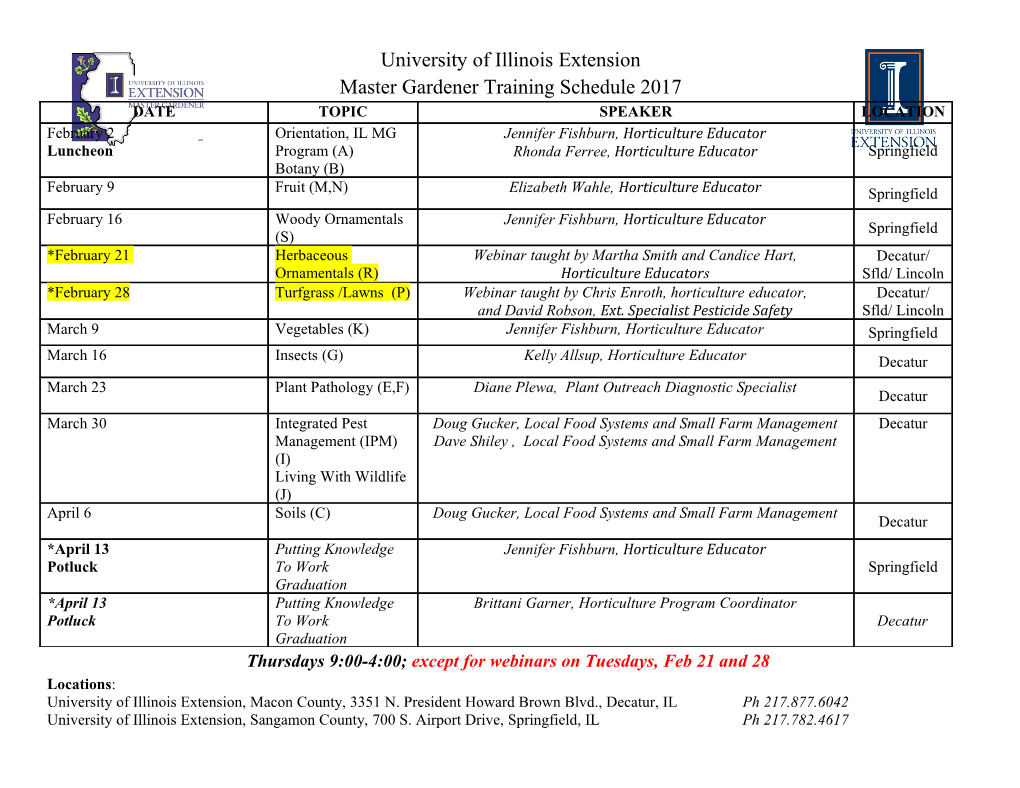
Integrated Modeling and Design of Photoelectrochemical Water-Splitting Cells By Alan Day Berger A dissertation submitted in partial satisfaction of the requirements for the degree of Doctor of Philosophy in Chemical Engineering in the Graduate Division of the University of California, Berkeley Committee in charge: Professor John Newman, Chair Professor Rachel Segalman Professor Costas Grigoropoulos Spring 2014 Abstract Integrated Modeling and Design of Photoelectrochemical Water-Splitting Cells by Alan Day Berger Doctor of Philosophy in Chemical Engineering University of California, Berkeley Professor John Newman, Chair The photoelectrochemical production of fuels is an interesting research topic that aims to provide a low-cost method for storing solar energy. A one-dimensional model of a photoelectrochemical cell for solar water splitting has been developed, with applicability to both wired and wireless designs. The model of the light absorber handles electron and hole transport. The model of the electrolyte accounts for mass transport through regions of aqueous solution, including stagnant diffusion layers and bulk regions to address mixing due to bubbles, natural convection, or other sources. A polymer membrane may be present in the electrolyte. The models of the light absorber and the electrolyte are integrated through the reactions taking place at the interface between them. Charge transfer from the semiconductor to the solution is handled using a kinetic model involving reactions between the species in both the light absorber and the electrolyte. A simplified model is also presented for use when concentration gradients in the electrolyte are negligible. The simplified model captures the effect of the electrolyte in the boundary conditions for the light-absorber. Throughout, the model is validated against experimental data. At the outset, simulated output compares favorably with current-potential data for a hydrogen-evolving light absorber with varying degrees of simulated solar illumination. Later, the program is able to match current- potential data and bulk pH values for a membrane electrolysis cell with several electrolytes. The model is first used to study the effect of changing the electrolyte on the performance of a photoelectrochemical cell. It is discovered that using supported dilute acids or buffered electrolytes in an attempt to work in near-neutral conditions is ineffective. Cells with neutral electrolytes cannot run at high current density due to transport limitations in the electrolyte and solubility limitations that are encountered due to electrodialysis. Later, an absorber-in-membrane design for a photoelectrochemical cell is considered. Gas crossover is identified as a significant issue in these systems, and metrics are developed for evaluating system performance properly. Material targets are established. For instance, membranes with ten times the gas-blocking properties of currently available polymers (i.e., Nafion) are desired. 1 Contents List of Figures ................................................................................................................................ iii List of Tables .................................................................................................................................. v Acknowledgements ........................................................................................................................ vi 1. Introduction ............................................................................................................................. 1 1.1. Solar Fuels ........................................................................................................................ 1 1.2. Photoelectrochemical cells ............................................................................................... 2 1.3. Approach .......................................................................................................................... 5 1.4. References ........................................................................................................................ 6 2. Integrated model of a Photoelectrochemical Cell ................................................................. 10 2.1. Introduction .................................................................................................................... 10 2.2. Cell geometry ................................................................................................................. 11 2.3. Governing Equations for Light Absorber....................................................................... 15 2.3.1. Boundary Conditions for Light Absorber ............................................................... 17 2.4. Governing Equations for Electrolyte.............................................................................. 21 2.5. Boundary conditions ...................................................................................................... 23 2.5.1. Boundary conditions for electrolyte/anode interface .............................................. 23 2.5.2. Boundary conditions for electrolyte/cathode interface ........................................... 25 2.5.3. Internal boundary conditions for electrolyte/membrane interface .......................... 26 2.6. Integrated Model with Simplified Electrolytic Transport .............................................. 26 2.7. Full Integrated Model ..................................................................................................... 29 2.7.1. Solution methods and parameters ........................................................................... 31 2.7.2. Experimental validation .......................................................................................... 31 2.8. References ...................................................................................................................... 33 3. Impact of Electrolyte pH on Photoelectrochemical Cell Performance ................................. 37 3.1. Approach ........................................................................................................................ 37 3.2. Contributions to the cell potential .................................................................................. 37 i 3.3. Comment on electrolyte pH ........................................................................................... 39 3.3.1. Solution composition at equilibrium ....................................................................... 40 3.3.2. Solution composition when passing current ........................................................... 40 3.4. Diffusion layers and mixing ........................................................................................... 41 3.5. Current due to shuttling of buffer species ...................................................................... 47 3.6. Cell potential .................................................................................................................. 51 3.6.1. Experimental validation .......................................................................................... 51 3.7. Model results for dilute acid with supporting electrolyte .............................................. 53 3.8. Model results for buffer electrolyte ................................................................................ 60 3.9. Solution pH .................................................................................................................... 62 3.9.1. Model results for dilute acid with supporting electrolyte ....................................... 62 3.9.2. Model results for buffer electrolyte ........................................................................ 64 3.10. Efficiencies and gas purity ......................................................................................... 68 3.11. Conclusions ................................................................................................................ 71 3.12. References .................................................................................................................. 71 4. Analysis of Absorber-in-Membrane Configuration for Photoelectrochemical Cells ........... 74 4.1. Introduction .................................................................................................................... 74 4.2. System description ......................................................................................................... 75 4.3. Effect of crossover: numerical solution with PEC model .............................................. 79 4.3.1. Net hydrogen collected and true efficiency of a PEC ............................................. 79 4.3.2. Trade-off between high conductivity and low permeability ................................... 83 4.3.3. Limits on the benefit of reducing permeability....................................................... 86 4.4. Effect of crossover: simplified semi-analytical solution ................................................ 89 4.5. Effect of absorber efficiency .......................................................................................... 91 4.6. Effect of absorber area fraction ...................................................................................... 95 4.7. Summary of absorber-in-membrane model.................................................................... 96 4.8. References .....................................................................................................................
Details
-
File Typepdf
-
Upload Time-
-
Content LanguagesEnglish
-
Upload UserAnonymous/Not logged-in
-
File Pages109 Page
-
File Size-