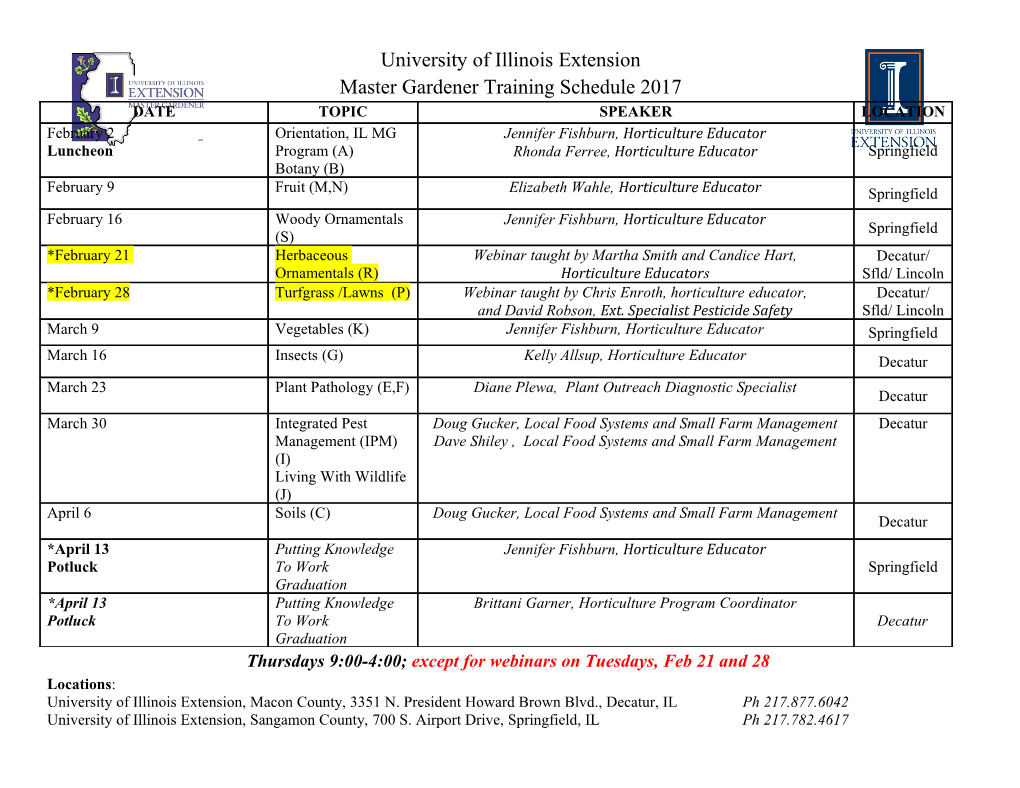
R EPORTS minus the background levels observed in the HSP in X-100 for 15 min at 4¡C with intermittent mixing, 62. M. R. Peterson, C. G. Burd, S. D. Emr, Curr. Biol. 9, 159 the control (Sar1-GDPÐcontaining) incubation that and elutes were pooled. Proteins were precipitated by (1999). prevents COPII vesicle formation. In the microsome MeOH/CH3Cl and separated by SDSÐpolyacrylamide 63. M. G. Waters, D. O. Clary, J. E. Rothman, J. Cell Biol. control, the level of p115-SNARE associations was gel electrophoresis (PAGE) followed by immunoblot- 118, 1015 (1992). less than 0.1%. ting using p115 mAb 13F12. 64. D. M. Walter, K. S. Paul, M. G. Waters, J. Biol. Chem. 46. C. M. Carr, E. Grote, M. Munson, F. M. Hughson, P. J. 51. V. Rybin et al., Nature 383, 266 (1996). 273, 29565 (1998). Novick, J. Cell Biol. 146, 333 (1999). 52. K. G. Hardwick and H. R. Pelham, J. Cell Biol. 119, 513 65. N. Hui et al., Mol. Biol. Cell 8, 1777 (1997). 47. C. Ungermann, B. J. Nichols, H. R. Pelham, W. Wick- (1992). 66. T. E. Kreis, EMBO J. 5, 931 (1986). ner, J. Cell Biol. 140, 61 (1998). 53. A. P. Newman, M. E. Groesch, S. Ferro-Novick, EMBO 67. H. Plutner, H. W. Davidson, J. Saraste, W. E. Balch, 48. E. Grote and P. J. Novick, Mol. Biol. Cell 10, 4149 J. 11, 3609 (1992). J. Cell Biol. 119, 1097 (1992). (1999). 54. A. Spang and R. Schekman, J. Cell Biol. 143, 589 (1998). 68. D. S. Nelson et al., J. Cell Biol. 143, 319 (1998). 49. P. Uetz et al., Nature 403, 623 (2000). 55. M. F. Rexach, M. Latterich, R. W. Schekman, J. Cell 69. We thank G. Waters for p115 cDNA and p115 mAbs; 50. GST-SNARE proteins were expressed in bacteria and Biol. 126, 1133 (1994). G. Warren for p97 and p47 antibodies; R. Scheller for purified on glutathione-Sepharose beads using stan- 56. A. Mayer and W. Wickner, J. Cell Biol. 136, 307 (1997). rbet1, membrin, and sec22 cDNAs; H. Plutner for dard methods. Immobilized GST-SNARE protein (0.5 excellent technical assistance; and P. Tan for help M) was incubated with rat liver cytosol (20 mg) or 57. M. D. Turner, H. Plutner, W. E. Balch, J. Biol. Chem. during the initial phase of this work. Supported by purified recombinant p115 (0.5 M)in1mlofNS 272, 13479 (1997). NIH grants GM 33301 and GM42336 and National buffer containing 1% BSA for 2 hours at 4¡C with 58. A. Price, D. Seals, W. Wickner, C. Ungermann, J. Cell Cancer Institute grant CA58689 (W.E.B.), a NIH Na- rotation. Beads were briefly spun (3000 rpm for 10 s) Biol. 148, 1231 (2000). tional Research Service Award (B.D.M.), and a Well- and sequentially washed three times with NS buffer 59. X. Cao and C. Barlowe, J. Cell Biol. 149, 55 (2000). come Trust International Traveling Fellowship and three times with NS buffer supplemented with 60. G. G. Tall, H. Hama, D. B. DeWald, B. F. Horazdovsky, (B.B.A.). 150 mM NaCl. Bound proteins were eluted three Mol. Biol. Cell 10, 1873 (1999). times in 50 l of 50 mM tris-HCl (pH 8.5), 50 mM 61. C. G. Burd, M. Peterson, C. R. Cowles, S. D. Emr, Mol. reduced glutathione, 150 mM NaCl, and 0.1% Triton Biol. Cell 8, 1089 (1997). 20 March 2000; accepted 22 May 2000 have two different catalytic activities, and One Sequence, Two Ribozymes: could access by neutral drift every sequence on both networks. With intersecting net- Implications for the Emergence works, RNAs with novel structures and ac- tivities could arise from previously existing ribozymes, without the need to carry non- of New Ribozyme Folds functional sequences as evolutionary inter- Erik A. Schultes and David P. Bartel* mediates. Here, we explore the proximity of neutral networks experimentally, at the level We describe a single RNA sequence that can assume either of two ribozyme of RNA function. We describe a close appo- folds and catalyze the two respective reactions. The two ribozyme folds share sition of the neutral networks for the hepatitis no evolutionary history and are completely different, with no base pairs (and delta virus (HDV) self-cleaving ribozyme probably no hydrogen bonds) in common. Minor variants of this sequence are and the class III self-ligating ribozyme. highly active for one or the other reaction, and can be accessed from prototype In choosing the two ribozymes for this in- ribozymes through a series of neutral mutations. Thus, in the course of evo- vestigation, an important criterion was that they lution, new RNA folds could arise from preexisting folds, without the need to share no evolutionary history that might con- carry inactive intermediate sequences. This raises the possibility that biological found the evolutionary interpretations of our RNAs having no structural or functional similarity might share a common results. Choosing at least one artificial ri- ancestry. Furthermore, functional and structural divergence might, in some bozyme ensured independent evolutionary his- cases, precede rather than follow gene duplication. tories. The class III ligase is a synthetic ri- bozyme isolated previously from a pool of ran- Related protein or RNA sequences with the ate isolates have the same fold and function, it dom RNA sequences (9). It joins an oligonu- same folded conformation can often perform is thought that they descended from a common cleotide substrate to its 5Ј terminus. The very different biochemical functions, indicating ancestor through a series of mutational variants prototype ligase sequence (Fig. 1A) is a short- that new biochemical functions can arise from that were each functional. Hence, sequence het- ened version of the most active class III variant preexisting folds. But what evolutionary mech- erogeneity among divergent isolates implies the isolated after 10 cycles of in vitro selection and anisms give rise to sequences with new macro- existence of paths through sequence space that evolution. This minimal construct retains the molecular folds? When considering the origin have allowed neutral drift from the ancestral activity of the full-length isolate (10). The HDV of new folds, it is useful to picture, among all sequence to each isolate. The set of all possible ribozyme carries out the site-specific self-cleav- sequence possibilities, the distribution of se- neutral paths composes a “neutral network,” age reactions needed during the life cycle of quences with a particular fold and function. connecting in sequence space those widely dis- HDV, a satellite virus of hepatitis B with a This distribution can range very far in sequence persed sequences sharing a particular fold and circular, single-stranded RNA genome (11). space (1). For example, only seven nucleotides activity, such that any sequence on the network The prototype HDV construct for our study are strictly conserved among the group I self- can potentially access very distant sequences by (Fig. 1B) is a shortened version of the antige- splicing introns, yet secondary (and presumably neutral mutations (3–5). nomic HDV ribozyme (12), which undergoes tertiary) structure within the core of the ri- Theoretical analyses using algorithms for self-cleavage at a rate similar to that reported bozyme is preserved (2). Because these dispar- predicting RNA secondary structure have for other antigenomic constructs (13, 14). suggested that different neutral networks are The prototype class III and HDV ribozymes interwoven and can approach each other very have no more than the 25% sequence identity Whitehead Institute for Biomedical Research and De- closely (3, 5–8). Of particular interest is expected by chance and no fortuitous struc- partment of Biology, Massachusetts Institute of Tech- nology, 9 Cambridge Center, Cambridge, MA 02142, whether ribozyme neutral networks approach tural similarities that might favor an intersec- USA. each other so closely that they intersect. If so, tion of their two neutral networks. Neverthe- *To whom correspondence should be addressed. E- a single sequence would be capable of fold- less, sequences can be designed that simulta- mail: [email protected] ing into two different conformations, would neously satisfy the base-pairing requirements 448 21 JULY 2000 VOL 289 SCIENCE www.sciencemag.org R EPORTS of both the HDV and ligase ribozymes, while prototype HDV ribozyme (40 substitutions, bonds in common, yet sequences such as this preserving most of the residues important for one deletion, and three insertions). In Fig. can readily be designed that might lie within, the activity of each ribozyme. An example of 2A, this sequence was color-coded in accor- or very near to, the neutral networks for both such a sequence is shown (Fig. 2A). This dance with the ligase secondary structure and ribozymes. sequence is 42 mutational steps away from then threaded into the HDV secondary struc- When this sequence was synthesized in the the prototype ligase (39 base substitutions, ture, illustrating that the two ribozyme folds two formats of Fig. 2A, catalytic activity was one point deletion, and two single-nucleotide have no base pairs in common. The two detectable for both self-ligation and site-specif- insertions) and 44 mutational steps from the tertiary structures probably have no hydrogen ic self-cleavage (Fig. 2B). Ligation occurred with formation of a 2Ј,5Ј-phosphodiester link- age (15), the regiospecificity of the class III Fig. 1. Secondary structures of the pro- ligase (9), indicating that the class III ligase fold totype ribozymes. When designing ri- was assumed by some of the molecules. Cleav- bozyme derivatives, we strived to main- age occurred with formation of a cyclic phos- tain important Watson-Crick pairing (thick dashes), wobble pairing (oval), phate (15), as expected for the HDV fold (11, and the identity of key residues (pink 16).
Details
-
File Typepdf
-
Upload Time-
-
Content LanguagesEnglish
-
Upload UserAnonymous/Not logged-in
-
File Pages5 Page
-
File Size-