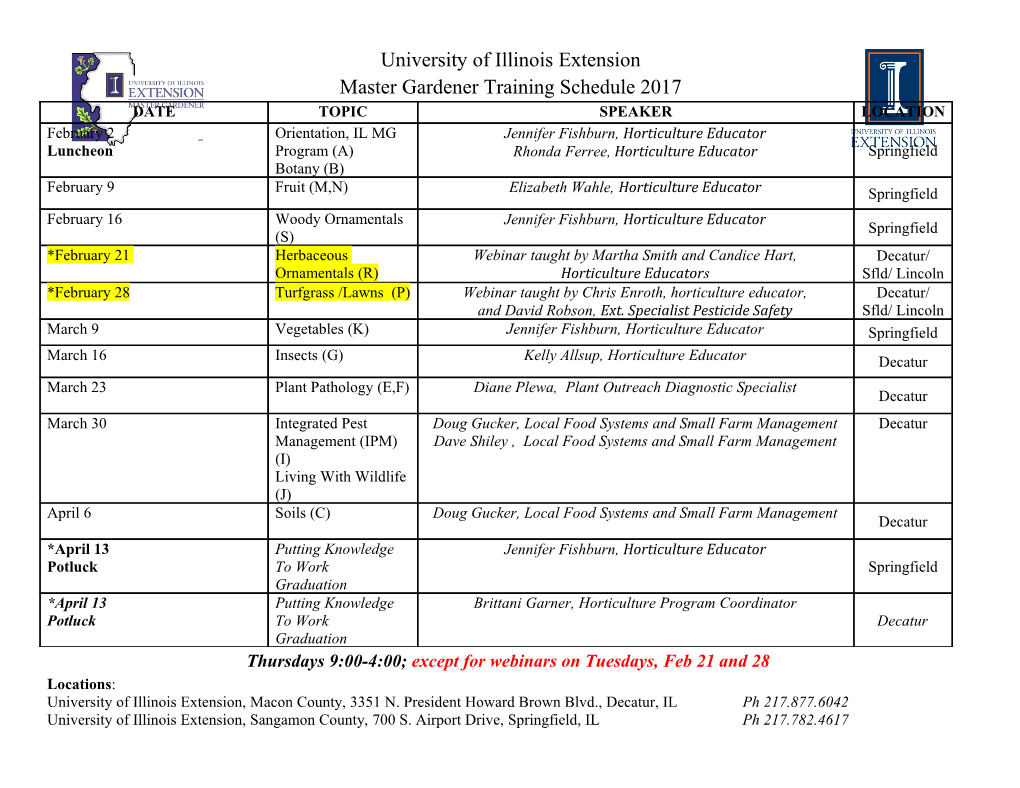
Synthesis of Nuclei at the Limits of Nuclear Stability P. Armbruster, GSI Darmstadt The cosmos of isotopes is limited and the part accessible to man restricted. One of the tasks of nuclear physics is to explore the limits and constraints on the production and stability of hot nuclear systems and the essential re­ quirements for a long-living ground state. At GSI, Darmstadt, for the first time the limit of instability to proton emission has been crossed and the limit of stability against spontaneous fission raised to the level of Z = 107 and 109. Fig. 1 — The chart of nuclides between Z = 37 and Z = 109. Shown also are the proton and neutron drip-tines and the lines of equal pro­ bability for neutron and proton emission and fis­ sion. 0 = isotopes discovered at GSI. With heavy ion accelerators, nuclear fused systems are heavier than each of the give increased ground state stability to reactions can be induced between all collision partners so that isotopes heavier elements beyond Z = 114. The isotopes of isotopes provided they are available for than those existing naturally can be made. these superheavy elements are much more targets in mg-amounts or for beams in But, beyond lead, the disruptive Coulomb neutron rich than the heaviest nuclei made g-amounts. A large variety of new nuclear forces become more and more dominant, until now. The most stable nucleus reactions have been found, but unfortuna­ thus limiting the production of heavier and cannot be reached by any combination of tely these reactions in most cases lead to heavier elements. available isotopes, but as known regions of nuclei which have been known before. To shell stabilisation are rather extended, there produce new species, two reactions are of Limitations on Fusion of Heavy Nuclei is hope that isotopes at accessible landing major interest: the fusion of two heavy For the production of isotopes of places still have high enough fission bar­ nuclei and the massive transfer of nucleons elements beyond the natural ones, three riers to allow detection. observed in deep inelastic heavy ion reac­ conditions have to be fulfilled each of The losses during de-excitation of a fus­ tions. If in the latter reaction one of the col­ which may limit the feasibility of producing ed compound system depend on the stabi­ lision partners picks up predominantly neu­ a given nucleus. lity of nuclei against fission in states far trons, this mechanism may be used to pro­ (1) To detect a nucleus, its half-life against above the ground state. Especially the tem­ duce neutron-rich new isotopes giving ac­ spontaneous fission must be sufficiently perature dependence of those contribu­ cess to those regions on the neutron-rich long; ns-half-lives these days can be mea­ tions to the fission barrier which are due to side of the valley of stability which have not sured. shell effects are of importance. As all fu­ been explored by fission product studies. (2) The probability of fission during the sion reactions produce nuclei that are However, all the isotopes produced are far cooling down phase of the fused com­ highly excited, the production of super­ from the neutron drip-line, the line where pound system must be small enough to heavy elements may be decided by such a nuclei become unstable to further increase guarantee production rates of at least a few dependence of shell effects on intrinsic ex­ in their neutron content. atoms per week. citation energy. To minimise the fission Fusion reactions allow us to produce (3) The Coulomb repulsion between the losses in the de-excitation phase, the highly proton-rich isotopes, Fig. 1, as all fused two collision partners during the fusion fissionable nuclei should be produced with systems possess less neutrons than the process must be small enough to allow the the smallest excitation energy possible. most stable isotopes at the corresponding formation of a fused monosystem. The colder the system formed, the better is atomic number. Isotopes at the proton The disappearance of a barrier against its chances of survival. But how cold can drip-line become accessible up to the spontaneous fission limits the detection of fusion be? region of lead. Beyond the drip-line, pro­ transiently formed isotopes near element Finally, the Coulomb repulsion between tons are expected to tunnel through the 110 although nuclei with high fission bar­ the nuclei may prevent the formation of a Coulomb barrier in a new kind of radioac­ riers resulting from a stabilisation by shell compound system. Passing the Coulomb tivity analogous to αα-decay. Moreover, the effects at N = 184 and Z = 114-126 may barrier, an appreciable amount of the radial 4 produced from symmetric collision partners count in addition that the proton and neu­ than from more asymmetric ones. It is tron ratio between the two partners is smaller, if especially stable nuclei are used equilibrated very quickly (within times of as collision partners notably 48Ca, 90Zr, 10-22 s) and that the nuclear system at the 10-22 s), and 208Pb. It is smaller for fusion Coulomb barrier for those systems where below the Coulomb barrier and it has been the "extra-push" becomes of importance, shown that there is fusion in addition to the is more compact than the two-touching quantummechanical barrier penetration. sphere configuration, a parameter Some collective motions in nuclei are describing the ratio of Coulomb and slower than the barrier passing time. The nuclear forces has been defined, which is fluctuations in shape allow the two nuclei applied to organise the vast amount of data to fuse (with a small probability) at larger collected in the past years. Fig. 3 shows the distances and hence a reduced Coulomb extra-push energy using as a reference a Fig. 2 — "Radiative fusion" is found in the reac­ barrier. The phenomenon is shown to de­ Bass-potential to calculate the Coulomb tion 90Zr + 90Zr → 180Hg at 3.9 MeV/nucleon. pend strongly on the nuclear structure of barrier as a function of this parameter. The The a-spectrum shows the y-channel, 1n-chan­ Coulomb forces surmounting 74% of the nel, 1p-channel, and further daughter decays. the collision partners. The number of evaporated particles in nuclear forces, the "extra-push" comes in­ energy on the long way from a two-tou- the de-excitation cascade after fusion is a to play. It increases beyond this point ching nuclei configuration to a compound measure of the excitation energy. It has system is transferred into intrinsic excita­ been decreased from the 4-5 neutrons in tion energy. This energy has to be dissipa­ the early fusion studies at Berkeley leading ted during the de-excitation phase, during to elements Z = 101-106, via 2-neutron which the losses by fission are increased. reactions found at Dubna, and 1-neutron The energy transferred into excitation de-excitation found in. 1979 at GSI, to a de­ energy is reflected as a virtual increase of excitation by pure y-emission found recent­ the Coulomb barrier, an "extra-push ener­ ly in the reaction 90Zr + 90Zr → 180Hg at gy" is needed to fuse. This limitation in the GSI. The a-spectrum of the isotopes pro­ entrance channel finally prevents the pro­ duced in the reaction is presented in Fig. 2. duction of heavy elements, even if the de­ It shows below the barrier at an excitation excitation process could be survived and energy of 17 MeV the 1 proton-, 1 neu­ a ground state stability would exist. tron-, and the y-channel. The reaction Nuclear theory is able to calculate combines all the advantages leading to a ground state properties of heavy nuclei. cold fusion reaction: heavy, symmetric, But to produce nuclei at the limits of stabili­ and shell stabilized collision partners fused ty an understanding of fusion dynamics below the barrier. The cross-section for Fig. 4 — Evaporation residue cross section for the production of the "pseudo superheavies" and the behaviour of hot nuclei is needed "radiative fusion" is high, about 50 µb, as around 216Th. The 4n-channel — 40 MeV excita­ as well. Progress here is of importance. fission competition is still small. The fused tion energy — shows liquid drop behaviour and Lacking other guidance, an interplay of system 180Hg is situated at the triple point no preferential yield at N = 126, whereas a slight reaction studies and production experi­ where proton and neutron separation ener­ increase is seen in the yield of the 1n-channel — ments was used to find ways to go beyond gies and the fission barrier are equal and 20 MeV excitation energy — indicating shell ef­ the elements reached previously. "Cold fu­ high, (Fig. 1). Around 180Hg we find the fects in spherical shell stabilized systems to sion" and "extra-push energy" are the new nuclei the most resistive to intrinsic excita­ become of importance in the production below ingredients in the recipes of element mak­ tion. If these are produced cold, they reveal this energy. ing. They allowed the limit reached at Z = the long searched "radiative fusion", the quadratically. Already at 84% about 20 106 to be passed in the early seventies at ultimate in cold fusion. MeV of additional energy is introduced into Lawrence Berkeley Lab. the system. The excitation energy at the "Extra-Push" barrier for reactions leading into the region Cold Fusion The ratio of disruptive Coulomb forces of superheavy elements is for all possible The excitation energy of a compound and attractive nuclear forces governs the combinations of isotopes increased by the system is the difference between the amalgamation of two nuclei into one.
Details
-
File Typepdf
-
Upload Time-
-
Content LanguagesEnglish
-
Upload UserAnonymous/Not logged-in
-
File Pages4 Page
-
File Size-