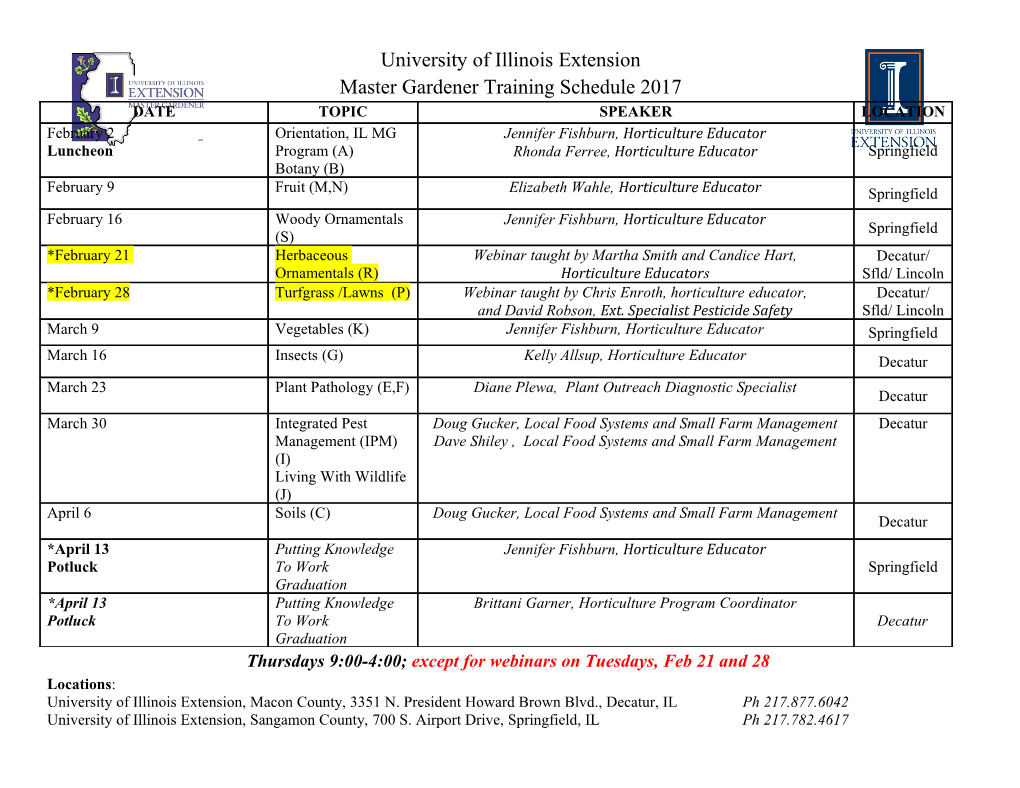
<p> Supplementary Information for:</p><p>Characterizing clay mineralogy in Lake Towuti, Indonesia, with reflectance spectroscopy</p><p>Andrea K. Weber a, *, 1, James M. Russell a, Timothy A. Goudge a, Mark R. Salvatore a, 2, John F. Mustard a, Satria Bijaksanab</p><p> a Department of Earth, Environmental, and Planetary Sciences, Brown University, 324 Brook St., Providence, RI 02912, United States. b Global Geophysics Research Group, Faculty of Mining and Petroleum Engineering, Institut Teknologi Bandung, Jalan Ganesa 10, Bandung, Indonesia, 40132.</p><p>*Corresponding author. Tel: +1 401 301 4429. E-mail address: [email protected]. Department of Earth, Environmental, and Planetary Sciences, Brown University, 324 Brook St., Providence, RI 02912, United States.</p><p>1 Present Address: School of Engineering and Applied Sciences, Harvard University, 58 Oxford Street, Cambridge, MA 02138</p><p>2 Present Address: Arizona State University, School of Earth and Space Exploration, Tempe, AZ 85287-1404</p><p>ASD measurements An Analytical Spectral Devices (ASD) FieldSpec 3 (ASD Inc., CO, USA) portable spectrometer </p><p>(subsequently referred to as an ASD Spectrometer) was the primary instrument used to measure the visible to near-infrared (VNIR) reflectance spectra of the Lake Towuti samples. The ASD </p><p>Spectrometer covers the wavelength range from 350-2,500 nm, has a spectral resolution of ~10 nm, a sampling interval of 1 nm, and was set to take an average of 25 spectral scans. In addition to the 25 averaged spectral scans taken by the ASD Spectrometer, an average of 5 consecutively collected spectra was taken for each sample to diminish variability in the ASD Spectrometer data. ASD Spectrometer measurements were made relative to a Spectralon® white reference, and corrected for the absolute reflectance properties of Spectralon®. ASD measurements were made using an external light source and bare fiber optic cable, with incidence and emission angles of approximately 30° and 0°, respectively.</p><p>Comparison of ASD and higher-resolution spectral data</p><p>In addition to ASD spectra, higher-resolution spectral data were acquired for three lake sediment samples using a Bidirectional Reflectance (BDR) spectrometer (Pieters 1983) at the Brown </p><p>University Keck/NASA Reflectance Experiment Lab (RELAB). These data were acquired to evaluate and validate the lower-resolution-spectral VNIR reflectance data rapidly acquired with the ASD Spectrometer.</p><p>Spectra from the high-spectral-resolution BDR spectrometer and the lower-spectral- resolution ASD Spectrometer have the same spectral features, indicating that the ASD </p><p>Spectrometer data are reliable (ESM Fig. 1). Both sets of data were analyzed using the built-in ENVI® continuum removal, which employs a convex hull algorithm. This algorithm fits linear segments between points on a spectrum that are local maxima, and is thus a multi-part linear continuum. Whereas the BDR and ASD Spectrometer data are similar, there are slight differences in the strength of the absorption bands that are likely caused by a number of factors including instrumental error, differences in calibration of the data to reflectance, differences in spectral resolution, and slightly different viewing geometries. However, differences in the region of interest for this study (~2.2-2.4 m) were minimal.</p><p>Modified Gaussian model</p><p>The modified Gaussian model (MGM) described by Sunshine et al. (1990) for electronic crystal field absorptions is based upon the principle that the precise energy (or inversely, wavelength) of an absorption feature is controlled by the relevant bond length within minerals. Whereas one precise bond length should give rise to an infinitely narrow absorption feature (i.e. a line absorption), defects within the confines of an imperfect crystal structure lead to an approximately</p><p>Gaussian distribution of bond lengths. Additionally, Sunshine et al. (1990) noted that the energy of an electronic absorption feature is controlled by the relevant bond length raised to the nth power, which, when fit to laboratory transmission spectra, yields an exponent of -1. This leads to the modified Gaussian equation used in the MGM:</p><p>[1] where m(x) is the modified Gaussian function, x is energy, s is the absorption strength, is the absorption center energy, and σ is the standard deviation, or width of the absorption. Although the MGM was initially developed and validated for crystal field absorptions caused by octahedrally coordinated Fe2+ within pyroxene (Sunshine et al. 1990), Mustard (1992) showed that the MGM can also be used to model vibrational absorption bands caused by OH within actinolite. For each region of interest (2.2 m and ~2.2-2.4 m), a beginning and end point was set to equal 1, allowing the absorption bands for each sample to be compared. The endpoints for the ~2.2-2.4 m region were 2.225 m and 2.365 m and the endpoints for the 2.2 region were </p><p>2.135 m and 2.235 m. Separate files were made for each sample for every region of interest and were then run through the MGM model. </p><p>XRD measurements</p><p>Randomly oriented powder XRD data (ESM Fig. 3) were collected on two samples using a </p><p>Bruker D2 PHASER instrument. This instrument uses a CuKα radiation source with 10 mA and </p><p>30 kV, and a Ni filter. Our experimental setup involved a 0.6 mm divergence, a 3 mm anti- scatter shield, and a 2.5° Soller slit. Our samples were run over the 2θ range 2-50°, with a step- size of ~0.02° 2θ, and a dwell time of 5 seconds per step. Our results were analyzed using the </p><p>Bruker DIFFRAC.EVA (version 3.1) program, and major mineral phases were identified using the International Centre for Diffraction Data (ICDD) PDF-2 mineral database in </p><p>DIFFRAC.EVA.</p><p>References: Mustard JF (1992) Chemical analysis of actinolite from reflectance spectra. Amer Miner 77: </p><p>345–258</p><p>Pieters CM (1983) Strength of mineral absorption features in the transmitted component of near-</p><p> infrared reflected light: First results from RELAB. J Geophys Res 88: 9534–9544</p><p>Sunshine JM, Pieters CM, Pratt SF (1990) Deconvolution of mineral absorption bands: An </p><p> improved approach. J Geophys Res 95: 6955–6966 ESM Table 1 Model parameters for each absorption band modeled with the MGM. Full width at</p><p> half maximum (FWHM) is used as a width indicator</p><p>Representative Mineral Center (m) FWHM (m) Standard Deviation (m)</p><p>Kaolinite 2.205 0.027 0.0127 Nontronite 2.295 0.025 0.0115 Saponite + Serpentine 2.315 0.025 0.0106 Serpentine 2.345 0.03 0.0106</p><p>ESM Table 2 Table of R values found in the graphs from Fig. 7</p><p>Element Associated R Value P-value Number of Mineral Observations</p><p>Al Kaolinite 0.8141 < 0.01 33</p><p>Mg Saponite + 0.8980 < 0.01 33 Serpentine Fe Nontronite 0.5119 < 0.01 33 ESM Fig. 1 Lake sediment spectra acquired with the BDR, (A) and (B), and ASD Spectrometer, </p><p>(C) and (D). Spectra in parts (A) and (C) are shown continuum removed. Note that spectra from the high-spectral-resolution BDR spectrometer (A and B) and the lower-spectral-resolution ASD </p><p>Spectrometer (C and D) have the same spectral features ESM Fig. 2 MGM fit of core top sample 10a-1G. (A) Fit at 2.21 µm. (B) Fit at 2.3 µm. The pink line is residual error. The blue lines are the modified Gaussians used for the fit, the red line is the modeled continuum, the black line is the overall fit, and the orange crosses show the sample spectra ESM Fig. 3 XRD data of samples 2A-1G-7-8.0 cm (green line) and 9A-1G-3.5-4.5 cm (blue line). Sample 2A-1G-7-8.0 cm has a strong 2.21 m Al-OH (kaolinite) absorption and Al-rich elemental chemistry, whereas sample 9A-1G-3.5-4.5 cm has a strong 2.34 m Mg-OH </p><p>(serpentine) absorption and Mg-rich elemental chemistry. Major peaks used for mineral identification are labeled with the diffraction plane shown in parentheses. K refers to kaolinite, S to serpentine, Q to quartz, and Sm to smectite</p>
Details
-
File Typepdf
-
Upload Time-
-
Content LanguagesEnglish
-
Upload UserAnonymous/Not logged-in
-
File Pages9 Page
-
File Size-