17). There is a major contrast in the two top technologies processing capability. Gasification can treat a batch of 50 kg of waste in one hour’s worth of operation, while Pyrolysis can process about 0.5 kg/cycle (cycle = 0.5 – 2 hours), meaning that continuous operation would be required for the latter. While batch operation may seem desirable against continuous operation, this does not necessarily equate to less crew time because aspects such as maintenance come into play. Pyrolysis is has been tested extensively on Earth and has a higher TRL than Gasification, thus providing the advantage of years of troubleshooting. Given that the design for this mission encompasses not only transit to Mars, but an extended stay on the Martian surface as well, gravity dependence becomes a critical issue in deciding which technology is more suitable for all scenarios. The only system out of the entire list of candidate technologies that is not foreseen to have any issues with changing gravity conditions is Pyrolysis, making it the winning technology for this trade study.</p><p>Table 7-18. Comparison of Top Three Trade Study Candidate Technologies</p><p>Earth Replications, Inc. Page 40 ASEN5116 Final Report Spacecraft Life Support Systems</p><p>Technolog Processing y Products Weight (kg) Consumables Capability</p><p>Gasification CO2, CO, H2, Ash, Energy 65 kg 10 - 20 kg/hr Heat, Steam H O, CO , CO, H , NH , Pyrolysis 2 2 2 3 62 kg 1 kg/hr Power CH4, Ash</p><p>Plasma H2O, N2, Trace gases, Ash 1413 kg 50 kg/hr Power, Air</p><p>One of the functions that Pyrolysis fails to provide is the ability to process metals. Even when Pyrolysis does break down small amounts of inorganic compounds, including metals, these types of waste should be avoided because of problematic clean up issues caused by the post-process residues. The approach to handle this type of waste, which will be mainly comprised of filter components, will be taken care of by storing the used filters during transit, and then, once in the surface habitat, these filters can be disassembled to remove the non-processable components. The leftovers are treated with Pyrolysis, thus reducing the amount of space that this type of waste occupied originally.</p><p>7.6 The Integrated System The WP Subsystem consists of these major components (See Figure 7-13):</p><p> Urinal Commode Bacteria/Odor Filter Compactor Pyrolysis Processable Materials Storage area Non-processable Materials Storage area</p><p>A mass, power and volume breakdown for each subsystem component is presented in Table 7-19. Table 7-19. System Components Mass Summary Total Mass (kg) System Component Power (kW) Volume (m3) (Includes spares) Toilet Assembly 16.33 0.224 0.078 Biological Waste Tank 17.40 0.000 0.100 Compactor 25.94 0.110 0.355 Pyrolysis 84.00 0.600 0.002 Non-processables Storage Tank 50.00 0.000 2.000 Chemicals 41.00 0.000 0.043</p><p>The collecting scheme for human waste follows the basic design principles used in the Space Shuttle (NASA-WCS 2102C). Urine and feces are collected separately by means </p><p>Earth Replications, Inc. Page 41 ASEN5116 Final Report Spacecraft Life Support Systems of a urinal and a commode. Feces and other decomposable matter such as food remains will be stabilized through chemical treatment to prevent/reduce bacterial growth while in storage, in a similar fashion to the products used on portable toilets. Several companies such as Walex Products (Walex 2002) and Toico Industries (Toico 2002) provide formaldehyde-free chemicals that not only disinfect, but help in the decomposition of the waste, thus reducing its volume. The feces’ residence time inside the biological waste tank will be minimum, as the goal will be to treat all processable waste on a continuous basis. Freeze drying (Lyophilization) was the alternate method considered for stabilization, however, chemical treatment is more appealing since it translates to less mass, power and volume penalties, in addition to eliminating the complexity of adding another mechanical component to the system. The AM Subsystem will take an integral part in the WP scheme by integrating a bacteria/odor filter for the re-circulated air around the urinal/commode area. </p><p>All other processable waste, such as plastics and trash from the FS Subsystem, will be placed in sealed plastic bags in a storage area. For pretreatment, this system will utilize a compactor to reduce waste volume prior to feeding it into the Pyrolysis unit.</p><p>Non-processable waste To Water from all subsystems Management</p><p>Commode (Feces) Urinal Other Subsystems Non-processable Subsystems H O 2 Materials Storage STORAGE All other Biological Pyrolysis processables Waste Carbon (plastics, paper, etc) Tank</p><p>Compactor</p><p>STORAGE Recycling</p><p>CO2, H2, NH3, CH4, NOx, SOx To Atmosphere Management</p><p>Figure 7-13.Waste Processing Subsystem Diagram</p><p>Finally, the Pyrolysis unit will accept all the processable waste (including the feces’ stabilizing chemical agent) and convert it into a gaseous and solid output. The resulting gases from this process (mainly CO2, H2, NH3, CH4, H2O, NOx and SOx) will be accepted by the AM Subsystem, except for H2O vapor, which will be condensed and sent to the WM Subsystem for further treatment. The residual carbon will be stored in the Non-</p><p>Earth Replications, Inc. Page 42 ASEN5116 Final Report Spacecraft Life Support Systems</p><p>Processable Materials Storage area for possible future use or to be discarded if no further processing is available.</p><p>7.7 Failure Analysis Equipment failure is highly probable on missions of extended duration. While design should provide for preventing malfunctions, no system is infallible. Failures must therefore be dealt with in planning. Table 7-20 summarizes the WP Subsystem approach to failures of the main components of the technologies previously described. Two levels of redundancy are provided for every major step in the WP cycle, most of which includes replacement of the faulty part followed by temporary storage, and/or dumping of waste if necessary.</p><p>Table 7-20 Waste Processing Subsystem Contingency Approach</p><p>Failure Redundancy (1st level) Redundancy (2nd level) Diapers (males/females) or Urinal vacuum Repair with spares. urine collection in plastic failure bags (males). Diapers or collection in bags Commode Repair with spares. (males/females). Process waste without Compactor Repair with spares. compaction. Repair with spares. Store Pyrolysis waste in the meantime. Store and dump waste as reactor failure Redundant unit will be in necessary. place at Mars base. Replace. Minimal capability Repair with sealant. Pipe leakage as not many spares will be Adjust connections. available. One of the most critical failures of the WP Subsystem revolves around the Pyrolysis unit. Major failure of main combustion shell/reactor in the Pyrolysis unit may result in higher concentration of contaminants in effluent due to incomplete combustion, which in turn might put a heavier load on the AM subsystem. The immediate contingency will be to store waste and replace the defective parts. As a last resource, excess material will be dumped while in transit.</p><p>7.8 Conclusions and Recommendations The WP Subsystem design complies with all the requirements established by the DRM. It provides storage for non-processable items and treatment for those that can be processed to recover and recycle resources. Pyrolysis is chosen as the technology that best balances functionality with overall system mass and power consumption. Challenges that accompany this technology include separation of the large volume and complex mixture of product gases. Incorporation of a carbon activation step would reduce non-processable</p><p>Earth Replications, Inc. Page 43 ASEN5116 Final Report Spacecraft Life Support Systems waste and increase the amount of reusable by-products. The WM Subsystem can make use of the activated carbon for trace contaminant control.</p><p>There is a great need for more in depth research for the technologies considered in this study. Since most of the technologies have a relatively low TRL (less than 5), there is a lack of experimental data showing how these systems operate under various gravitational conditions. </p><p>Although multiple aspects of waste processing have been considered, the issue of soil contamination from the Martian surface to the living headquarters will have to be addressed. Many EVAs will certainly contribute to dust accumulation and possibly hindrance of systems such as atmosphere particle control.</p><p>7.9 Waste Management Subsystem References</p><p>1. Davidson, William. 2002. Supercritical Water Oxidation: State of the Art Environmental Technology. General Atomics Advanced Technologies Group. Available from the World Wide Web: http://www.ga.com/atg/aps/scwo.html 2. Eckart, Peter. 1996. Spaceflight Life Support and Biospherics. Torrance: Microcosm Press 3. Ferrall, J.F., G.B. Ganapathi, N.K. Rohatgi and P.K. Seshan. 1994. Life Support Systems Analysis and Technical Trades for a Lunar Outpost. NASA Technical Memorandum 109927 4. Horneck, G., DLR, "Advanced Life Support Systems and Human Exploratory Missions", 2nd Workshop on Advanced Life Support, ESTEC, 17-18 April 2002. 5. NASA Research Opportunities Online. 2002. Advanced Life Support Program (ALS) and the Advanced Environmental Monitoring and Control (AEMC) Program Technology Assessment Matrix. Available from the World Wide Web: http://research.hq.nasa.gov/code_u/matrix/index.cfm. 6. Verostko, C., J. Joshi, M. Alazraki and J. Fisher. 2002. Solid Waste Processing and Resource Recovery Workshop Report - Vol I. Engineering Directorate, Crew and Thermal Systems Division. CTSD-ADV-474. Available also from the World Wide Web: http://advlifesupport.jsc.nasa.gov/PubNew.html 7. Verostko, C., J. Joshi, M. Alazraki and J. Fisher. 2002. Solid Waste Processing and Resource Recovery Workshop Report Appendix - Vol II. Engineering Directorate, Crew and Thermal Systems Division. CTSD-ADV-474. Available also from the World Wide Web: http://advlifesupport.jsc.nasa.gov/PubNew.html 8. Hanford, A. J. and M. K. Ewert. 2001. Advanced Life Support Systems Integration, Modeling, and Analysis Reference Missions Document. Engineering Directorate, Crew and Thermal Systems Division. CTSD-ADV-383. Available also from the World Wide Web: http://advlifesupport.jsc.nasa.gov/PubNew.html</p><p>Earth Replications, Inc. Page 44 ASEN5116 Final Report Spacecraft Life Support Systems</p><p>9. Hanford, A. J., M. K. Ewert and D. L. Henninger. 2002. Advanced Life Support Baseline Values and Assumptions Document. Engineering Directorate, Crew and Thermal Systems Division. CTSD-ADV-383. Available also from the World Wide Web: http://advlifesupport.jsc.nasa.gov/PubNew.html 10. National Aeronautics and Space Administration. Waste Collection System Workbook WCS 2102C. November 26, 1984. Johnson Space Center. Advanced Training Series. Training Division. Systems Training Branch. 11. Walex Products, Portable Sanitation, <http://www.walex.com/pages/portable.html> (9 December 2002). 12. Toico Industries, Cleaning Supplies, <http://www.toico.com/cleaningsupplies.html> (9 December 2002).</p><p>Earth Replications, Inc. Page 45 ASEN5116 Final Report Spacecraft Life Support Systems</p><p>8.0 FOOD SUPPLY 8.1 Baseline Functional Requirements Food Supply needs to supply the six crew members with the proper amount of protein, carbohydrates and fat daily for the mission duration. Below, Table 8-8-21 shows the input masses of these basic food constituents. These masses correlate with the Metabolic Load Balance shown in section 2.3.</p><p>Table 8-8-21. Mass of Food Type (kg/person-day)6 Food Type Chemical Carbon Hydrogen Oxygen Nitrogen Total Composition</p><p>Protein C4H5ON 0.0770 0.0081 0.0257 0.0255 0.1332</p><p>Carbohydrate C6H12O6 0.1489 0.0250 0.1984 0 0.3723</p><p>Fat C16H32O2 0.0858 0.0144 0.0143 0 0.1145</p><p>The above table shows values before the 10% per person-day safety factor is added. Therefore, the total mass for each food type is now is shown Table 8-22,</p><p>Table 8-22. Food Type Mass Food Type Mass (kg/person-day) Protein 0.1465 Carbohydrate 0.4095 Fat 0.1260 Total Daily Allowance 0.6820 (dry) Water Content 1.2650 Total Daily Allowance 1.9470 (wet)</p><p>This will provide each crew member with a low fat, high carbohydrate diet with the following percentages shown in Table 8-23.</p><p>Table 8-23. Percent of Food Type Per Daily Meal Food Type Percentage of Daily Meal Protein 25% Carbohydrate 70% Fat 5%</p><p>US Recommend Daily Allowances (USRDA) show that a minimum of 2000 kcal/day is needed to provide the minimum amount of nutrients and vitamins. Based on the above amounts, Table 8-24 shows the amount of calories that is to be provided.</p><p>Earth Replications, Inc. Page 46 ASEN5116 Final Report Spacecraft Life Support Systems</p><p>Table 8-24. Caloric Provision Calories per Food Type Mass in grams gram8 Total Calories Protein 146.630 4 586.520 Carbohydrate 409.530 4 1638.120 Fat 12.595 9 113.355 Total kcals 2337.995</p><p>Table 8-25 and Table 8-26 show the recommended amounts of vitamins and minerals for mission duration ranges. This is a requirement to provide nutritional meals to the crew members, but was not included in the trade study.</p><p>Table 8-25. Daily Recommended Vitamin Intake for Mission 30 day to 1 year2 Nutrient Recommendation Amount Vitamin A 1000μg Vitamin D 10 μg Vitamin E 20 mg Vitamin K 65-80 μg Vitamin C 100 mg Vitamin B12 2 μg Vitamin B6 2 mg Thiamin 1.5 mg Riboflavin 2 mg Folate 400 μg Niacin 20 mg Biotin 100 μg Pantothenic Acid 5 mg</p><p>Earth Replications, Inc. Page 47 ASEN5116 Final Report Spacecraft Life Support Systems</p><p>Table 8-26 Daily Recommended Mineral Intake for Mission 30 day to 1 year2 Nutrient Recommendation Amount Calcium 1000-1200 mg Phosphorus 1000-1200 mg Magnesium 280-350 mg Sodium <3500 mg Potassium 3500 mg Iron 10 mg Copper 1.5-3.0 mg Manganese 2.0-5.0 mg Fluoride 4.0 mg Zinc 15 mg Selenium 70 μg Iodine 150 μg Chromium 100-200 μg</p><p>8.2 Baseline Architecture The baseline open loop design concept is to bring everything along at launch with no re- supply. Therefore, the Food Supply subsystem will need to bring along the food and preparation water for the entire mission duration of 780-days (transit and surface stay). Figure 8-14 shows the basic open loop food subsystem block diagram. shows the masses for the food subsystem components.</p><p>Earth Replications, Inc. Page 48 ASEN5116 Final Report Spacecraft Life Support Systems</p><p>Fresh Food Storage </p><p>Freezer Oven Microwave </p><p>Heat </p><p>H2O Food Human Preparation </p><p>Waste Waste </p><p>Figure 8-14 Food Subsystem Baseline Open Loop Design</p><p>Table 8-27. Baseline Open Loop Subsystem Component Mass Component Description Mass (kg) Mass for 6 Crew Members Per Day (kg) Fresh Food (wet) 1.9470 (person-day) 11.682 Packaging 0.5/day N/C Freezer/Refrigerator 400/unit N/C Microwave 35/unit N/C Oven 50/unit N/C Food Prep Water 0.8690 (person-day) 5.214 Total 488.316 502.396</p><p>Using the numbers in , the total mass needed for the Food Supply Subsystem can be calculated for the entire mission duration. Table 8-28 shows the total mass per phase and the total mass. Table 8-28. Total Subsystem Baseline Mass Phase Mass (kg/six-CM) Transit (180 days) 3616.20 Surface Stay (600 days) 10173.60 Total Subsystem Mass 13789.80</p><p>Earth Replications, Inc. Page 49 ASEN5116 Final Report Spacecraft Life Support Systems</p><p>8.3 Subsystem Assumptions Assumptions made throughout the trade study process are identified. At a high level, assumptions must be stated to allow proper interface between subsystems. In the subsystem level, it is necessary to record all assumptions to ensure accurate comparison between technologies. The following assumptions have been made when designing the Food Supply Subsystem:</p><p> Any Biological Regenerable Plant Growth system will only be a supplement not a back up to the base line food supply scheme. Food will be allotted by daily allowances. Trading one day’s meals for another will be allowed, but consumption will be limited to one daily allowance per day. Each daily allowance will met the minimum RDA levels of vitamins and minerals (per Table 8-25 and Table 8-26). 10% for each person/day was added to the baseline daily requirements for Protein, Carbohydrates and Fat. This assumption will provide: o Additional food for variance in kcal/person intake o Additional food for contingency (mission duration is longer than planned) o Additional food for variance in eating habits between crew members Packaging mass is assumed to be 0.5 kg/day of food.7 Water to prepare or re-hydrate any food will be provided by the water management subsystem. The choose food technology type will contain the minimum vitamin and mineral content shown in Table 8-5 and 8-6 above.</p><p>8.4 Candidate Technologies A preliminary list of candidate technologies has been established to satisfy the requirements for the Food Supply Subsystem. The candidate technologies are identified in Table 8-29 and are mapped to the function that they help provide. Biological technologies shown here are for additional information only and were not part of the trade study. The DRM constrained the design to using Physio-Chemical Technologies as the primary system basis, and biological only as an experimental supplement. </p><p>Earth Replications, Inc. Page 50 ASEN5116 Final Report Spacecraft Life Support Systems</p><p>Table 8-29. Candidate Technologies for Food Supply Food Supply Function Candidate Technologies 3 Rehydratable Food 3 Thermostabilized Food 3 Intermediate Moisture Food 3 Natural Form Food 3 Irradiated Meat</p><p>Candidates but not as primary source 4 Algae 4 Fungi Supply Food 4 Hydroponics – Vegetable Plant Growth o Nutrient Film Technique5 o Gravel Culture System5 o Sand Culture System5 o Sawdust Culture System5 o Rockwool Culture System5 4 Aeroponics 4 Zenoponics 4 Animals as food 4 Aquaculture</p><p>8.5 Subsystem Technology Trade Study For the food supply subsystem trades, the overall mass was the driving trade variable. The goal of the trade study was to minimize overall subsystem mass while still providing all the nutrient and mineral requirements to the crew members. The four main food types used in the trade were; Dehydrated, Fresh/Natural Form, Frozen and Thermo-stabilized. Figure 8-15 shows a comparison of subsystem mass including consumables using all of one type or a mix. </p><p>Earth Replications, Inc. Page 51 ASEN5116 Final Report Spacecraft Life Support Systems</p><p>Total Food Weight Comparison</p><p>12000</p><p>10000</p><p>8000</p><p>Total Weight in Thermal Stablized 6000 Kilograms (kg) Frozen Dehydrated 4000 Fresh</p><p>2000</p><p>0 1 2 3 4 5 6 7 8 9 10 11 12 13 14 15 16 17 18 19 20 21 Trade Case Number</p><p>Figure 8-15 Food Supply Comparison Graph</p><p>8.5.1 Results From Figure 8-15 it can be seen that a food supply solely of dehydrated food is the lowest overall mass. Figure 8-16 shows the new system. The oven and freezer/refrigerator are no longer needed, because all the food is dehydrated. Also, the water content within the food is no longer an additional mass to the food supply subsystem. In the integrated design, the water content of the food is negligible and the re-hydration water will come from the water management subsystem. This differs from bringing all the re-hydration water along which was the baseline open loop design.</p><p>Earth Replications, Inc. Page 52 ASEN5116 Final Report Spacecraft Life Support Systems</p><p>Fresh Food Storage </p><p>Heat Microwave Microwave </p><p>H2O Food Human Preparation </p><p>Waste Waste </p><p>Figure 8-16 Closed Loop Design for Food Supply Subsystem</p><p>Table 8-10. Closed Loop Mass for Food Supply Subsystem</p><p>Add. Weight Total Weight Component # Weight (kg) (kg) (kg) Dehydrated Food 1 3612.27 722.45 4334.73 Microwave 2 70.00 14.00 154.00 Totals 4488.73</p><p>Comparing to the 13789.80 kg subsystem mass for the open loop design, closing the water loop to provide food prep water and removing the water content in the food supply is a large savings. The trade and redesign has provided a mass savings of 32.5% for the food supply subsystem.</p><p>8.6 Food Supply Subsystem References 1. Hoffman, S.J., Kaplen, D.L., “Human Exploration of Mars: The Reference Mission of the NASA Mars Exploration Study Team”; 1997 2. NASA FTGSC Space Food Insights http://www.ag.iastate.edu/centers/ftcsc/pages/insig.htm 3. ASEN 5116 Class Notes, “Food Supply”; November 12, 2002. University of Colorado – Boulder. http://www.colorado.edu/ASEN/asen5116/Lect-18.htm 4. Spacecraft Life Support and Biospherics, Eckart, 1996.</p><p>Earth Replications, Inc. Page 53 ASEN5116 Final Report Spacecraft Life Support Systems</p><p>5. Hydroponic Food Production, Horward M. Resh, Ph.D, 2001 6. Ferral, J.F., Ganapathi, G.B., Rohatgi, N.K., Seshan, P.K., “Life Support Systems Analysis and Technical Trades for a Lunar Outpost” NASA Technical Memorandum 109927, 1994. 7. Larson, W.J., Pranke, L.K., Human Spaceflight: Mission Analysis and Design, MacGraw-Hill, New York. 8. Guyton, A.C., Hall, J.E., Textbook of Medical Physiology, W.B. Saunders Company, Pennsylvania, 2000.</p><p>Earth Replications, Inc. Page 54 ASEN5116 Final Report Spacecraft Life Support Systems</p><p>9.0 SYSTEM INTEGRATE DESIGN RESULTS 9.1 System Architecture The integrated system is comprised of four subsystems: Atmosphere Management (AM), Food Supply (FS), Water Management (WM), and Waste Processing (WP). The details of each subsystem are discussed in earlier sections and the interactions are highlighted here. The main interactions within the system are between AM, WM, and WP. AM takes inputs from WP in the form of output gases from the Pyrolysis system and outputs CO2 to WP as feed gases to the Pyrolysis system. WP takes input of all wastes from AM, FS, and WM. The WM subsystem takes in H2O produced by WP during pyrolysis processing as well as the used water from laundry and hygiene. WP outputs potable and hygiene water to FS. The interactions are shown in Figure 9-17. Figure 9-18 shows the overall system diagram for the surface stay on Mars. The subsystem interactions remain the same except, except that the AM subsystem interfaces with ISRU plant on Mars to take advantage of the Martian resources. </p><p>Earth Replications, Inc. Page 55 ASEN5116 Final Report Spacecraft Life Support Systems</p><p>Atmosphere Food Pressure Control CH CH4 Dehydrated N2 CO2 Food CO2 , H2 C</p><p>SOE O2 Food CO2 HUMAN 2BMS Preparation G G C a a Low CO2 Air O s s 2</p><p>S S r</p><p> i HEPA e c e TCCS h p p C C Filter A a a O O r r i r 2 a 2 a Humidity , N , FDS Microwave t</p><p> t</p><p>GC/MS H H o H o Control 3 2 r 2 r , , , N</p><p>N N O H H x Air , Human Accommodations 3 3 S , , O</p><p>C C x HUMAN H H 4 4 , ,</p><p>O N N HUMAN 2 O2</p><p>O 2 O NH3, Nox Hygiene x x , , SO</p><p> x</p><p>S S O O CO2 x x Laundry Vent</p><p>Processable Materials Urine Pretreatment Urinal Commode Storage HUMAN Oxone Pretreated Urine VCD AES (Feces) F Compactor e Hygiene H O</p><p> c 2 Sulfuricacid</p><p>Other waste e B s Brine r i n H O e 2</p><p>Iodine Removal Bed H 2 HUMAN O Ultra Filtration Pyrolysis RO ISE Monitoring MCV Monitoring Milli Q Iodine Non-processable Materials Materials Potable H O Waste Storage 2 Water</p><p>H2O</p><p>Figure 9-17 System Block Diagram – Transit</p><p>Earth Replications, Inc. Page 56 ASEN5116 Final Report Spacecraft Life Support Systems</p><p>Atmosphere Food Pressure Control CH 4 Dehydrated N2 CH4 A A A A t t t t Food m m m m CO2 , H2 M M o o o o s a s s a s p r p p r p Sabatier s s h h h h Food CO2</p><p> e 2 e e e HUMAN r r r r TSA Preparation e e</p><p> e Preparation e G G C a a O s s Low CO 2 Air 2</p><p>S S r i e c e h</p><p> p HEPA p</p><p>C TCCS C A a a O O Filter</p><p> r H i r H2 r a a 2 2 , , N H O t 2 Microwave t Microwave</p><p> o Humidity o H H H GC/MS FDS r r 3 2 2</p><p>, Control ,</p><p>,</p><p>N</p><p>N N O H H x ,</p><p>Human Accommodations</p><p>3 Human Accommodations 3 S Air , , O</p><p>C C x HUMAN H H 4 4 , ,</p><p>SPWE SPWE N N HUMAN O2 O O O2 O NH 3, Nox Hygiene x x , , SO</p><p> x</p><p>S S ISRU O O CO 2 x x Laundry</p><p>Processable Materials Urine Pretreatment Urinal Commode Storage HUMAN Urinal Commode Oxone Pretreated Urine VCD AES (Feces) F Compactor e c Hygiene H 2O Sulfuricacid e</p><p>Other waste s B</p><p> r Brine i n</p><p> e H 2O</p><p>Iodine Removal Bed H 2 HUMAN O Ultra Filtration Pyrolysis RO ISE Monitoring MCV Monitoring Milli Q Iodine Non-processable Materials Potable H O Waste Storage 2 Water</p><p>H2O</p><p>Figure 9-18 System Block Diagram - Surface</p><p>Earth Replications, Inc. Page 57 ASEN5116 Final Report Spacecraft Life Support Systems</p><p>9.2 Mass Balance Results 9.3 Equivalent System Mass Results The integrated system design ESM was calculated with the information provided in Figure 9-19. This figure show the breakdown of each subsystem and its’ components and the values that were obtain or estimated for Mass, Power and Volume. The additional weight column provides inclusion of consumables, such as LiOH canisters, piping and storage tank components.</p><p>Earth Replications, Inc. Page 58 ASEN5116 Final Report Spacecraft Life Support Systems</p><p>CLOSED LOOP ESM Atmosphere Add. Total Total Total Crew Weight Weight Weight Power Power Volume Volume Time Component # (kg) (kg) (kg) (kW) (kw) (m3) (m3) (hrs/day) Portable Fire Extinguisher (PFE) 3 15.10 0.00 45.30 0.00 0.00 0.04 0.12 0.00 Scattering Smoke Detector 8 1.50 0.00 12.00 0.00 0.02 0.04 0.32 0.00 Portable Breathing Apparatus 12 2.80 0.00 33.60 0.00 0.00 0.31 3.72 0.00 UV/IR Flame Detector 3 3.70 0.00 11.10 0.10 0.30 0.00 0.01 0.00 Pressure Control 1 74.00 0.00 74.00 0.10 0.10 0.41 0.41 0.00 Pressure Control Sensors 2 2.00 0.00 4.00 0.01 0.01 0.00 0.03 0.00 Gas Chromatograph/Mass Spectrometer (mini) 3 1.80 0.00 5.40 0.02 0.05 0.03 0.09 0.00 Trace Contaminant Control Subassembly (TCCS) 1 78.20 163.00 241.20 0.18 0.18 0.50 0.50 0.01 HEPA Filters 15 2.00 260.00 290.00 0.00 0.00 0.10 1.50 0.01 Common Cabin Air Assembly 2 112.00 0.00 224.00 0.47 0.94 0.40 0.80 0.00 Intermodule Ventilation Fan 2 4.76 0.00 9.52 0.55 1.10 0.01 0.02 0.00 Intermodule Ventilation Valve 12 5.10 0.00 61.20 0.01 0.07 0.01 0.12 0.00 Cabin Fan Assembly 1 26.90 0.00 26.90 0.41 0.41 0.15 0.15 0.00 2 Bed Molecular Sieve 2 96.00 28.80 220.80 0.50 0.50 0.60 1.20 0.00 Solide Oxide Electrolysis 2 220.00 44.00 484.00 6.50 6.50 0.80 1.60 0.00 Oxygen Buffer Tank 1 100.00 34.00 134.00 0.00 0.00 0.45 0.45 0.00 Nitrogen Buffer Tank 1 26.00 18.90 44.90 0.00 0.00 0.25 0.25 0.00 Totals 1921.92 10.16 11.28 0.02</p><p>Water Add. Total Total Total Crew Weight Weight Weight Power Power Volume Volume Time Component # (kg) (kg) (kg) (kW) (kw) (m3) (m3) (hrs/day) VCD 1 101.00 43.80 144.80 0.12 0.12 0.49 0.49 0.00 AES 1 45.00 30.00 75.00 1.00 1.00 0.30 0.30 0.01 UF/RO 2 90.00 34.00 214.00 0.60 0.60 0.35 0.70 0.01 MiilQ 2 11.00 85.68 107.68 0.00 0.00 0.06 0.12 0.01 MCV 4 10.00 3.80 43.80 0.01 0.01 0.06 0.24 0.00 Iodine removal bed 2 5.00 10.00 20.00 0.00 0.00 0.06 0.12 0.01 Quality Monitor 2 39.00 12.00 90.00 0.08 0.08 0.08 0.16 0.08 Urine/RO Brine Storage Tank 1 3.60 0.00 3.60 0.00 0.00 0.06 0.06 0.00 VCD Brine Storage Tank 1 5.00 0.00 5.00 0.00 0.00 0.08 0.08 0.00 Water Storage 1 32.43 0.00 32.43 0.20 0.20 0.61 0.61 0.00 Plumbing 20% Hardware 1 101.40 0.00 101.40 0.00 0.00 0.06 0.06 0.00 Buffer Water 1 324.28 0.00 324.28 0.00 0.00 0.00 0.00 0.00 Totals 1161.99 2.01 2.94 0.12</p><p>Waste Add. Total Total Total Crew Weight Weight Weight Power Power Volume Volume Time Component # (kg) (kg) (kg) (kW) (kw) (m3) (m3) (hrs/day) Toilet assembly 1 16.00 19.20 35.20 0.22 0.22 0.08 0.08 0.08 Septic tank 1 14.50 2.90 17.40 0.00 0.00 0.10 0.10 0.00 Compactor 1 15.10 10.84 25.94 0.11 0.11 0.36 0.36 0.00 Pyrolysis 1 60.00 24.00 84.00 0.60 0.60 0.00 0.00 0.08 Non-processables Storage 1 50.00 0.00 50.00 0.00 0.00 2.00 2.00 0.08 Totals 212.54 0.93 2.54 0.25</p><p>Food Add. Total Total Total Crew Weight Weight Weight Power Power Volume Volume Time Component # (kg) (kg) (kg) (kW) (kw) (m3) (m3) (hrs/day) Dehydrated Food 1 3612.27 722.45 4334.73 0.00 0.00 37.00 37.00 3.50 Microwave 2 70.00 14.00 154.00 1.80 3.60 0.15 0.30 0.00 Totals 4488.73 3.60 37.30 3.50</p><p>Grand Totals 7785.18 16.71 54.06 3.89</p><p>Figure 9-19. Final Design Subsystem Mass, Power and Volume</p><p>Earth Replications, Inc. Page 59 ASEN5116 Final Report Spacecraft Life Support Systems</p><p>By using the Equations (1), the total system ESM was calculated to be 7,791 kg.</p><p>ESM total TotalMass 87* Power 1.54*CrewTime (1)</p><p>This is approximately a 102 order of magnitude savings over the Open Loop Design. Even though there are more components to the final design, the mass savings comes mostly from closing the water loop. Instead of bringing all the water that the six crew members will need, the system recycles and reclaims most of the water needed daily.</p><p>Earth Replications, Inc. Page 60 ASEN5116 Final Report Spacecraft Life Support Systems</p><p>10.0 SYSTEM CONCLUSIONS & RECOMMENDATIONS This report represents a 2nd iteration design of a life support system. The final system design shows a significant improvement over the open loop design, as seen in the final equivalent system mass results. Further improvements can be made with more iterations of the design process. With further iteration of the design, more loop closure can certainly be obtained. Also, detailed mass flows of all inter-subsystem products should be computed in more detail to insure proper interaction of the technologies.</p><p>Further development and testing of promising technologies needs to be done to improve TRLs. Particularly, further research on Advanced Carbon Formation Reactors (ACFR), Water Vapor Electrolysis (WVE), and Ammonia Dissociation would help to gain further loop closure of the overall system. Particularly, Ammonia Dissociation systems would allow for further closure of the N2 loop. Additionally, in the waste processing technologies, further development of charcoal reactivation methods would decrease the overall resupply mass of the system. Charcoal reactivation would eliminate the need to take resupply charcoal for such systems as trace contaminant control. Also, soil removal (from EVAs) was not addressed in this study, but is recognized to be a potential problem that needs to be solved.</p><p>Earth Replications, Inc. Page 61 ASEN5116 Final Report Spacecraft Life Support Systems</p><p>11.0 APPENDIX A: REQUIREMENTS</p><p>Earth Replications, Inc. Page 62 ASEN5116 Final Report Spacecraft Life Support Systems</p><p>Rqmt Type (C,F, Rqmt # P,D) I/Fs Requirement Source AM,WM, Limit length of time that the crew is continuously exposed to the interplanetary C S010 WP,FS space environment (6 months - 8 months max). DRM Page 1-7 DRM Page 1-8 AM,WM, The system shall support surface operations for during of 600 days (plus safety C S020 WP,FS buffer). DRM Page 1-7 AM,WM, The system shall use in-situ resources to the extent possible to support surface C S030 WP,FS duration. DRM Page 1-7 AM,WM, F S040 WP,FS The system shall support a crew of 6 during entire mission. DRM Page 1-13 AM,WM, D S050 WP,FS Deployment of Bioregenerative life support system is to augment the LSS only. DRM Page 1-14 D AM, WM S060 The system shall use in-situ resources to produce (water, O2, N2 and Argon). DRM Page 1-16 AM,WM, C S070 WP,FS The entire mission must be completed without resupply from Earth. DRM Page 1-16 AM,WM, Transit Habitat must operate in 1-G Micro-G, Martian-G (3/8-G) and F S080 WP,FS Launch/Decent. DRM Page 1-21 AM,WM, D S090 WP,FS During Transit power source is Solar Arrays ( 30 KW). DRM Page 1-22 DRM Page 3-98 AM,WM, During Surface power source is 2 Nuclear reactors (Primary and Backup each D S100 WP,FS capable of 160 KW) (50 kW) and solar arrays used during flight. DRM Page 1-22 (Appendix A.3.2.2 page 2) S110 F AM The system must provide contamination and particle control. DRM Page 1-35 AM,WM, C S120 WP,FS The system must have as much loop closure as possible. DRM Page 1-35 AM,WM, F S130 WP,FS The system shall promote introduction of locally produced consumables. DRM Page 1-35 S140 F FS The system must be able to produce food. DRM Page 1-35 S150 F WP The system must collection and process trash and waste. DRM Page 1-35 The system must have a high efficiency and lighter weight active thermal P AM S160 control system. DRM Page 1-35 S170 F AM The system must provide fire detection, prevention and suppression. DRM Page 1-35 AM,WM, D S180 WP,FS Total system mass should not exceed 10.7 tons. DRM Page 3-82 (Appendix A.3.2.2 page 2) AM,WM, D S190 WP,FS Life Support mass should not exceed 4661 kg. (Appendix page 3 of 8, Table A4-2) AM,WM, D S200 WP,FS Total system power should not exceed 12.7 KW. DRM Page 3-93</p><p>Earth Replications, Inc. Page 63 ASEN5116 Final Report Spacecraft Life Support Systems</p><p>System Requirements Rqmt Type (C,F, Rqmt # P,D) I/Fs Requirement Source AM,WM, F S210 WP,FS The system should have two backup levels functional redundancy. DRM Page 3-99 AM,WM, Crews will have the capability to explore in the vicinity of the surface outpost F S220 WP,FS out to 500 kilometers. DRM, page 1-8 AM,WM, Long-range surface rovers will have the capability to operate at a remote site F S230 WP,FS for up to 10 days with total trip time of less than 14 days. DRM, page 1-23 AM,WM, During remote site operations, up to 16-hours will be available for EVA F S240 WP,FS operations. DRM, page 1-23 & 3-50 AM,WM, Long-range surface rovers will have the capability to carry 2 persons, or 4 F S250 WP,FS persons in the event of an emergency. DRM, page 1-23 AM,WM, Systems must be highly reliable and highly autonomous to improve the P S260 WP,FS effectiveness of surface operations. DRM, page 1-8 AM,WM, The system should allow for 2 emergency EVAs during transit by 2 crew F S270 WP,FS members with an 8-hour duration. DRM Addendum, page 15 AM,WM, The system should allow for 1 8-hour EVA per week during surface F S280 WP,FS operations. DRM Addendum, page 15-16 AM,WM, C Mars must be protected from biological contamination from Earth that would WP,FS S290 interfere with or confound the search for natural Martian organisms. DRM, page 1-37 AM,WM, D S300 WP,FS The first level of life support redundancy should be fully automated. DRM, page 3-15 AM,WM, The Earth-Mars and Mars-Earth transit times will not exceed 180 days and C S310 WP,FS will not be less than 120 days. DRM, page 3-42 AM,WM, All life support systems should use common hardware and software whenever D S320 WP,FS possible. DRM, page 3-61 AM,WM, F S330 WP,FS Life critical systems will require automatic fault detection. DRM, page 3-62 AM,WM, The earth return vehicle life support system will be able to operate C S340 WP,FS autonomously for up to 4 years. DRM, page 3-86 The earth return and crew transit/surface habitat vehicles will have an internal AM,WM, D volume defined by a 7.5 meter diameter cylinder that is 4.6 meters long with S350 WP,FS elliptical end-caps. DRM, page 3-90</p><p>Earth Replications, Inc. Page 64 ASEN5116 Final Report Spacecraft Life Support Systems</p><p>System Requirements Rqmt Type (C,F, Rqmt # P,D) I/Fs Requirement Source The earth return and crew transit/surface habitat vehicles will have an internal AM,WM, D habitable space defined by the 7.5 meter diameter cylinder, separated into 2 WP,FS S360 floors of 3 meters each. DRM, page 3-90 The crew transit/surface habitat vehicle will have an inflatable Trans/Hab with AM,WM, D an internal volume defined by a 7.5 meter diameter cylinder that is 4.6 meters WP,FS S370 long with elliptical end-caps. DRM, page 3-90; assumption for size The earth return vehicle and crew transit/surface habitat vehicle will have no D AM, WM S380 more than 0.5 kg/day of gas leakage. Larson & Prank, 2000, page 568 The earth return vehicle and crew transit/surface habitat vehicle will have D AM S390 airlocks with an internal volume of 2 m3. Larson & Prank, 2000, page 568 Life support systems shall provide a sufficient total pressure to prevent the D AM S400 vaporization of body fluids NASA MSIS, section 5.1.2.1 Life support systems shall provide free oxygen at sufficient partial pressure for D AM S410 adequate respiration. NASA MSIS, section 5.1.2.1 Life support systems shall provide oxygen at a partial pressure not so great as D AM S415 to induce oxygen toxicity. NASA MSIS, section 5.1.2.1 Life support systems shall, for long durations (in excess of two weeks), provide D AM S420 some physiologically inert gas to prevent atelactasis. NASA MSIS, section 5.1.2.1</p><p>D AM All other atmospheric constituents provide by the life support system must be S430 physiologically inert or of low enough concentration to preclude toxic effects. NASA MSIS, section 5.1.2.1 Life support systems shall provide a breathing atmosphere composition that D AM S435 will minimize flame/explosive hazard. NASA MSIS, section 5.1.2.1 D AM S440 Life support systems shall provide an ambient temperature 18.3-26.7 deg C. NASA MSIS, section 5.8.3.1; Eckart (1996), page 92 S450 D AM, WM Life support systems shall provide a relative humidity of 25-70%. NASA MSIS, section 5.8.3.1; Eckart (1996), page 92 S460 D AM Life support systems shall provide cabin ventilation of 0.076-0.203 m/s. NASA MSIS, section 5.8.3.1; Eckart (1996), page 92 Life support systems shall provide the capability to limit trace contaminants to D AM the Space Maximum Allowable Concentration (SMAC) for the duration of the S470 mission. Eckart (1996), page 94</p><p>Earth Replications, Inc. Page 65 ASEN5116 Final Report Spacecraft Life Support Systems</p><p>System Requirements Rqmt Type (C,F, Rqmt # P,D) I/Fs Requirement Source Each crew member will have a nominal daily schedule for the duration of the mission of: AM,WM, - 8 hours sleep D WP,FS - 5 hours light work (e.g. leisure activities) - 9 hours medium work (e.g. standard work duties) S480 - 2 hours heavy work (e.g. exercise) Eckart (1996), page 93</p><p>AM,WM, During crew work periods, the summation of the individual sound pressure D WP,FS levels from all operating systems and subsystems should not exceed exposure S490 limits that will cause hearing loss or interfere with voice communication. NASA MSIS, section 5.4.2.1</p><p>AM,WM, During crew sleep and rest periods, noise levels should not exceed noise levels D WP,FS that interfere with sleep or comfort and the hearing of wanted sounds. S500 NASA MSIS, section 5.4.2.1 Vibration generation and penetration shall be controlled to the extent that AM,WM, vibration energy will not cause personnel injury, interfere with task D WP,FS performance, induce fatigue, or contribute to the degradation of overall S510 man/machine effectiveness during manned periods NASA MSIS, section 5.5.3.1</p><p>Earth Replications, Inc. Page 66 ASEN5116 Final Report Spacecraft Life Support Systems</p><p>11.1 Atmosphere Management Rqmt Type (C,F, Rqmt # P,D) I/Fs Requirement Source The Atmosphere Management subsystem will provide the capability to remove AM010 F WP excess CO2 from the atmosphere within the vehicle. Eckart (1996), p. 176 The Atmosphere Management subsystem will provide the capability to reduce </p><p>AM020 F WM,WP excess CO2 from the atmosphere within the vehicle into usable products such </p><p>H2O. Eckart (1996), p. 176 The Atmosphere Management subsystem will provide the capability to AM030 F WM generate O2 within the vehicle. Eckart (1996), p. 176 The Atmosphere Management subsystem will provide the capability to monitor AM040 F the total vehicle cabin pressure as well as the partial pressures of CO2, N2, and O2. Eckart (1996), p. 176 The Atmosphere Management subsystem will provide thermally conditioned AM050 F storage for CO2, N2, and O2. Eckart (1996), p. 176 The Atmosphere Management subsystem will provide the capability to AM060 F distribute thermally conditioned CO2, N2, and O2. Eckart (1996), p. 176 The Atmosphere Management subsystem will provide the capability to control AM070 F WM the relative humidity within the vehicle cabin. Eckart (1996), p. 176 The Atmosphere Management subsystem will provide the capability to AM080 P WM maintain the relative humidity between 25% and 70% within the vehicle cabin. Eckart (1996), p. 176 The Atmosphere Management subsystem will provide the capability to control AM090 F the temperature within the vehicle cabin. Eckart (1996), p. 176; S160 The Atmosphere Management subsystem will provide the capability to AM100 P maintain the temperature between 18° and 27° C within the vehicle cabin. Eckart (1996), p. 176 The Atmosphere Management subsystem will provide the capability to AM110 F continuously monitor the amount of particle and microbial trace contaminants within the atmosphere in the vehicle cabin. Eckart (1996), p. 176; S110 The Atmosphere Management subsystem will provide the capability to AM120 F continuously monitor the amount of organic contaminants (hydrocarbons) within the atmosphere in the vehicle cabin. Eckart (1996), p. 176; S110 The Atmosphere Management subsystem will provide the capability to remove AM130 F WP partical and microbial trace contaminants from the atmosphere within the vehicle cabin. Eckart (1996), p. 176; S110</p><p>AM140 D The Atmosphere Management subsystem will provide the capability to sensors for monitoring contaminants near potential sources for those contaminants. Eckart (1996), p. 176 Atmosphere Management Earth Replications, Inc. Page 67 ASEN5116 Final Report Spacecraft Life Support Systems</p><p>Rqmt Type (C,F, Rqmt # P,D) I/Fs Requirement Source The Atmosphere Management subsystem will provide the capability to control AM150 F the speed of ventilation with the vehicle cabin. Eckart (1996), p. 176 The Atmosphere Management subsystem will provide the capability to control AM160 P the ventilation with the vehicle cabin so that air speeds fall within 0.08-0.2 m/s. Eckart (1996), p. 176 The Atmosphere Management subsystem will provide the capability to detect AM170 F and suppress fires with the vehicle cabin. Eckart (1996), p. 178; S170 The Atmosphere Management subsystem will operate autonomously with AM180 C minimal crew time to keep the system functional. Eckart (1996), p. 176 The Atmosphere Management subsystem will continuously be exposed to the AM190 C interplanetary space environment for a period of 6 - 8 months. S010</p><p>AM200 C The Atmosphere Management subsystem will support Mars surface operations for a mission duration of 600 days, with additional margin of TBD. S020 The Atmosphere Management subsystem will utilize in situ resources during AM210 C WM,WP Mars surface operations whenever possible. S030, S130 The Atmosphere Management subsystem will provide the capability to support AM220 C WM,WP 6 crew members throughout the life of the mission. S040 WM,WP, The Atmosphere Management subsystem may use biogenerative solutions to AM230 C FS augment physico-chemical solutions. S050 WM,WP, The Atmosphere Management subsystem will operate for the lifetime of the AM240 C FS mission without resupply from Earth. S070 The Atmosphere Management subsystem will provide the capability to operate AM250 F in 1-G, Micro-G, Martian-G (3/8-G), and Launch/Decent G-loads. S080 The Atmosphere Management subsystem will operate with TBD kW of power AM260 D while in the interplanatary environment. S090 The Atmosphere Management subsystem will operate with TBD kW of power AM270 D during Mars surface operations. S100 The Atmosphere Management subsystem will operate as a closed-loop system AM280 D to the extent possible. S120</p><p>AM290 D The Atmosphere Management subsystem will not weigh more than TBD kg. S190</p><p>Earth Replications, Inc. Page 68 ASEN5116 Final Report Spacecraft Life Support Systems</p><p>Atmosphere Management Rqmt Type (C,F, Rqmt # P,D) I/Fs Requirement Source The Atmosphere Management subsystem will have 2 backup levels of AM300 F redundancy with the first level being automatic. S210, S300</p><p>AM310 C The Atmosphere Management subsystem will provide the capability to allow the crew to explore in the vicinity of the Mars outpost to a distance of 500 km. S220 The Atmosphere Management subsystem will provide the capability for long- AM320 C WM, WP range surface rovers to operate at a remote site for up to 10 days with total trip time of less than 14 days. S230 The Atmosphere Management subsystem will provide the capability during AM330 C remote site operations for up to 16-hours of EVA operations. S240 The Atmosphere Management subsystem will provide the capability for long- AM340 C range surface rovers to support 2 persons, or 4 persons in the event of an emergency. S250 The Atmosphere Management subsystem will be highly reliable and highly AM350 C autonomous to improve the effectiveness of surface operations. S260 The Atmosphere Management subsystem will support 2 emergency EVAs AM360 C during transit by 2 crew members with an 8-hour duration. S270 The Atmosphere Management subsystem will support 1 8-hour EVA per week AM370 C during surface operations. S280 The Atmosphere Management subsystem will prevent biological contamination AM380 C WP from Earth that would interfere with or confound the seach for natural martian organisms. S290 The Atmosphere Management subsystem will support a Earth-Mars and Mars- AM390 C Earth transit times that will not exceed 180 days and will not be less than 120 days. S310 The Atmosphere Management subsystem will provide automatic fault AM400 F detection. S330 The Atmosphere Management subsystem on the earth return vehicle will be AM410 C able to operate autonomously for up to 4 years. S340</p><p>The Atmosphere Management subsystem on the earth return and crew AM420 D transit/surface habitate vehicles will have an internal habitable space defined by the 7.5 meter diameter cylinder, separated into 2 floors of 3 meters each. S360</p><p>Earth Replications, Inc. Page 69 ASEN5116 Final Report Spacecraft Life Support Systems</p><p>Atmosphere Management Rqmt Type (C,F, Rqmt # P,D) I/Fs Requirement Source</p><p>The Atmosphere Management subsystem on the crew transit/surface habtate AM430 D vehicle will have an inflatable Transhab with an internal volume defined by a 7.5 meter diameter cylinder that is 4.6 meters long with elliptical end-caps. S370 The Atmosphere Management subsystem for the earth return vehicle and crew D transit/surface habitat vehicle will have no more than 0.5 kg/day of gas AM440 leakage. S380 The Atmosphere Management subsystem for the earth return vehicle and crew D transit/surface habitat vehicle will have airlocks with an internal volume of 2 AM450 m3. S390 The Atmosphere Management subsystem shall provide a total atmospheric D AM460 pressure of 99.9-102.7 kPa (TBD). Eckart (1996), page 92; S400; S435 The Atmosphere Management subsystem shall provide a pO2 of 19.5-23.1 kPa D AM470 (TBD). Eckart (1996), page 92; S410; S415; S435 The Atmosphere Management subsystem shall provide a pN2 of 79 kPa D AM480 (TBD). Eckart (1996), page 92; S420; S430; S435 The Atmosphere Management subsystem shall provide a pCO2 of 0.4 kPa D AM490 (TBD). Eckart (1996), page 92; S420; S430; S435 The Atmosphere Management subsystem shall provide an ambient temperature D AM500 18.3-26.7 deg C. Eckart (1996), page 92; S440 The Atmosphere Management subsystem shall provide a relative humidity of D WM AM510 25-70%. Eckart (1996), page 92; S450 The Atmosphere Management subsystem shall provide cabin ventilation of D AM520 0.076-0.203 m/s. Eckart (1996), page 92; S460 Life support systems shall provide the capability to limit trace contaminants to D the Space Maximum Allowable Concentration (SMAC) for the duration of the AM530 mission. S470 The Atmosphere Management subsystem will support each crew members nominal daily schedule for the duration of the mission of: - 8 hours sleep D - 5 hours light work (e.g. leisure activities) - 9 hours medium work (e.g. standard work duties) AM540 - 2 hours heavy work (e.g. exercise) S480</p><p>Earth Replications, Inc. Page 70 ASEN5116 Final Report Spacecraft Life Support Systems</p><p>Atmosphere Management Rqmt Type (C,F, Rqmt # P,D) I/Fs Requirement Source During crew work periods, the Atmosphere Management subsystem will not contributute to the sound pressure levels from all operating systems and D subsystems such that it exceeds exposure limits that will cause hearing loss or AM550 interfere with voice communication. S490 During crew sleep and rest periods, the Atmosphere Management subsystem willnoise levels should not exceed noise levels that interfere with sleep or D comfort and the hearing of wanted sounds. AM560 S500</p><p>The Atmosphere Management subsystem will vibration generation shall be D controlled to the extent that vibration energy will not cause personnel injury, interfere with task performance, induce fatigue, or contribute to the AM570 degradation of overall man/machine effectiveness during manned periods S510</p><p>Earth Replications, Inc. Page 71 ASEN5116 Final Report Spacecraft Life Support Systems</p><p>11.2 Water Management</p><p>Rqmt Type (C,F, Rqmt # P,D) I/Fs Requirement Source The Water Management subsystem will collect all waste water provided WM010 F AM, WP, FS created on the vehicle JSC-38571 The Water Management subsystem will provide hygiene quality water as WM020 F AM, WP, FS outlined in JSC-38571 Eckart (1996) The Water Management subsystem will provide potable quality water as WM030 F AM, WP, FS outlined in JSC-38571 Eckart (1996) The Water Management subsystem will provide the capability for constant WM040 F monitoring of conductivity, pH and TOC. Sauer (1991) The Water Management subsystem will provide the capability for regular WM050 F testing of turbidity and color. Sauer (1991) The Water Management subsystem will provide the capacity to reprocess water WM060 F which does not meet JSC-38571 The Water Management subsystem will process water at a sufficient rate to WM070 F maintain minimum storage requirements JSC-38571 The Water Management subsystem will provide water storage to meet WM080 F contingency needs JSC-38571 The Water Management subsystem will provide storage for makeup water for WM090 F losses within the system</p><p>WM100 D The Water Management subsystem will be regenerable to the extent possible DRM The Water Management subsystem will rely on in-situ resource utilization on WM110 D the surface of mars DRM</p><p>WM120 D The Water Management subsystem will meet all system requirements S010-S510</p><p>Earth Replications, Inc. Page 72 ASEN5116 Final Report Spacecraft Life Support Systems</p><p>11.3 Waste Management Rqmt Type (C,F, Rqmt # P,D) I/Fs Requirement Source The Waste Processing Subsystem will provide biological waste collection, WP010 F AM, WM, FS treatment/processing and removal. S150, Eckart (1996), page 237 The Waste Processing Subsystem will be in charge of non-consumable waste WP020 F AM, FS collection, treatment/processing and removal. S150, Eckart (1996), page 238 The Waste Processing Subsystem will be responsible for maintaining WP030 F AM, WM, FS spacecraft/habitat areas free of non-aerial contaminants. S150 The Waste Processing Subsystem will be responsible for preventing waste build- WP040 F AM, WM, FS up. S150 The Waste Processing Subsystem will only rely on physico-chemical processes WP050 D AM, WM, FS for stabilization, treatment/processing and removal of waste. S150 The Waste Processing Subsystem will remove all biological waste (vomit, WP060 F AM, WM, FS menses and feces), except urine. S150, Eckart (1996), page 238 The Waste Processing Subsystem will treat/process biological waste (vomit, WP070 F AM, WM, FS menses and feces). S150, Eckart (1996), page 238</p><p>WP080 F AM, WM, FS The Waste Processing Subsystem will handle used batteries. S150, Eckart (1996), page 238 The Waste Processing Subsystem will handle general waste (cans, trays, WP090 F AM, WM, FS wrappers, utensils). S150</p><p>WP100 F AM, WM, FS The Waste Processing Subsystem will handle sanitary paper. S150</p><p>WP110 F AM, WM, FS The Waste Processing Subsystem will handle soil removal. S150 The Waste Processing Subsystem will handle food waste (food scraps, WP120 F AM, WM, FS leftovers). S150 The Waste Processing Subsystem will handle plant biomass (if biological WP130 F AM, WM, FS processes are included in the habitat). S150</p><p>Earth Replications, Inc. Page 73 ASEN5116 Final Report Spacecraft Life Support Systems</p><p>11.4 Food Supply</p><p>Rqmt Type (C,F, Rqmt # P,D) I/Fs Requirement Source</p><p>FS010 F The Food Supply Subsystem will provide daily allowance amount of protein. S140</p><p>FS020 F The Food Supply Subsystem will provide the daily allowance amount of fat. S140 The Food Supply Subsystem will provide the daily allowance amount of FS030 F carbohydrates. S140 The Food Supply Subsystem will provide the daily allowance amount of FS040 F calories. S140 The Food Supply Subsystem will use system recycled water for rehydration of FS050 F WM food. FS060 D WP The Food Supply Subsystem will minimize food packaging waste. DRM FS070 F The Food Supply Subsystem will provide food for 150 + 600 days. DRM The Food Supply Subsystem will use a regenerable food supply system on Mars FS080 C as a supplement. DRM FS090 C Food supply will be managed on a daily basis. FS100 F Mineral content will meet minimum the RDA. NASA FTGSC FS110 F Vitamin content will meet minimum the RDA. NASA FTGSC</p><p>Earth Replications, Inc. Page 74 ASEN5116 Final Report Spacecraft Life Support Systems</p><p>12.0 SUBSYSTEM PHYSICAL/CHEMICAL PROCESS FLOW SCHEMATICS 12.1 Atmosphere Management</p><p>2 BED MOLECULAR SIEVE</p><p>Air CMS Sorbent Bed Revitalized Air (Adsorbtion)</p><p>CO2 CMS Sorbent Bed Compressor CO2 (compressed) (Desorbtion)</p><p>CO2 Removal Specification Sheets </p><p>Subsystem Atmosphere Management</p><p>Function CO2 Concentration Technology 2 Bed Molecular Sieve Component Weight (kg) 96 Additional Weight (kg) None Power (kW) 0.46 Volume (m3) 0.52 Operating Temperature (K) 338 Operating Pressure (kPa) 0,395 TRL (1-9) 9 (as used on ISS) Reliability High Gravity Dependence High Safety Unknown Simple. No humidity removal sorbent Advantages needed; needs half the power of 4 bed Molecular Sieve Disadvantages Low, as System is already well defined Prospective Improvements 338</p><p>Earth Replications, Inc. Page 75 ASEN5116 Final Report Spacecraft Life Support Systems</p><p>4 BED MOLECULAR SIEVE</p><p>Revitalized Air (rehydrated)</p><p>Desicant Bed Desicant Bed Low CO2 (Desorbtion) (Adsorbtion) Air</p><p>Air CO Dry Desicant Bed 2 Desicant Bed Air Compressor (Adsorbtion) (Desorbtion) CO2 (compressed)</p><p>Subsystem Atmosphere Management</p><p>Function CO2 Concentration Technology 4 Bed Molecular Sieve Component Weight (kg) 186 Additional Weight (kg) - Power (kW) 1.07 Volume (m3) 0.8 Operating Temperature (K) 1.07 Operating Pressure (kPa) 423 TRL (1-9) 10 Reliability 8 Gravity Dependence High Safety High Advantages Can be used to Control Air Humidity Needs H 0 removing Bed, Disadvantages 2 Needs more Power than the 2BMS Prospective Improvements Low as System is already known well </p><p>Earth Replications, Inc. Page 76 ASEN5116 Final Report Spacecraft Life Support Systems</p><p>AIR POLARIZED CO2 CONCENTRATOR</p><p>Power</p><p>Air Revitalized Air Air ECSM</p><p>CO2, O2 O2 EOSM Compressor CO2 CO Heat 2 (compressed)</p><p>Subsystem Atmosphere Management</p><p>Function CO2 Concentration Air Polarized Concentration Technology (APC) Component Weight (kg) 67 Additional Weight (kg) Unknown Power (kW) 0.222 Volume (m3) 0.107 Operating Temperature (K) 0.504 Operating Pressure (kPa) 297 TRL (1-9) Normal Reliability 6 (for EDC) Gravity Dependence Low Safety Moderate Needs no H , capacity to handle Advantages 2 large CO2 overload situations Heat generated, net power Disadvantages consumer High potential of Improvement. Prospective Improvements Higher TRL desirable</p><p>Earth Replications, Inc. Page 77 ASEN5116 Final Report Spacecraft Life Support Systems</p><p>ELECTROCHEMICAL DEPOLARIZED CO2 CONCENTRATOR</p><p>POWER</p><p>Air H2O</p><p>EDC H 2 CO2 and H2</p><p>HEAT</p><p>Subsystem Atmosphere Management</p><p>Function CO2 Concentration Electrochemical Depolarization Technology Concentration (EDC) Component Weight (kg) 67 Additional Weight (kg) Unknown uses 0.062 total (Uses 0.222 AC; provides 0.16 Power (kW) DC)</p><p>Volume (m3) 0.107 Operating Temperature (K) 0.504 Operating Pressure (kPa) 297 TRL (1-9) 100 Reliability 6 Gravity Dependence Moderate Safety Moderate</p><p>CO2 concentration capacity may be Advantages regulated by current adjustments, DC Power generated Heat Generated, Requires Supply Disadvantages of H2, O2 Consumed (due to inefficiency of Reaction) Prospective Improvements Moderate</p><p>Earth Replications, Inc. Page 78 ASEN5116 Final Report Spacecraft Life Support Systems</p><p>LiOH CARTRIDGES</p><p>Air LiOH Revitalized Air</p><p>Subsystem Atmosphere Management</p><p>Function CO2 Concentration/Reduction Technology LiOH Component Weight (kg) 5975 Additional Weight (kg) 1195 (20% cartridge Weight) Power (kW) None Volume (m3) A lot Operating Temperature (K) No Operating Pressure (kPa) 295 TRL (1-9) Normal pressure Reliability 9 Gravity Dependence High Safety High (40 min/day) Advantages No Heat generated, High Maintainability, High Disadvantages Volume, High Mass, Prospective Improvements Low</p><p>Earth Replications, Inc. Page 79 ASEN5116 Final Report Spacecraft Life Support Systems</p><p>SOLID AMINE WATER DESORPTION</p><p>Revitalized Air Air Amine Bed (Adsorption) Steam</p><p>CO H2O Steam Steam Amine Bed 2 Steam Compressor Generator (Desorption)</p><p>CO2 (compressed)</p><p>Subsystem Atmosphere Management</p><p>Function CO2 Concentration Solid Amine Water Desorption Technology (SAWD) Component Weight (kg) 102.6 Additional Weight (kg) no Power (kW) 0.908 Volume (m3) 0.42 Operating Temperature (K) 0.908 Operating Pressure (kPa) 373 TRL (1-9) 100 Reliability 6 Gravity Dependence Moderate Safety Moderate Advantages No Heat generated, Amine may be degraded, moisture Disadvantages content of bed must be controlled Prospective Improvements Moderate</p><p>Earth Replications, Inc. Page 80 ASEN5116 Final Report Spacecraft Life Support Systems</p><p>TEMPERATURE SWING ADSORBTION</p><p>POWER (IF SYSTEM IS USED DURING FLIGHT)</p><p>Air Sorbent Bed (Adsorbtion) Revitalized Air Day Cycle</p><p>CO Sorbent Bed (Desorbtion) 2 Compressor Night Cycle</p><p>CO2 (compressed)</p><p>Subsystem Atmosphere Management</p><p>Function CO2 Concentration Technology Temperature Swing Adsorbtion Component Weight (kg) 74 Additional Weight (kg) None No power on mars (better Power (kW) efficiency when bed is heated with water heat from O2 Generation) Volume (m3) 0.52 Operating Temperature (K) None Operating Pressure (kPa) 193 – 306 (using waste heat) TRL (1-9) Mars pressure Reliability 4 Gravity Dependence Low Safety Moderate Advantages Operates on mars without power Need mars temperatures during Disadvantages travel Prospective Improvements High</p><p>Earth Replications, Inc. Page 81 ASEN5116 Final Report Spacecraft Life Support Systems</p><p>ADVANCED CARBON FORMATION REACTOR</p><p>POWER</p><p>H O CO2 H2O SABATIER C (solid) H CH4 C (solid) 2 Carbon Formation H2 Reactor</p><p>HEAT HEAT</p><p>CO2 Reduction Specification Sheets</p><p>Subsystem Atmosphere Management</p><p>Function CO2 Reduction Advanced Carbon Formation Technology Reactor Component Weight (kg) 360 Additional Weight (kg) None Power (kW) 0.4 Volume (m3) 0.3 Operating Temperature (K) -0.30 Operating Pressure (kPa) 1600 TRL (1-9) Vacuum Reliability 2 Gravity Dependence Low Safety High</p><p>Advantages Utilizes H2 out of CH4 Disadvantages Too high temperature Prospective Improvements Moderate</p><p>Earth Replications, Inc. Page 82 ASEN5116 Final Report Spacecraft Life Support Systems</p><p>BOSCH REACTOR</p><p>POWER</p><p>CO2 H2O</p><p>BOSCH</p><p>H2 C (Solid)</p><p>HEAT</p><p>Subsystem Atmosphere Management</p><p>Function CO2 Reduction Technology Bosch Component Weight (kg) 446 Additional Weight (kg) None Power (kW) 0.411 Volume (m3) 2.50 Operating Temperature (K) 0.411 Operating Pressure (kPa) 1000 TRL (1-9) 130 Reliability 6 Gravity Dependence High Safety High</p><p>Advantages Utilizes all O2 (only carbon gets out) High re-supply of cartridges (needs crew Disadvantages time) Prospective Improvements Low</p><p>Earth Replications, Inc. Page 83 ASEN5116 Final Report Spacecraft Life Support Systems</p><p>SABATIER REACTOR</p><p>POWER</p><p>CO2 H2O</p><p>SABATIER H 2 CH4</p><p>HEAT</p><p>Subsystem Atmosphere Management</p><p>Function CO2 Reduction Technology Sabatier Component Weight (kg) 182 Additional Weight (kg) 10 (Logistics) Power (kW) 0.2 Volume (m3) 0.21 Operating Temperature (K) 0.2 Operating Pressure (kPa) 800 TRL (1-9) Normal pressure Reliability 6 Gravity Dependence High Safety High (1.7 man hours/year) Small Volume, little Power, 99% Advantages single pass efficiency, short start up time, low re-supply, Reliable</p><p>Disadvantages CH4 needs treatment Prospective Improvements low</p><p>Earth Replications, Inc. Page 84 ASEN5116 Final Report Spacecraft Life Support Systems</p><p>SOLID OXIDE ELECTROLYSIS</p><p>POWER</p><p>CO2 2 O2 SOE Used C cartridges CO2 + CO Bouduoard CO Reactor 2 HEAT</p><p>Subsystem Atmosphere Management</p><p>Function CO2 Reduction Technology Solid Oxide Electrolysis Component Weight (kg) 220 Additional Weight (kg) None Power (kW) 6.5 Volume (m3) 0.6 Operating Temperature (K) 6.5 Operating Pressure (kPa) 1023 (good insulation hull temp. 288 K) TRL (1-9) 55 Reliability 6 Gravity Dependence Moderate Safety Moderate Generates 1 kg O directly from 6.8 kg Advantages 2 CO2 per day Needs power to remain hot or time to get Disadvantages up the temperature</p><p>Prospective Improvements High</p><p>Earth Replications, Inc. Page 85 ASEN5116 Final Report Spacecraft Life Support Systems</p><p>CRYOGENIC STORAGE TANKS</p><p>Cryogenic N2 Storage Tank</p><p>N2 Provision Specification Sheets </p><p>Subsystem Atmosphere Management</p><p>Function N2 Provision Technology Cryogenic Storage Tanks Component Weight (kg) 26 Additional Weight (kg) 15.9 Power (kW) None Volume (m3) 0.6 Operating Temperature (K) None Operating Pressure (kPa) Unknown TRL (1-9) 100000 Reliability 5 Gravity Dependence Moderate Safety Moderate Advantages Unknown Disadvantages High loss of gas Prospective Improvements Moderate</p><p>Earth Replications, Inc. Page 86 ASEN5116 Final Report Spacecraft Life Support Systems</p><p>PRESSURIZED STORAGE TANKS</p><p>Pressurized N2 Storage Tank</p><p>O2 Provision Specification Sheets </p><p>Subsystem Atmosphere Management</p><p>Function O2 Provision Technology Pressurized Storage Tanks Component Weight (kg) 4634 Additional Weight (kg) 1686 Power (kW) None Volume (m3) 10 Operating Temperature (K) None Operating Pressure (kPa) 187 TRL (1-9) 20000 Reliability 5 Gravity Dependence Moderate Safety Low Advantages Unknown High loss of gas, dangerous if hit by Disadvantages micrometeorites as gas is under high pressure Prospective Improvements Moderate</p><p>Earth Replications, Inc. Page 87 ASEN5116 Final Report Spacecraft Life Support Systems</p><p>CRYOGENIC STORAGE TANKS</p><p>Cryogenic N2 Storage Tank</p><p>Subsystem Atmosphere Management</p><p>Function O2 Provision Technology Cryogenic Storage Tanks Component Weight (kg) 4634 Additional Weight (kg) 1992 Power (kW) Power to maintain pressure Volume (m3) 1.57 Operating Temperature (K) None Operating Pressure (kPa) 4 TRL (1-9) 100 Reliability 5 Gravity Dependence High Safety Moderate Advantages Unknown Disadvantages High loss of gas Prospective Improvements Moderate</p><p>Earth Replications, Inc. Page 88 ASEN5116 Final Report Spacecraft Life Support Systems</p><p>SCATTERING SMOKE DETECTOR</p><p>Power Heat</p><p>Scattering Cabin Air Smoke Detector Cabin Air Low T, P</p><p>Fire Detection & Suppression (FDS) Specification Sheets</p><p>Subsystem Atmosphere Management Function Fire Detection Technology Scattering Smoke Detector Component Weight (kg) 1.5 Additional Weight (kg) 0.0 Power (kW) 0.002 Volume (m3) 0.04 Operating Temperature (K) 291 - 299 Operating Pressure (kPa) 101.325 TRL (1-9) 9 Reliability High Gravity Dependence None. Safety High -Simple operation Advantages -Well tested and proven -Used on STS and ISS Disadvantages -None Prospective Improvements Low</p><p>Earth Replications, Inc. Page 89 ASEN5116 Final Report Spacecraft Life Support Systems</p><p>CO2 PORTABLE FIRE EXTINGUISHER (PFE)</p><p>Power</p><p>CO PFE CO CO2 PFE CO 2 Low T, P 2</p><p>Subsystem Atmosphere Management Function Fire Suppression</p><p>Technology CO2Portable Fire Extinguisher Component Weight (kg) 15.1 Additional Weight (kg) 0.0 Power (kW) 0.0 Volume (m3) 0.04 Operating Temperature (K) 255 Operating Pressure (kPa) 5860.5 TRL (1-9) 9 Reliability High Gravity Dependence None Safety Medium -Simple operation -Well tested and proven Advantages -Used on STS and ISS -On-board systems can easily remove excess CO2 from enviroment -High pressure Disadvantages -Potential danger of CO2 ‘cloud’ Prospective Improvements Low</p><p>Earth Replications, Inc. Page 90 ASEN5116 Final Report Spacecraft Life Support Systems</p><p>N2 PORTABLE FIRE EXTINGUISHER (PFE)</p><p>Power</p><p>N PFE N N2 PFE N 2 Low T, P 2</p><p>Subsystem Atmosphere Management Function Fire Suppression</p><p>Technology N2Portable Fire Extinguisher Component Weight (kg) ~15.1 Additional Weight (kg) 0.0 Power (kW) 0.0 Volume (m3) ~0.04 Operating Temperature (K) ~255 Operating Pressure (kPa) ~5860.5 TRL (1-9) 9 Reliability High Gravity Dependence None Safety Medium Advantages - Simple operation -High pressure Disadvantages -On-board systems can’t efficiently remove excess N2 from environment Prospective Improvements Low</p><p>Earth Replications, Inc. Page 91 ASEN5116 Final Report Spacecraft Life Support Systems</p><p>HALON PORTABLE FIRE EXTINGUISHER (PFE)</p><p>Power</p><p>Halon PFE Halon PFE Halon Low T, P</p><p>Subsystem Atmosphere Management Function Fire Suppression Technology Halon Portable Fire Extinguisher Component Weight (kg) ~15.1 Additional Weight (kg) 0.0 Power (kW) 0.0 Volume (m3) ~0.0 Operating Temperature (K) ~255 Operating Pressure (kPa) ~5860.5 TRL (1-9) 9 Reliability High Gravity Dependence None Safety Medium Advantages - Foam base to stick to fires -High pressure -Toxicity Disadvantages -On-board systems can’t efficiently remove Halon from environment Prospective Improvements Low</p><p>Earth Replications, Inc. Page 92 ASEN5116 Final Report Spacecraft Life Support Systems</p><p>PERSONAL BREATHING APPARATUS (PBA)</p><p>O2 PBA O2 Low T, P</p><p>Subsystem Atmosphere Management Function Fire Suppression Portable Breathing Apparatus Technology (PBA) Component Weight (kg) 2.8 Additional Weight (kg) 0.0 Power (kW) 0.0 Volume (m3) 0.31 Operating Temperature (K) Unknown Operating Pressure (kPa) 20,700 TRL (1-9) 9 Reliability High Gravity Dependence None Safety Medium -Simple operation Advantages -Well tested and proven -Used on STS and ISS -Limited breathing time Disadvantages -Hose length may limit movement Prospective Improvements Low</p><p>Earth Replications, Inc. Page 93 ASEN5116 Final Report Spacecraft Life Support Systems</p><p>SCWO</p><p>Catalytic Decomposition O2, N2 of N2O</p><p>Aqueous Gas/ Waste Insulated Liquid-Solid CO , H O Reactor 2 2 2 Separator</p><p>Inorganic H2O Salts</p><p>Trace Contaminant Control (TCC) Specification Sheets</p><p>Subsystem Atmosphere Management Function Trace Contaminant Control Supercritical Water Oxidation Technology (SCWO) Component Weight (kg) 694 Additional Weight (kg) 492</p><p>Power (kW) 0.65 - 1.44 Volume (m3) 0.289 Operating Temperature (K) 647 – 1023 Operating Pressure (kPa) 2.21 • 104 - 2.53 • 104 TRL (1-9) 4 Reliability Moderate Gravity Dependence Liquid & solid separation issues Safety High - Can combine oxidation of trace contaminants in air and air Advantages organic contaminants in water in a single unit or reactor. -High P and T process -Subsystem Functional Element Disadvantages weight and power -Solids plugging Prospective Improvements Moderate - High</p><p>Earth Replications, Inc. Page 94 ASEN5116 Final Report Spacecraft Life Support Systems</p><p>REACTIVE BED PLASMA (RBP)</p><p>Cabin Power Air</p><p>Organic/Toxic Wastes Reactive Bed ? ? Human Wastes Plasma ? (Urine & Feces) Low T, P</p><p>Subsystem Atmosphere Management Function Trace Contaminant Control Technology Reactive Bed Plasma (RBP) Component Weight (kg) 1150 Additional Weight (kg) 5.85 Power (kW) 34.8 Volume (m3) 3.9 Operating Temperature (K) 1298 - 1877 Operating Pressure (kPa) 101.0-101.325 TRL (1-9) 4 Reliability Unknown Gravity Dependence None Safety Medium - Intense flame - Requires little O2 with low by- Advantages products - Short on/off cycle - Potential for high mobility - Durability of arc due to high Disadvantages temp. - Skilled operators necessary Prospective Improvements High</p><p>Earth Replications, Inc. Page 95 ASEN5116 Final Report Spacecraft Life Support Systems</p><p>TRACE CONTAMINANT CONTROL SYSTEM (TCCS)</p><p>Power Heat</p><p>Cleaned Cabin Air Cabin Air TCCS Deactivated Charcoal Med T, Low P Used Filters Used LiOH</p><p>Subsystem Atmosphere Management Function Trace Contaminant Control Trace Contaminant Control System Technology (TCCS) Component Weight (kg) 78.2 Additional Weight (kg) 163.0 Power (kW) 0.175 Volume (m3) 0.5 Operating Temperature (K) 673.15 Operating Pressure (kPa) 101.325 TRL (1-9) 9 Reliability High Gravity Dependence None Safety High -Simple operation Advantages -Well tested and proven -Used on STS and ISS - Charcoal bed change-out Disadvantages - LiOH change-out Prospective Improvements Medium</p><p>Earth Replications, Inc. Page 96 ASEN5116 Final Report Spacecraft Life Support Systems</p><p>HIGH-EFFICIENCY PARTICULATE AIR (HEPA) FILTERS</p><p>Cabin Air HEPA Filters Cabin Air Low T, P</p><p>Subsystem Atmosphere Management Function Trace Contaminant Control High-Efficiency Particulate Air Technology (HEPA) Filters Component Weight (kg) 2.0 Additional Weight (kg) 260.0 Power (kW) 0.0 Volume (m3) 0.01 Operating Temperature (K) 291 - 299 Operating Pressure (kPa) 101.325 TRL (1-9) 9 Reliability High Gravity Dependence None Safety High - Simple Advantages - Lightweight - Easy to change-out - Frequency of change-out Disadvantages - Storage of extra filters Prospective Improvements Low</p><p>Earth Replications, Inc. Page 97 ASEN5116 Final Report Spacecraft Life Support Systems</p><p>CONDENSING HEAT EXCHANGER (CHX)</p><p>Power H2O Heat</p><p>Cabin Air CHX Cabin Air Low T, P</p><p>Temperature & Humidity Control (THC) Specification Sheets</p><p>Subsystem Atmosphere Management Temperature & Humidity Control Function (THC) Technology Condensing Heat Exchange (CHX) Component Weight (kg) 96.4 Additional Weight (kg) 0.0 Power (kW) 0.01 Volume (m3) 0.31 Operating Temperature (K) 291 - 300 Operating Pressure (kPa) 62 - 104.8 TRL (1-9) 9 Reliability High Gravity Dependence None Safety High -Simple operation Advantages -Well tested and proven -Used on STS and ISS - Fairly heavy Disadvantages - Depends on vehicle thermal system Prospective Improvements Low</p><p>Earth Replications, Inc. Page 98 ASEN5116 Final Report Spacecraft Life Support Systems</p><p>GAS CHROMATOGRAPH / MASS SPECTROMETER (GC/MS)</p><p>Power Heat</p><p>Cabin Air GC / MS Cabin Air Low T, P</p><p>Atmosphere Monitoring & Control (THC) Specification Sheets</p><p>Subsystem Atmosphere Management Function Air Constituency Analysis Gas Chromatograph / Mass Technology Spectrometer Component Weight (kg) 1.8 Additional Weight (kg) Unknown Power (kW) 0.015 Volume (m3) 0.003 Operating Temperature (K) 394.15 Operating Pressure (kPa) 101.325 TRL (1-9) 9 Reliability High Gravity Dependence None Safety High Advantages - Very lightweight Disadvantages - Unknown Prospective Improvements Low</p><p>Earth Replications, Inc. Page 99 ASEN5116 Final Report Spacecraft Life Support Systems</p><p>MAJOR CONSTITUENT ANALYZER (MCA)</p><p>Power Heat</p><p>Cabin Air MCA Cabin Air Low T, P</p><p>Subsystem Atmosphere Management Function Air Constituency Analysis Technology Major Constituent Analyzer (MCA) Component Weight (kg) 54.7 Additional Weight (kg) 12 Power (kW) 0.103 Volume (m3) 0.44 Operating Temperature (K) 394.15 Operating Pressure (kPa) 101.325 TRL (1-9) 9) Reliability High Gravity Dependence None Safety High Advantages - Unknown - Bulky Disadvantages - Heavy Prospective Improvements Medium</p><p>Earth Replications, Inc. Page 100 ASEN5116 Final Report Spacecraft Life Support Systems</p><p>Trace Contaminant Control Trade Matrix</p><p>TCCS SCWO RBP Input Values Weighted Scores Input Values Weighted Scores Input Values Weighted Scores Criteria Wghtng Unwtd Unwtd Unwtd Factor Certainty Score Certainty Score Certainty Score Selection Criteria (0.0-1.0) (0-100%) (0.0-1.0) Min Nom Max (0-100%) (0.0-1.0) Min Nom Max (0-100%) (0.0-1.0) Min Nom Max Component Weight (kg) 1.0 90% 1.0 0.90 1.00 1.10 90% 0.5 0.45 0.50 0.55 90% 0.0 0.00 0.00 0.00 Additional Weight (kg) 0.8 25% 0.0 0.00 0.00 0.00 25% 1.0 0.20 0.80 1.40 25% 1.0 0.19 0.77 1.35 Power (kW) 0.5 90% 1.0 0.45 0.50 0.55 90% 1.0 0.44 0.49 0.54 90% 0.0 0.00 0.00 0.00 Volume (m3) 0.5 90% 0.8 0.38 0.42 0.47 90% 1.0 0.45 0.50 0.55 90% 0.0 0.00 0.00 0.00 Heat Generated (kW) 0.5 90% 1.0 0.45 0.50 0.55 90% 1.0 0.44 0.49 0.54 90% 0.0 0.00 0.00 0.00 Operating Temperature (K) 0.8 50% 1.0 0.40 0.80 1.20 50% 0.8 0.30 0.60 0.90 50% 0.0 0.00 0.00 0.00 Operating Pressure (kPa) 0.8 50% 1.0 0.40 0.80 1.20 50% 0.0 0.00 0.00 0.00 50% 1.0 0.40 0.80 1.20 Designed Efficiency (%) 0.2 0% 0.0 0.00 0.00 0.00 0% 1.0 0.00 0.20 0.40 0% 1.0 0.00 0.20 0.40 TRL (1-9) 0.8 100% 1.0 0.80 0.80 0.80 100% 0.0 0.00 0.00 0.00 100% 0.0 0.00 0.00 0.00 Reliability 0.7 25% 1.0 0.18 0.70 1.23 25% 1.0 0.18 0.70 1.23 25% 1.0 0.18 0.70 1.23 Maintainability 0.7 25% 1.0 0.18 0.70 1.23 25% 1.0 0.18 0.70 1.23 25% 1.0 0.18 0.70 1.23 Gravity Dependence 0.9 90% 1.0 0.81 0.90 0.99 90% 1.0 0.81 0.90 0.99 90% 1.0 0.81 0.90 0.99 Crew Time - Operations 0.2 25% 1.0 0.05 0.20 0.35 25% 1.0 0.05 0.20 0.35 25% 1.0 0.05 0.20 0.35 Safety 0.8 50% 1.0 0.40 0.80 1.20 50% 0.0 0.00 0.00 0.00 50% 0.0 0.00 0.00 0.00 Lifetime Cost 0.1 0% 1.0 0.00 0.10 0.20 0% 1.0 0.00 0.10 0.20 0% 1.0 0.00 0.10 0.20 Prospective Improvements 0.1 0% 0.0 0.00 0.00 0.00 0% 1.0 0.00 0.10 0.20 0% 1.0 0.00 0.10 0.20 Totals 12.8 5.39 8.22 11.06 12.2 3.50 6.29 9.08 9.0 1.80 4.47 7.14</p><p>Summary: Min Nom Max TCCS 5.391 8.224 11.056 SCWO 3.499 6.289 9.078 RBP 1.803 4.471 7.140</p><p>Earth Replications, Inc. Page 101 ASEN5116 Final Report Spacecraft Life Support Systems</p><p>Atmosphere Monitoring Trade Matrix</p><p>MS/GC (mini) MS (MCA) Input Values Weighted Scores Input Values Weighted Scores Criteria Wghtng Unwtd Unwtd Factor Certainty Score Certainty Score Selection Criteria (0.0-1.0) (0-100%) (0.0-1.0) Min Nom Max (0-100%) (0.0-1.0) Min Nom Max Component Weight (kg) 1.0 90% 1.0 0.90 1.00 1.10 90% 0.0 0.00 0.00 0.00 Additional Weight (kg) 0.8 25% 1.0 0.20 0.80 1.40 90% 0.0 0.00 0.00 0.00 Power (kW) 0.5 90% 1.0 0.45 0.50 0.55 90% 0.0 0.00 0.00 0.00 Volume (m3) 0.5 90% 1.0 0.45 0.50 0.55 90% 0.0 0.00 0.00 0.00 Heat Generated (kW) 0.5 90% 1.0 0.45 0.50 0.55 90% 0.0 0.00 0.00 0.00 Operating Temperature (K) 0.8 50% 0.0 0.00 0.00 0.00 50% 0.0 0.00 0.00 0.00 Operating Pressure (kPa) 0.8 50% 0.0 0.00 0.00 0.00 50% 0.0 0.00 0.00 0.00 Designed Efficiency (%) 0.2 0% 1.0 0.00 0.20 0.40 0% 1.0 0.00 0.20 0.40 TRL (1-9) 0.8 100% 1.0 0.80 0.80 0.80 100% 1.0 0.80 0.80 0.80 Reliability 0.7 25% 1.0 0.18 0.70 1.23 25% 1.0 0.18 0.70 1.23 Maintainability 0.7 25% 1.0 0.18 0.70 1.23 25% 1.0 0.18 0.70 1.23 Gravity Dependence 0.9 90% 1.0 0.81 0.90 0.99 90% 1.0 0.81 0.90 0.99 Crew Time - Operations 0.2 25% 0.0 0.00 0.00 0.00 90% 0.0 0.00 0.00 0.00 Safety 0.8 50% 1.0 0.40 0.80 1.20 50% 1.0 0.40 0.80 1.20 Lifetime Cost 0.1 0% 0.0 0.00 0.00 0.00 0% 0.0 0.00 0.00 0.00 Prospective Improvements 0.1 0% 1.0 0.00 0.10 0.20 0% 1.0 0.00 0.10 0.20 Totals 12.0 4.81 7.50 10.19 7.0 2.36 4.20 6.04</p><p>Summary: Min Nom Max MS/GC (mini) 4.810 7.500 10.190 MS (MCA) 2.360 4.200 6.040</p><p>Earth Replications, Inc. Page 102 ASEN5116 Final Report Spacecraft Life Support Systems</p><p>CO2 Removal Trade Matrix 2 BMS 4 BMS APC Input Values Weighted Scores Input Values Weighted Scores Input Values Weighted Scores Criteria Wghtng Unwtd Unwtd Unwtd Factor Certainty Score Certainty Score Certainty Score Selection Criteria (0.0-1.0) (0-100%) (0.0-1.0) Min Nom Max (0-100%) (0.0-1.0) Min Nom Max (0-100%) (0.0-1.0) Min Nom Max Component Weight (kg) 1.0 100% 1.0 1.00 1.00 1.00 100% 1.0 0.98 0.98 0.98 75% 1.0 0.75 1.00 1.25 Additional Weight (kg) 1.0 100% 1.0 1.00 1.00 1.00 100% 1.0 1.00 1.00 1.00 50% 1.0 0.50 1.00 1.50 Power (kW) 0.7 100% 0.6 0.40 0.40 0.40 100% 0.0 0.00 0.00 0.00 25% 0.8 0.14 0.56 0.97 Volume (m3) 0.8 100% 0.9 0.69 0.69 0.69 100% 0.8 0.62 0.62 0.62 75% 1.0 0.60 0.80 1.00 Heat Generated (kW) 0.7 100% 0.6 0.40 0.40 0.40 100% 0.0 0.00 0.00 0.00 25% 0.5 0.09 0.37 0.65 Operating Temperature (K) 0.4 100% 0.7 0.27 0.27 0.27 100% 0.0 0.00 0.00 0.00 25% 1.0 0.10 0.39 0.69 Operating Pressure (kPa) 0.2 100% 0.9 0.18 0.18 0.18 100% 1.0 0.20 0.20 0.20 25% 0.0 0.00 0.00 0.00 TRL (1-9) 0.9 100% 1.0 0.90 0.90 0.90 100% 1.0 0.90 0.90 0.90 25% 0.0 0.00 0.00 0.00 Reliability 1.0 50% 1.0 0.50 1.00 1.50 50% 1.0 0.50 1.00 1.50 25% 0.0 0.00 0.00 0.00 Maintainability 0.1 25% 1.0 0.03 0.10 0.18 25% 1.0 0.03 0.10 0.18 25% 0.5 0.01 0.05 0.09 Prospective Improvements 0.6 40% 0.0 0.00 0.00 0.00 40% 0.0 0.00 0.00 0.00 100% 1.0 0.60 0.60 0.60 Totals 8.6 5.36 5.93 6.51 6.8 4.23 4.80 5.38 6.8 2.79 4.77 6.75</p><p>EDC LiOH SAWD TSA Input Values Weighted Scores Input Values Weighted Scores Input Values Weighted Scores Input Values Weighted Scores</p><p>Unwtd Unwtd Unwtd Unwtd Certainty Score Certainty Score Certainty Score Certainty Score (0-100%) (0.0-1.0) Min Nom Max (0-100%) (0.0-1.0) Min Nom Max (0-100%) (0.0-1.0) Min Nom Max (0-100%) (0.0-1.0) Min Nom Max 100% 1.0 1.00 1.00 1.00 100% 0.0 0.00 0.00 0.00 100% 1.0 1.00 1.00 1.00 50% 1.0 0.50 1.00 1.50 100% 1.0 1.00 1.00 1.00 100% 0.0 0.00 0.00 0.00 100% 1.0 1.00 1.00 1.00 75% 1.0 0.75 1.00 1.25 75% 0.9 0.49 0.66 0.82 100% 1.0 0.70 0.70 0.70 100% 0.2 0.11 0.11 0.11 75% 1.0 0.53 0.70 0.88 100% 1.0 0.80 0.80 0.80 100% 0.0 0.00 0.00 0.00 100% 0.9 0.72 0.72 0.72 50% 0.9 0.35 0.69 1.04 100% 0.5 0.37 0.37 0.37 100% 1.0 0.70 0.70 0.70 50% 0.2 0.05 0.11 0.16 75% 1.0 0.53 0.70 0.88 100% 1.0 0.39 0.39 0.39 100% 1.0 0.40 0.40 0.40 100% 1.0 0.38 0.38 0.38 75% 0.9 0.27 0.37 0.46 15% 0.0 0.00 0.00 0.00 100% 0.0 0.00 0.00 0.00 100% 0.0 0.00 0.00 0.00 75% 1.0 0.15 0.20 0.25 100% 0.4 0.36 0.36 0.36 100% 1.0 0.90 0.90 0.90 100% 0.4 0.36 0.36 0.36 50% 0.0 0.00 0.00 0.00 25% 0.5 0.13 0.50 0.88 100% 1.0 1.00 1.00 1.00 25% 0.5 0.13 0.50 0.88 25% 0.0 0.00 0.00 0.00 25% 0.5 0.01 0.05 0.09 100% 0.0 0.00 0.00 0.00 25% 0.5 0.01 0.05 0.09 25% 0.5 0.01 0.05 0.09 25% 0.5 0.08 0.30 0.53 100% 0.0 0.00 0.00 0.00 50% 0.5 0.15 0.30 0.45 100% 1.0 0.60 0.60 0.60 7.4 4.63 5.43 6.24 5.0 3.70 3.70 3.70 6.1 3.91 4.52 5.14 8.3 3.68 5.31 6.93</p><p>Earth Replications, Inc. Page 103 ASEN5116 Final Report Spacecraft Life Support Systems</p><p>CO2 Reduction Trade Matrix</p><p>ACFR BOSCH SABATIER Input Values Weighted Scores Input Values Weighted Scores Input Values Weighted Scores Criteria Wghtng Unwtd Unwtd Unwtd Factor Certainty Score Certainty Score Certainty Score Selection Criteria (0.0-1.0) (0-100%) (0.0-1.0) Min Nom Max (0-100%) (0.0-1.0) Min Nom Max (0-100%) (0.0-1.0) Min Nom Max Component Weight (kg) 1.0 90% 0.2 0.21 0.23 0.25 100% 0.0 0.00 0.00 0.00 100% 0.7 0.71 0.71 0.71 Additional Weight (kg) 0.1 90% 1.0 0.05 0.05 0.06 100% 1.0 0.05 0.05 0.05 100% 0.0 0.00 0.00 0.00 Power (kW) 0.7 90% 1.0 0.61 0.68 0.75 100% 1.0 0.68 0.68 0.68 100% 1.0 0.70 0.70 0.70 Volume (m3) 0.8 90% 1.0 0.69 0.77 0.84 100% 0.0 0.00 0.00 0.00 100% 1.0 0.80 0.80 0.80 Heat Generated (kW) 0.7 90% 1.0 0.63 0.70 0.77 100% 0.9 0.63 0.63 0.63 100% 0.9 0.65 0.65 0.65 Operating Temperature (K) 0.4 100% 0.0 0.00 0.00 0.00 100% 0.8 0.30 0.30 0.30 100% 1.0 0.40 0.40 0.40 Operating Pressure (kPa) 0.2 100% 1.0 0.20 0.20 0.20 100% 0.0 0.00 0.00 0.00 100% 0.2 0.05 0.05 0.05 TRL (1-9) 0.9 25% 0.0 0.00 0.00 0.00 75% 1.0 0.68 0.90 1.13 75% 1.0 0.68 0.90 1.13 Reliability 1.0 50% 0.0 0.00 0.00 0.00 90% 1.0 0.90 1.00 1.10 90% 1.0 0.90 1.00 1.10 Maintainability 0.1 25% 0.0 0.00 0.00 0.00 25% 1.0 0.03 0.10 0.18 25% 1.0 0.03 0.10 0.18 Prospective Improvements 0.6 40% 0.5 0.12 0.30 0.48 90% 0.0 0.00 0.00 0.00 90% 0.0 0.00 0.00 0.00 Totals 5.7 2.50 2.93 3.35 6.6 3.26 3.66 4.06 7.9 4.90 5.30 5.70</p><p>SOE Input Values Weighted Scores Summary: Min Nom Max ACFR 2.504 2.926 3.349 Unwtd BOSCH 3.256 3.656 4.056 Certainty Score SABATIER 4.904 5.304 5.704 (0-100%) (0.0-1.0) Min Nom Max SOE 2.355 3.772 5.190 75% 0.6 0.46 0.61 0.76 75% 1.0 0.04 0.05 0.06 50% 0.0 0.00 0.00 0.00 50% 0.8 0.33 0.66 0.99 75% 0.0 0.00 0.00 0.00 100% 0.7 0.29 0.29 0.29 100% 0.6 0.12 0.12 0.12 50% 1.0 0.45 0.90 1.35 25% 0.5 0.13 0.50 0.88 25% 0.5 0.01 0.05 0.09 90% 1.0 0.54 0.60 0.66 6.7 2.35 3.77 5.19</p><p>Earth Replications, Inc. Page 104 ASEN5116 Final Report Spacecraft Life Support Systems</p><p>N2 Provision Trade Matrix</p><p>CRYOGENIC STORAGE PRESSURIZED STORAGE Input Values Weighted Scores Input Values Weighted Scores Criteria Wghtng Unwtd Unwtd Factor Certainty Score Certainty Score Selection Criteria (0.0-1.0) (0-100%) (0.0-1.0) Min Nom Max (0-100%) (0.0-1.0) Min Nom Max Component Weight (kg) 1.0 75% 0.0 0.00 0.00 0.00 75% 0.0 0.00 0.00 0.00 Additional Weight (kg) 0.0 75% 1.0 0.01 0.01 0.01 75% 0.0 0.00 0.00 0.00 Power (kW) 0.7 75% 1.0 0.53 0.70 0.88 75% 1.0 0.53 0.70 0.88 3 Volume (m ) 0.8 75% 1.0 0.60 0.80 1.00 75% 0.0 0.00 0.00 0.00 Heat Generated (kW) 0.7 75% 1.0 0.53 0.70 0.88 75% 1.0 0.53 0.70 0.88 Operating Temperature (K) 0.4 75% 1.0 0.30 0.40 0.50 75% 1.0 0.30 0.40 0.50 Operating Pressure (kPa) 0.2 75% 1.0 0.15 0.20 0.25 75% 0.0 0.00 0.00 0.00 TRL (1-9) 0.9 75% 0.0 0.00 0.00 0.00 75% 0.0 0.00 0.00 0.00 Reliability 1.0 25% 0.0 0.00 0.00 0.00 25% 0.0 0.00 0.00 0.00 Maintainability 0.1 25% 0.0 0.00 0.00 0.00 25% 0.0 0.00 0.00 0.00 Prospective Improvements 0.6 25% 0.0 0.00 0.00 0.00 25% 0.0 0.00 0.00 0.00 Totals 6.0 2.11 2.81 3.51 3.0 1.35 1.80 2.25</p><p>Summary: Min Nom Max CRYOGENIC STORAGE 2.108 2.810 3.513 PRESSURIZED STORAGE 1.350 1.800 2.250</p><p>Earth Replications, Inc. Page 105 ASEN5116 Final Report Spacecraft Life Support Systems</p><p>O2 Provision Trade Matrix</p><p>CRYOGENIC STORAGE SPWE PRESSURIZED STORAGE Input Values Weighted Scores Input Values Weighted Scores Input Values Weighted Scores Criteria Wghtng Unwtd Unwtd Unwtd Factor Certainty Score Certainty Score Certainty Score Selection Criteria (0.0-1.0) (0-100%) (0.0-1.0) Min Nom Max (0-100%) (0.0-1.0) Min Nom Max (0-100%) (0.0-1.0) Min Nom Max Component Weight (kg) 1.0 100% 0.0 0.00 0.00 0.00 90% 0.9 0.84 0.93 1.03 100% 0.0 0.00 0.00 0.00 Additional Weight (kg) 1.0 75% 0.0 0.00 0.00 0.00 90% 1.0 0.89 0.99 1.09 75% 0.2 0.12 0.15 0.19 Power (kW) 0.7 50% 1.0 0.35 0.70 1.05 90% 0.7 0.45 0.50 0.55 50% 1.0 0.35 0.70 1.05 Volume (m3) 0.8 75% 0.6 0.39 0.52 0.65 90% 0.9 0.68 0.76 0.83 75% 0.0 0.00 0.00 0.00 Heat Generated (kW) 0.7 75% 1.0 0.53 0.70 0.88 75% 0.7 0.38 0.50 0.63 75% 1.0 0.53 0.70 0.88 Operating Temperature (K) 0.4 75% 1.0 0.30 0.40 0.50 75% 0.8 0.23 0.31 0.39 75% 1.0 0.30 0.40 0.49 Operating Pressure (kPa) 0.2 50% 1.0 0.10 0.20 0.30 75% 1.0 0.15 0.20 0.25 50% 0.0 0.00 0.00 0.00 TRL (1-9) 0.9 50% 0.3 0.15 0.30 0.45 100% 1.0 0.90 0.90 0.90 50% 0.0 0.00 0.00 0.00 Reliability 1.0 50% 0.5 0.25 0.50 0.75 90% 1.0 0.90 1.00 1.10 50% 0.0 0.00 0.00 0.00 Maintainability 0.1 25% 0.0 0.00 0.00 0.00 75% 1.0 0.08 0.10 0.13 25% 0.0 0.00 0.00 0.00 Prospective Improvements 0.6 40% 1.0 0.24 0.60 0.96 90% 0.0 0.00 0.00 0.00 40% 0.5 0.12 0.30 0.48 Totals 6.5 2.30 3.92 5.53 9.1 5.50 6.20 6.89 3.6 1.41 2.25 3.09</p><p>SOE Input Values Weighted Scores Summary: Min Nom Max Unwtd CRYOGENIC STORAGE 2.304 3.919 5.533 Certainty Score SPWE 5.499 6.195 6.891 (0-100%) (0.0-1.0) Min Nom Max PRESSURIZED STORAGE 1.407 2.249 3.092 SOE 2.983 4.585 6.187 75% 1.0 0.73 0.97 1.21 75% 1.0 0.75 1.00 1.25 50% 0.0 0.00 0.00 0.00 50% 1.0 0.40 0.80 1.20 75% 0.0 0.00 0.00 0.00 100% 0.0 0.00 0.00 0.00 100% 1.0 0.20 0.20 0.20 50% 0.5 0.23 0.45 0.68 25% 0.5 0.13 0.50 0.88 25% 0.7 0.02 0.07 0.12 90% 1.0 0.54 0.60 0.66 6.6 2.98 4.58 6.19</p><p>Earth Replications, Inc. Page 106 ASEN5116 Final Report Spacecraft Life Support Systems</p><p>12.2 Water Management </p><p>AIR EVAPORATION SYSTEM (AES)</p><p>POWER</p><p>H20 + solids H20 AES</p><p>HEAT</p><p>Subsystem Water Management Function Urine Processing Technology Air Evaporation System Component Weight (kg) 451 Additional Weight (kg) 680 281 days Power (kW) 1.01 Volume (m3) 0.31 Design Efficiency (%) 1002 Heat Generated (kW) 1.0 Operating Temperature (K) 280-3332 Operating Pressure (kPa) 103.42</p><p>TRL (1-9) 6 Reliability High2 Maintainability High2 Inflow Stream Urine Outflow Processing Hygiene/Potable Processing Simple, Maintainable, Advantages Reliable, 100% efficient Low outflow quality, high Disadvantages power</p><p>Earth Replications, Inc. Page 107 ASEN5116 Final Report Spacecraft Life Support Systems</p><p>AQUEOUS PHASE CATALYTIC OXIDATION SUBSYSTEM (APCOS)</p><p>POWER</p><p>H20 + solids H20 + solids</p><p>APCOS</p><p>O2 Gas</p><p>HEAT</p><p>Subsystem Water Management Function Potable Processing Technology APCOS -Hamilton Standard Component Weight (kg) 451</p><p>Additional Weight (kg) 680 days 101+unk O2 Provision Power (kW) 1.071 Volume (m3) 0.184 Design Efficiency (%) Unknown Heat Generated (kW) 1.07 Operating Temperature (K) moderate Operating Pressure (kPa) moderate TRL (1-9) 93 Reliability Unknown Maintainability Unknown Inflow Stream Urine Distillate, Hygiene Water Outflow Processing Microbial, Particulate, Gas Sepeation Advantages Very Low Media Consumables Oxygen Consumption Unknown Disadvantages Sparse Info Availability</p><p>Earth Replications, Inc. Page 108 ASEN5116 Final Report Spacecraft Life Support Systems</p><p>IODINE MICROBIAL CHECK VALVE</p><p>H 0 H 0 + Iodine H20 MCV 2</p><p>HEAT</p><p>Subsystem Water Management Function Microbial Control Iodine Microbial Check Technology Valve Component Weight (kg) 101 Additional Weight (kg) 680 days 1.51 + 23.0 Power (kW) 0.0121 Volume (m3) ?? Design Efficiency (%) N/A Heat Generated (kW) 0.012 Operating Temperature (K) ?? Operating Pressure (kPa) 310 TRL (1-9) 93 Reliability High2 Maintainability High2 Inflow Stream Processed Hygiene Water Outflow Processing Iodine Removal Simple, Maintainable, Advantages Reliable Disadvantages Small Consumable Mass</p><p>Earth Replications, Inc. Page 109 ASEN5116 Final Report Spacecraft Life Support Systems</p><p>MILLIMULTIFILTRATION-Q ABSORBTION BED</p><p>POWER POWER</p><p>H20 + solids H20 H20 H20 MF MILLI-Q</p><p>HEAT HEAT</p><p>Subsystem Water Management Function Hygiene Post Processing Technology Mili-Q Absorbtion Component Weight (kg) 111 Additional Weight (kg) 680 days 861 Power (kW) 0.231 Volume (m3) 0.061 Design Efficiency (%) 100 Heat Generated (kW) 0.06 Operating Temperature (K) 293 Operating Pressure (kPa) 310 TRL (1-9) 63 Reliability High Maintainability High Pre Processed Hygiene Inflow Stream Water Outflow Processing Hygiene Water Simple, Maintainable, Advantages Reliable Consumable Mass, Disadvantages Proprietary</p><p>Earth Replications, Inc. Page 110 ASEN5116 Final Report Spacecraft Life Support Systems</p><p>REVERSE OSMOSIS AND ULTRAFILTRATION</p><p>POWER</p><p>H20 + solids H20</p><p>RO/UF Brine H20</p><p>HEAT</p><p>Subsystem Water Management Function Potable Processing Technology Multifiltration Component Weight (kg) 5101 Additional Weight (kg) 680 days 30921 Power (kW) 0.851 Volume (m3) 1.15 Design Efficiency (%) 1001 Heat Generated (kW) 0.85 Operating Temperature (K) 289 – 3282 Operating Pressure (kPa) 70 – 2102 TRL (1-9) 93 Reliability High2 Maintainability High2 Urine Distillate, Hygiene Inflow Stream Water Outflow Processing Microbial Simple, Maintainable, Advantages Reliable Disadvantages Mass</p><p>Earth Replications, Inc. Page 111 ASEN5116 Final Report Spacecraft Life Support Systems</p><p>VAPOR COMPRESSION DISTILLATION (VCD)</p><p>POWER</p><p>H20 Pretreated Urine VCD Brine</p><p>HEAT Subsystem Water Management Function Potable Processing Reverse Technology Osmosis/UltraFiltration Component Weight (kg) 901 Additional Weight (kg) 680 days 33.51 Power (kW) 0.351 Volume (m3) 0.31 Design Efficiency (%) 911 Heat Generated (kW) 0.35 Operating Temperature (K) 280 – 3202 Operating Pressure (kPa) 310 – 31002 TRL (1-9) +63 Reliability High2 Maintainability High2 Urine Distillate, Hygiene Inflow Stream Water Outflow Processing For TOC and Microbial Simple, Maintainable, Advantages Reliable Disadvantages Not 100% efficient</p><p>Subsystem Water Management</p><p>Earth Replications, Inc. Page 112 ASEN5116 Final Report Spacecraft Life Support Systems</p><p>CONDUCTIVITY</p><p>POWER</p><p>H2O H2O CONDUCTIVITY</p><p>Function Urine Distillation Vapor Compression Technology Distillation Component Weight (kg) 1012 Additional Weight (kg) 680 days 46.61 Power (kW) 0.1152 Volume (m3) 0.492 Design Efficiency (%) 952 Heat Generated (kW) 0.115 Operating Temperature (K) 3162 Operating Pressure (kPa) Low TRL (1-9) 6+ Reliability High Maintainability Medium Inflow Stream Pre-treated Urine Outflow Processing Hygiene/Potable Processing Advantages Low Power Consumption Disadvantages Complexity</p><p>Subsystem Water Management Function Water Monitoring</p><p>Earth Replications, Inc. Page 113 ASEN5116 Final Report Spacecraft Life Support Systems</p><p>ELECTRONIC NOSE</p><p>POWER</p><p>H2O H2O EN</p><p>Technology Conductivity TRL (1-9) 8-9 Reliability High give gross, general quality Advantages indication does not address TOC Disadvantages level Prospective Improvements high</p><p>Subsystem Water Management Function Water Monitoring</p><p>Earth Replications, Inc. Page 114 ASEN5116 Final Report Spacecraft Life Support Systems</p><p>ION SPECIFIC ELECTRODES</p><p>POWER</p><p>H2O H2O ISE</p><p>Technology Electronic Nose TRL (1-9) 6 Reliability Moderate monitor taste and metals. For odor (TRL 5) This will eliminate the need for the crews to do the odor or the tasting test of unsanitized Advantages water Disadvantages NOC (TRL 1) Prospective Improvements high</p><p>Subsystem Water Management Function Water Monitoring</p><p>Earth Replications, Inc. Page 115 ASEN5116 Final Report Spacecraft Life Support Systems</p><p>TEST KITS</p><p>H O 2 H O TEST KIT 2</p><p>Ion Specific Electrodes Technology (ISE) TRL (1-9) 2-8 Reliability High Mulitfuctions. Monitor conductivity (TRL 8), pH (TRL 6), iodine (TRL 2), TOC/COD (TRL 3), Advantages hardness (TRL 4) Low TRL for some parameter such as iodine Disadvantages monitoring Prospective Improvements High</p><p>Subsystem Water Management Function Water Monitoring</p><p>Earth Replications, Inc. Page 116 ASEN5116 Final Report Spacecraft Life Support Systems</p><p>Technology Test Kits TRL (1-9) 5-9 Reliability High Advantages Simple, potable, reliable Manual, need separate chem-strip for each particular parameter of Disadvantages interest Prospective Improvements High</p><p>TOTAL ORGANIC CARBON (TOC) - CONDUCTIVITY</p><p>POWER</p><p>H2O H2O TOC</p><p>Earth Replications, Inc. Page 117 ASEN5116 Final Report Spacecraft Life Support Systems</p><p>Subsystem Water Management Function Water Monitoring Total Organic Carbon Technology (TOC) - conductivity TRL (1-9) 6 Reliability High specialized in monitoring Advantages TOC/COD only Disadvantages only monitor TOC/COD Prospective Improvements high</p><p>Earth Replications, Inc. Page 118 ASEN5116 Final Report Spacecraft Life Support Systems</p><p>UF/R.O +apcos multifiltration UF/R.O.+milliq Input Values Weighted Scores Input Values Weighted Scores Input Values Weighted Scores Input Values Weighted Scores Criteria Wghtng Unwtd Unwtd Unwtd Unwtd Factor Certainty Score Certainty Score Certainty Score Certainty Score Selection Criteria (0.0-1.0) (0-100%) (0.0-1.0) Min Nom Max (0-100%) (0.0-1.0) Min Nom Max (0-100%) (0.0-1.0) Min Nom Max (0-100%) (0.0-1.0) Min Nom Component Weight (kg) 0.7 100% 0.7 0.51 0.51 0.51 100% 0.0 0.00 0.00 0.00 100% 0.8 0.56 0.56 0.56 100% 1.0 0.70 0.70 Additional Weight (kg) 0.7 25% 1.0 0.17 0.69 1.21 100% 0.0 0.00 0.00 0.00 100% 1.0 0.67 0.67 0.67 100% 1.0 0.70 0.70 Power (kW) 0.7 100% 0.0 0.00 0.00 0.00 100% 0.4 0.28 0.28 0.28 100% 0.6 0.41 0.41 0.41 100% 1.0 0.70 0.70 Volume (m3) 0.5 25% 0.7 0.08 0.33 0.58 100% 0.0 0.00 0.00 0.00 100% 0.7 0.33 0.33 0.33 100% 1.0 0.50 0.50 Heat Generated (kW) 0.5 100% 0.0 0.00 0.00 0.00 100% 0.4 0.18 0.18 0.18 100% 0.6 0.29 0.29 0.29 100% 1.0 0.50 0.50 Operating Temperature (K) 0.5 25% 0.0 0.00 0.00 0.00 100% 0.3 0.14 0.14 0.14 100% 0.3 0.14 0.14 0.14 100% 1.0 0.50 0.50 Operating Pressure (kPa) 0.5 25% 0.8 0.10 0.41 0.72 100% 0.9 0.44 0.44 0.44 100% 0.0 0.00 0.00 0.00 100% 1.0 0.50 0.50 Designed Efficiency (%) 0.5 25% 1.0 0.13 0.50 0.88 100% 1.0 0.50 0.50 0.50 100% 1.0 0.50 0.50 0.50 100% 0.0 0.00 0.00 TRL (1-9) 0.7 100% 1.0 0.70 0.70 0.70 100% 1.0 0.70 0.70 0.70 100% 0.8 0.54 0.54 0.54 100% 0.0 0.00 0.00 Maintainability 0.7 25% 0.3 0.06 0.23 0.41 75% 1.0 0.53 0.70 0.88 75% 1.0 0.53 0.70 0.88 100% 0.0 0.00 0.00 Crew Time - Operations 0.5 50% 0.5 0.13 0.25 0.38 50% 0.0 0.00 0.00 0.00 50% 0.0 0.00 0.00 0.00 100% 1.0 0.50 0.50 Safety 0.5 25% 0.5 0.06 0.25 0.44 75% 1.0 0.38 0.50 0.63 75% 0.0 0.00 0.00 0.00 100% 0.5 0.25 0.25 Totals 6.5 1.94 3.88 5.82 5.9 3.14 3.44 3.74 6.7 3.98 4.16 4.33 8.5 4.85 4.85</p><p>Summary: Min Nom Max UF/R.O +apcos 1.944 3.881 5.818 multifiltration 3.144 3.444 3.744 UF/R.O.+milliq 3.982 4.157 4.332 0 4.850 4.850 4.850 Alternative #5 4.600 4.600 4.600 Alternative #6 5.100 5.100 5.100 Alternative #7 5.100 5.100 5.100 Alternative #8 5.100 5.100 5.100 Alternative #9 4.850 4.850 4.850 Alternative #10 5.100 5.100 5.100</p><p>Earth Replications, Inc. Page 119 ASEN5116 Final Report Spacecraft Life Support Systems</p><p>VCD AES Input Values Weighted Scores Input Values Weighted Scores Input Values Weighted Scores Input Values Weighted Scores Criteria Wghtng Unwtd Unwtd Unwtd Unwtd Factor Certainty Score Certainty Score Certainty Score Certainty Score Selection Criteria (0.0-1.0) (0-100%) (0.0-1.0) Min Nom Max (0-100%) (0.0-1.0) Min Nom Max (0-100%) (0.0-1.0) Min Nom Max (0-100%) (0.0-1.0) Min Nom Component Weight (kg) 1.0 100% 0.0 0.00 0.00 0.00 100% 0.6 0.55 0.55 0.55 100% 1.0 1.00 1.00 1.00 100% 1.0 1.00 1.00 Additional Weight (kg) 1.0 100% 0.0 0.00 0.00 0.00 100% 0.6 0.58 0.58 0.58 100% 1.0 1.00 1.00 1.00 100% 1.0 1.00 1.00 Power (kW) 1.0 100% 0.9 0.89 0.89 0.89 100% 0.0 0.00 0.00 0.00 100% 1.0 1.00 1.00 1.00 100% 1.0 1.00 1.00 Volume (m3) 0.5 100% 0.0 0.00 0.00 0.00 100% 0.4 0.19 0.19 0.19 100% 1.0 0.50 0.50 0.50 100% 1.0 0.50 0.50 Heat Generated (kW) 0.5 100% 0.9 0.44 0.44 0.44 100% 0.0 0.00 0.00 0.00 100% 1.0 0.50 0.50 0.50 100% 1.0 0.50 0.50 Operating Temperature (K) 0.5 100% 0.0 0.02 0.02 0.02 100% 0.0 0.00 0.00 0.00 100% 1.0 0.50 0.50 0.50 100% 1.0 0.50 0.50 Operating Pressure (kPa) 0.5 100% 1.0 0.48 0.48 0.48 100% 0.0 0.00 0.00 0.00 100% 1.0 0.50 0.50 0.50 100% 1.0 0.50 0.50 Designed Efficiency (%) 1.0 100% 1.0 0.95 0.95 0.95 100% 1.0 1.00 1.00 1.00 100% 0.0 0.00 0.00 0.00 100% 0.0 0.00 0.00 TRL (1-9) 1.0 100% 1.0 1.00 1.00 1.00 100% 1.0 1.00 1.00 1.00 100% 0.0 0.00 0.00 0.00 100% 0.0 0.00 0.00 Maintainability 0.5 75% 0.3 0.13 0.17 0.21 100% 1.0 0.50 0.50 0.50 100% 0.0 0.00 0.00 0.00 100% 0.0 0.00 0.00 Crew Time - Operations 0.5 50% 1.0 0.25 0.50 0.75 50% 0.0 0.00 0.00 0.00 100% 1.0 0.50 0.50 0.50 100% 1.0 0.50 0.50 Safety 0.5 75% 0.7 0.25 0.33 0.42 75% 1.0 0.38 0.50 0.63 100% 0.0 0.00 0.00 0.00 100% 0.0 0.00 0.00 Totals 6.7 4.40 4.78 5.15 5.5 4.20 4.32 4.45 8.0 5.50 5.50 5.50 8.0 5.50 5.50</p><p>Summary: Min Nom Max VCD 4.400 4.775 5.150 AES 4.199 4.324 4.449 0 5.500 5.500 5.500 0 5.500 5.500 5.500 Alternative #5 5.500 5.500 5.500 Alternative #6 5.500 5.500 5.500 Alternative #7 5.500 5.500 5.500 Alternative #8 5.500 5.500 5.500 Alternative #9 5.500 5.500 5.500</p><p>Earth Replications, Inc. Page 120 ASEN5116 Final Report Spacecraft Life Support Systems</p><p>12.3 Waste Processing</p><p>PYROLYSIS</p><p>“Pyro-gas” Power Heat Gas treatment H2 H2O 2 2 or CO2 CO separation</p><p>CH4 NH3 Mixed waste Reactor (no metals) High T, P “Char” Char Activation or (C, N2, Ash) Disposal</p><p>Recycle and further breakdown</p><p>Subsystem Waste Processing Function Anaerobic breakdown of waste Technology Pyrolysis Processing</p><p>Component Weight (kg) 60 (4) Additional Weight (kg) 12</p><p>Power (kW) 0.6 (4) Volume (m3) 0.002 (4) Operating Temperature (K) 900 - 1300 (4) Operating Pressure (kPa) 500 - 2000 (4) TRL (1-9) 3 (4) Reliability High Microgravity and hypergravity concerns Gravity Dependence about separation of solid, liquid, and gas streams. (4) Safety High - Flexibility and adaptability with regard to feedstock (4) Advantages - Simplicity, low mass, and potentially useful products (4) - Reduction of waste volume (4) - Microgravity processing sensitivity (4) Disadvantages - High temperature operation (4) - Complex processing scheme (4) Prospective Improvements High</p><p>Earth Replications, Inc. Page 121 ASEN5116 Final Report Spacecraft Life Support Systems</p><p>GASIFICATION</p><p>Energy Recirculation (1)</p><p>Energy Energy Recirculation (2) Recirculation (3)</p><p>Heat Waste Gasifier High T Recovery Low T Energy Syn Gas Recovery Power Syn Gas Converter and Purifier Effluents</p><p>Ash and Energy Energy Slag Output Output</p><p>Verostko et. al (2002)</p><p>Subsystem Waste Processing Converts waste to gaseous Function products Technology Gasification</p><p>Component Weight (kg) 50 (4) Additional Weight (kg) 15*</p><p>Power (kW) 1 (4) Volume (m3) 2 (4) Operating Temperature (K) 400 - 1000 (4) Operating Pressure (kPa) 101.13 (4) TRL (1-9) 2 (4) Reliability High Gravity Dependence Gas-solid separation issues. (4) Safety High - Compact, reliable, safe to operate (4) -Potential for recovery of waste Advantages heat and valuable side products (4) (4) -Minimum NOx and SO2 -Little size reduction or preprocessing (4) -Low TRL (4) Disadvantages -High T (4) -Complex process (4) Prospective Improvements High</p><p>Earth Replications, Inc. Page 122 ASEN5116 Final Report Spacecraft Life Support Systems</p><p>SCWO</p><p>Catalytic Decomposition O2, N2 of N2O</p><p>Aqueous Gas/ Waste Insulated Liquid-Solid CO , H O Reactor 2 2 2 Separator</p><p>Inorganic H2O Salts</p><p>Subsystem Waste Processing Function Waste Oxidation Technology Supercritical Water Oxidation Component Weight (kg) 694 (1) Additional Weight (kg) 492 (5) (1) Power (kW) 0.65 - 1.44 Volume (m3) 0.289 (5) Operating Temperature (K) 647 – 1023 (1) Operating Pressure (kPa) 2.21 • 104 - 2.53 • 104 (1) TRL (1-9) 4 (5) Reliability Moderate Gravity Dependence Liquid & solid separation issues (5) Safety High -Processes all waste. (5 ) - High destruction efficiencies at short residence times (< 5 minutes). (1)(2) Advantages -Minimizes consumables and hazardous solids. (3) Low NOx and SOx (2) -Totally contained process. (2) -Clean products (5) -High P and T process (1) -Subsystem Functional Element Disadvantages weight and power (3) -Solids plugging (5) Prospective Improvements Moderate - High</p><p>Earth Replications, Inc. Page 123 ASEN5116 Final Report Spacecraft Life Support Systems</p><p>INCINERATION (BATCH & CONTINUOUS)</p><p>Power</p><p>Exhaust Gas Controlled H O CO N Continuous 2 2 2 Mixed waste (no metals) Thermal H20 Combustor Fly Ash</p><p>Subsystem Waste Processing sterilization & mass reduction Function of waste (no trace metals) (5) Incineration (Batch & Technology Continuous) Component Weight (kg) 303 Additional Weight (kg) 1296</p><p>Power (kW) 6.6 (4) 3 Volume (m ) 4.54 (4)</p><p>Operating Temperature (K) 814 (1) - 1400 (4)</p><p>Operating Pressure (kPa) ambient 101 (1)</p><p>TRL (1-9) 4 (4) Reliability High -Fluidized bed not microgravity Gravity Dependence capable (4) -Gas-solid separations occur (4) Safety Medium</p><p>- Sterile end product (1) - Mass and volume reduction (1) Advantages - Low operating pressure (3) - Minimizes hazardous solids (3) -Processes all waste (4)</p><p>- Incomplete combustion (1) -High operating temperature Disadvantages (1)) -Low water quality (2) -Flue gas contaminants (4) Prospective Improvements High</p><p>Earth Replications, Inc. Page 124 ASEN5116 Final Report Spacecraft Life Support Systems</p><p>PLASMA ARC</p><p>Power Heat</p><p>Exhaust Gas Reactor Mixed waste Scrubber Scrubber H2O CO2 N2 (no metals) High T Trace Gases Air Plasma Torch</p><p>Subsystem Waste Processing Sterilization & mass reduction of Function waste (except metals) (4)</p><p>Technology Plasma Thermal Arc Destruction (4)</p><p>Component Weight (kg) 1150 (4)</p><p>Additional Weight (kg) 263.7(4)</p><p>Power (kW) 34.8 (4) 3 Volume (m ) 3.9 (4)</p><p>Operating Temperature (K) 1100 - 1700 (4)</p><p>Operating Pressure (kPa) ambient (4)</p><p>TRL (1-9) 4 (4) Reliability High Gravity Dependence Heat transfer affected (4) - All surfaces need to be kept at safe temperatures Safety (4) - Electrical power can be shut off quickly in case of an emergency (4)</p><p>Advantages Processes all waste (4) - High power and temperature Disadvantages (4) - Scaledown difficult (4) Prospective Improvements Medium</p><p>Earth Replications, Inc. Page 125 ASEN5116 Final Report Spacecraft Life Support Systems</p><p>ELECTROCHEMICAL OXIDATION</p><p>Power</p><p>Off-gassing</p><p>All waste Reactor All waste H20 Anolyte</p><p>Subsystem Waste Processing sterilization and mass reduction Function of waste (processes all wastes) (5) Technology Electrochemical Oxidation</p><p>Component Weight (kg) 3310 (4)</p><p>Additional Weight (kg) 660 (4)</p><p>Power (kW) 20 (4) 3 Volume (m ) 4.2 (4) - 5.1(4)</p><p>Operating Temperature (K) 320 (4) - 422(1)</p><p>Operating Pressure (kPa) ambient (4)</p><p>TRL (1-9) 4 (4) Reliability Low -separation of the gases formed at Gravity Dependence the anode and cathode (5) Hazardous - includes Safety caustic and/or acidic liquids and gases including pure oxygen (5)</p><p>-Low power requirements. (1) -Does not consume oxygen. Advantages (1) -Low temperature and pressure.(4) -Low production of oxides. (4)</p><p>-Gaseous emissions include Cl2.(4) Disadvantages -Requires consistent and very fine particle waste. (4) Prospective Improvements Medium</p><p>Earth Replications, Inc. Page 126 ASEN5116 Final Report Spacecraft Life Support Systems</p><p>Electrochemical Ox. SCWO Gasification Input Values Weighted Scores Input Values Weighted Scores Input Values Weighted Scores Criteria Wghtng Unwtd Unwtd Unwtd Factor Certainty Score Certainty Score Certainty Score Selection Criteria (0.0-1.0) (0-100%) (0.0-1.0) Min Nom Max (0-100%) (0.0-1.0) Min Nom Max (0-100%) (0.0-1.0) Min Nom Max Component Weight (kg) 1.0 100% 0.0 0.00 0.00 0.00 100% 0.8 0.80 0.80 0.80 100% 1.0 1.00 1.00 1.00 Additional Weight (kg) 0.8 0% 0.0 0.00 0.00 0.00 50% 0.4 0.14 0.28 0.43 0% 1.0 0.00 0.80 1.60 Power (kW) 0.5 100% 0.0 0.00 0.00 0.00 100% 1.0 0.49 0.49 0.49 100% 1.0 0.50 0.50 0.50 Volume (m3) 0.5 100% 0.0 0.00 0.00 0.00 100% 1.0 0.50 0.50 0.50 100% 0.6 0.32 0.32 0.32 Heat Generated (kW) 0.0 100% 1.0 0.00 0.00 0.00 100% 0.0 0.00 0.00 0.00 100% 1.0 0.00 0.00 0.00 Operating Temperature (K) 0.8 100% 1.0 0.80 0.80 0.80 100% 0.0 0.00 0.00 0.00 100% 0.0 0.03 0.03 0.03 Operating Pressure (kPa) 0.8 100% 1.0 0.80 0.80 0.80 100% 0.0 0.00 0.00 0.00 100% 1.0 0.80 0.80 0.80 Designed Efficiency (%) 0.0 0% 0.8 0.00 0.00 0.00 100% 0.0 0.00 0.00 0.00 100% 1.0 0.00 0.00 0.00 TRL (1-9) 1.0 100% 1.0 1.00 1.00 1.00 100% 1.0 1.00 1.00 1.00 100% 0.0 0.00 0.00 0.00 Reliability 0.8 100% 0.0 0.00 0.00 0.00 50% 0.5 0.20 0.40 0.60 100% 1.0 0.80 0.80 0.80 Maintainability 0.5 100% 1.0 0.50 0.50 0.50 100% 1.0 0.50 0.50 0.50 100% 1.0 0.50 0.50 0.50 Gravity Dependence 0.8 100% 1.0 0.80 0.80 0.80 100% 1.0 0.80 0.80 0.80 100% 1.0 0.80 0.80 0.80 Crew Time - Operations 0.0 100% 0.0 0.00 0.00 0.00 100% 0.0 0.00 0.00 0.00 100% 1.0 0.00 0.00 0.00 Safety 0.8 100% 0.0 0.00 0.00 0.00 90% 1.0 0.72 0.80 0.88 90% 1.0 0.72 0.80 0.88 Lifetime Cost 0.0 100% 0.0 0.00 0.00 0.00 100% 0.0 0.00 0.00 0.00 100% 1.0 0.00 0.00 0.00 Prospective Improvements 0.5 100% 0.7 0.33 0.33 0.33 100% 0.7 0.33 0.33 0.33 80% 1.0 0.40 0.50 0.60 7.5 4.23 4.23 4.23 8.3 5.49 5.91 6.33 13.7 5.87 6.85 7.83</p><p>Summary: Min Nom Max Electrochemical Ox. 4.233 4.233 4.233 SCWO 5.486 5.909 6.331 Gasification 5.873 6.853 7.833</p><p>Earth Replications, Inc. Page 127 ASEN5116 Final Report Spacecraft Life Support Systems</p><p>Plasma Gasification Batch Incineration Input Values Weighted Scores Input Values Weighted Scores Input Values Weighted Scores Criteria Wghtng Unwtd Unwtd Unwtd Factor Certainty Score Certainty Score Certainty Score Selection Criteria (0.0-1.0) (0-100%) (0.0-1.0) Min Nom Max (0-100%) (0.0-1.0) Min Nom Max (0-100%) (0.0-1.0) Min Nom Max Component Weight (kg) 1.0 100% 0.0 0.00 0.00 0.00 100% 1.0 1.00 1.00 1.00 0% 0.8 0.00 0.77 1.54 Additional Weight (kg) 0.8 100% 0.8 0.64 0.64 0.64 0% 1.0 0.00 0.80 1.60 0% 0.0 0.00 0.00 0.00 Power (kW) 0.5 100% 0.0 0.00 0.00 0.00 100% 1.0 0.50 0.50 0.50 0% 0.8 0.00 0.42 0.83 Volume (m3) 0.5 100% 0.3 0.13 0.13 0.13 100% 1.0 0.50 0.50 0.50 50% 0.0 0.00 0.00 0.00 Heat Generated (kW) 0.0 100% 1.0 0.00 0.00 0.00 100% 1.0 0.00 0.00 0.00 100% 1.0 0.00 0.00 0.00 Operating Temperature (K) 0.8 100% 0.0 0.00 0.00 0.00 100% 1.0 0.80 0.80 0.80 100% 0.4 0.34 0.34 0.34 Operating Pressure (kPa) 0.8 100% 1.0 0.80 0.80 0.80 100% 1.0 0.80 0.80 0.80 100% 1.0 0.80 0.80 0.80 Designed Efficiency (%) 0.0 100% 0.0 0.00 0.00 0.00 100% 1.0 0.00 0.00 0.00 100% 0.5 0.00 0.00 0.00 TRL (1-9) 1.0 100% 0.7 0.67 0.67 0.67 100% 0.0 0.00 0.00 0.00 100% 0.7 0.67 0.67 0.67 Reliability 0.8 100% 1.0 0.80 0.80 0.80 100% 1.0 0.80 0.80 0.80 50% 1.0 0.40 0.80 1.20 Maintainability 0.5 100% 1.0 0.50 0.50 0.50 100% 0.0 0.00 0.00 0.00 0% 0.5 0.00 0.25 0.50 Gravity Dependence 0.8 100% 1.0 0.80 0.80 0.80 100% 1.0 0.80 0.80 0.80 100% 1.0 0.80 0.80 0.80 Crew Time - Operations 0.0 100% 0.0 0.00 0.00 0.00 100% 0.0 0.00 0.00 0.00 100% 0.0 0.00 0.00 0.00 Safety 0.8 95% 1.0 0.76 0.80 0.84 90% 1.0 0.72 0.80 0.88 50% 0.7 0.27 0.53 0.80 Lifetime Cost 0.0 100% 0.0 0.00 0.00 0.00 100% 0.0 0.00 0.00 0.00 100% 0.0 0.00 0.00 0.00 Prospective Improvements 0.5 80% 0.5 0.20 0.25 0.30 80% 1.0 0.40 0.50 0.60 0% 1.0 0.00 0.50 1.00 Totals 8.2 5.30 5.39 5.48 12.0 6.32 7.30 8.28 9.4 3.28 5.88 8.48 Continuous Incineration Pyrolysis Input Values Weighted Scores Input Values Weighted Scores</p><p>Summary: Min Nom Max Unwtd Unwtd Plasma 5.297 5.387 5.477 Certainty Score Certainty Score Gasification 6.320 7.300 8.280 (0-100%) (0.0-1.0) Min Nom Max (0-100%) (0.0-1.0) Min Nom Max Batch Incineration 3.276 5.880 8.484 100% 0.8 0.77 0.77 0.77 100% 1.0 0.99 0.99 0.99 Continuous Incineration 4.478 5.030 5.581 50% 0.0 0.00 0.00 0.00 0% 1.0 0.00 0.80 1.60 Pyrolysis 4.797 6.107 7.417 100% 0.8 0.42 0.42 0.42 100% 0.9 0.43 0.43 0.43 100% 0.0 0.00 0.00 0.00 100% 1.0 0.50 0.50 0.50 100% 0.0 0.00 0.00 0.00 100% 0.9 0.00 0.00 0.00 100% 0.6 0.46 0.46 0.46 100% 0.6 0.46 0.46 0.46 100% 0.0 0.00 0.00 0.00 100% 0.0 0.00 0.00 0.00 100% 0.5 0.00 0.00 0.00 100% 1.0 0.00 0.00 0.00 100% 1.0 1.00 1.00 1.00 100% 0.3 0.33 0.33 0.33 80% 1.0 0.64 0.80 0.96 80% 1.0 0.64 0.80 0.96 50% 0.5 0.13 0.25 0.38 30% 1.0 0.15 0.50 0.85 100% 1.0 0.80 0.80 0.80 100% 1.0 0.80 0.80 0.80 100% 0.0 0.00 0.00 0.00 100% 0.0 0.00 0.00 0.00 50% 0.7 0.27 0.53 0.80 50% 0.0 0.00 0.00 0.00 100% 0.0 0.00 0.00 0.00 100% 1.0 0.00 0.00 0.00 100% 0.0 0.00 0.00 0.00 100% 1.0 0.50 0.50 0.50 6.8 4.48 5.03 5.58 11.7 4.80 6.11 7.42</p><p>Earth Replications, Inc. Page 128 ASEN5116 Final Report Spacecraft Life Support Systems</p><p>References 1. Eckart, Peter. 1996. Spaceflight Life Support and Biospherics. Torrance: Microcosm Press 2. Davidson, William. 2002.Supercritical Water Oxidation: State of the Art Environmental Technology. General Atomics, Advanced Technologies Group. Available from the World Wide Web: http://www.ga.com/atg/aps/scwo.html 3. Ferrall, J.F., G.B. Ganapathi, N.K. Rohatgi and P.K. Seshan. 1994. Life Support Systems Analysis and Technical Trades for a Lunar Outpost. NASA Technical Memorandum 109927 4. Verostko, C., J. Joshi, M. Alazraki and J. Fisher. 2002. Solid Waste Processing and Resource Recovery Workshop Report - Vol I. Engineering Directorate, crew and Thermal Systems Division. CTSD-ADV-474. Available also from the World Wide Web: http://advlifesupport.jsc.nasa.gov/PubNew.html 5. Verostko, C., J. Joshi, M. Alazraki and J. Fisher. 2002. Solid Waste Processing and Resource Recovery Workshop Report Appendix - Vol II. Engineering Directorate, crew and Thermal Systems Division. CTSD-ADV-474. Available also from the World Wide Web: http://advlifesupport.jsc.nasa.gov/PubNew.html</p><p>Earth Replications, Inc. Page 129 </p>
Details
-
File Typepdf
-
Upload Time-
-
Content LanguagesEnglish
-
Upload UserAnonymous/Not logged-in
-
File Pages135 Page
-
File Size-