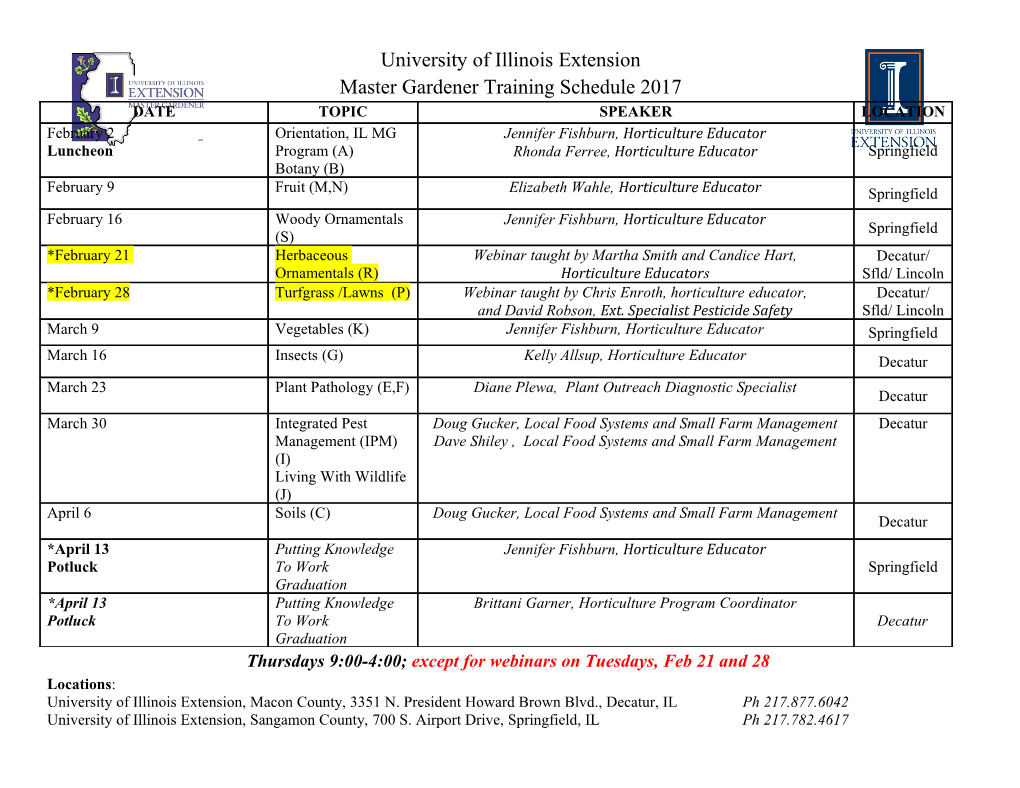
Origin and Evolution of the Sierra Nevada and Walker Lane themed issue Paleochannels, stream incision, erosion, topographic evolution, and alternative explanations of paleoaltimetry, Sierra Nevada, California John Wakabayashi Department of Earth and Environmental Sciences, California State University, Fresno, California 93740, USA ABSTRACT of late Cenozoic uplift and stream incision 2008; Cassel et al., 2009, 2012; Chamberlain suggests a relationship with triple-junction et al., 2012), and interpreted steep paleogradi- Geologic relationships in the Sierra migration, possibly associated with slab ents of Oligocene and Eocene stream deposits Nevada, California, show negligible stream window development, with a second uplift (Cassel and Graham, 2011; Cassel et al., 2012). incision between Eocene and Late Miocene– pulse related to delamination and limited These interpretations directly contradict pro- Pliocene time. Stream incision of up to ~1 km to the southern Sierra (San Joaquin River posals for late Cenozoic rock and surface uplift began at (from south to north) ca. 20 Ma in drainage and southward). Basement features based on thermochronology (McPhillips and the Kern to Kings River drainages, between may have signifi cantly infl uenced along- and Brandon, 2010, 2012), geodetically measured 6 and 10 Ma in the San Joaquin River drain- across-strike differences in Cenozoic tec- current uplift rates of the range (Hammond age, 3.6–4 Ma in the Stanislaus and Moke- tonics and geomorphic response. et al., 2012), and a combination of geologic lumne River drainages, and ca. 3 Ma in the fi eld relationships and evaluation of geomorphic American and Feather River drainages. INTRODUCTION process (Lindgren, 1911; Hudson, 1955; Bate- These differences in incision timing greatly man and Wahrhaftig, 1966; Christensen, 1966; exceed the time of knickpoint retreat, based For over a century, geomorphologists have Huber, 1981, 1990; Unruh, 1991; Wakabayashi on the example of the North Fork Feather used the Sierra Nevada of California as a natu- and Sawyer, 2001; Jones et al., 2004; Stock River, where the knickpoint may have ral laboratory to explore general geomorphic et al., 2004, 2005; Clark et al., 2005; Figueroa retreated over 100 km in less than 300 k.y. process (e.g., Lawson, 1903; Matthes, 1937; and Knott, 2010; Kemp, 2012). based on ages of interfl uve-capping ande- Wahrhaftig, 1965). In the past few decades, the This paper will fi rst review data on stream sites and an inset basalt fl ow. The knickpoint Sierra Nevada has attracted attention as a local- incision, erosion, and evidence for pre–late in the Stanislaus River may have retreated ity for evaluating climatically versus tectonically Cenozoic relief (“paleorelief”) following Waka- over 50 km in less than 400 k.y. based on driven geomorphic evolution (Small and Ander- bayashi and Sawyer (2001), but with signifi cant somewhat looser constraints. Eocene paleo- son, 1995), an example of epeirogenic uplift updates and additions on the timing of incision, channels show lowest gradients parallel to (uplift not associated with major shortening evaluation of knickpoint migration speed, fault- the range axis, steepest ones perpendicular, or collision) and its causes (e.g., Unruh, 1991; ing, exhumation and erosion rates, along-strike and reaches with signifi cant “uphill” gra- Ducea and Saleeby, 1998; Jones et al., 2004; Le differences, and paleorelief east of the current dients that rise in the paleo-downstream Pourhiet et al., 2006), as well as a location for Sierra Nevada. I will then revisit the relationship direction. Modern Sierran rivers lack this evaluation of erosion and weathering processes between paleochannel gradients and azimuth relationship. The azimuth-gradient relation- and their forcing mechanisms (e.g., Small and and “inverse” (uphill in present-day setting) ships of paleochannels, especially the uphill Anderson, 1995; Granger et al., 2001; Riebe gradients (from Lindgren, 1911; Hudson, 1955; gradients, require late Cenozoic tilting and et al., 2000, 2001; Stock et al., 2005; Clark et al., Jones et al., 2004) that starkly contrasts with uplift. Incision began in spite of decreasing 2005; Phillips et al., 2011). modern stream reaches. The stream incision discharge and increasing sediment load and A debate has emerged as to whether or not late history and paleogradient-azimuth relation will must have resulted from steepening associ- Cenozoic rock and surface uplift has occurred in then be discussed in the context of supporting ated with tilting and uplift. Stable-isotope the Sierra Nevada, and this debate has implica- evidence for late Cenozoic uplift, followed by paleoaltimetry apparently records a profi le tions for analysis of general geomorphic process presentation of alternative interpretations of the similar to that of the modern range and areas and evaluation of recently developed methods paleoaltimetry data, and speculations on con- east of it, in spite of signifi cant vertical defor- in paleoaltimetry. Some suggested a lack of late nections between tectonic mechanisms, uplift, mation that postdates the age of the sampled Cenozoic uplift on the basis of thermochronol- and topographic evolution. deposits, suggesting fairly recent reequilibra- ogy (e.g., House et al., 1998), or on the basis of tion, in contrast to the published interpre- the generally thin Sierra Nevada crust and the GENERAL GEOLOGIC FRAMEWORK tations of closed-system behavior since the tectonic setting of the Sierra Nevada (Wernicke AND TECTONIC HISTORY Oligocene or Eocene. Such apparent open- et al., 1996). More recently, researchers have system behavior agrees with studies showing proposed a lack of late Cenozoic uplift on the The 600-km-long Sierra Nevada is the most progressive hydration of volcanic glass and basis of paleobotany (e.g., Hren et al., 2010), prominent mountain range in California. The the correspondence between weathering and isotopic paleoaltimetry (e.g., Poage and Cham- Sierra Nevada and the Central Valley belong erosion rates. Northward-younging initiation berlain, 2002; Mulch et al., 2006; Crowley et al., to the Sierra Nevada microplate, an element of Geosphere; April 2013; v. 9; no. 2; p. 191–215; doi:10.1130/GES00814.1; 7 fi gures; 1 table. Received 30 April 2012 ♦ Revision received 1 December 2012 ♦ Accepted 23 December 2012 ♦ Published online 5 February 2013 For permission to copy, contact [email protected] 191 © 2013 Geological Society of America Downloaded from http://pubs.geoscienceworld.org/gsa/geosphere/article-pdf/9/2/191/3342129/191.pdf by guest on 01 October 2021 Wakabayashi the broad Pacifi c–North American plate bound- lacks the simple block topography of regions to inson and Snyder, 1978). The resultant crustal ary (Argus and Gordon, 1991) (Fig. 1). The the north, and in the southernmost part of the thickening apparently resulted in a broad region Sierra Nevada microplate displays little inter- Kern drainage, the highest elevations are on a of elevated topography, comparable to the nal deformation north of the Kern River drain- divide west of the crest, and topography of this Altiplano or Tibetan Plateau (Christiansen and age and moves northwestward relative to stable region is controlled more by faults along the Yeats, 1992; Dilek and Moores, 1999), with a North America at ~10–14 mm/yr, a fourth or Kern River than the FFS (Saleeby et al., 2009). drainage divide hundreds of kilometers east of fi fth of the dextral motion of the plate boundary Crestal elevations vary from 2100 to 2700 m the current crest of the Sierra Nevada (Chris- (Argus and Gordon, 1991; Dixon et al., 2000). in the northern part of the range to 4000–4400 m tiansen and Yeats, 1992; Henry, 2008; Henry The microplate is bounded on the west by an in the central to southern part of the range, with and Faulds, 2010; Henry et al., 2012). Follow- active fold-and-thrust belt that marks the east- the highest elevations in the headwaters of the ing cessation of Cretaceous arc magmatism, ern margin of the Coast Range province (e.g., Kings and Kern Rivers, and maximum elevations exhumation resulted in surface exposure of the Went worth and Zoback, 1989), and on the east decreasing to the south. The height of the eastern batholith. Eocene stream gravels, sourced well by a prominent east-facing escarpment that escarpment varies from ~1000 m in the northern- east of the present Sierran crest (e.g., Garside marks the Sierra Nevada Frontal fault system most part of the range to nearly 3300 m at Lone et al., 2005), were deposited on the exhumed (referred to as Frontal fault system or FFS herein), Pine (Fig. 2). granitic and older metamorphic rocks in what a zone of normal, normal-dextral, and dextral The axis of the range that parallels smoothed became the northern Sierra Nevada (Bateman faulting (e.g., Clark et al., 1984; Beanland and (fi tted across drainages) elevation contours on and Wahrhaftig, 1966). Clark, 1994; Unruh et al., 2003; Le et al., 2007) the western fl ank varies from about N35°W Regional-scale Cenozoic volcanism began (Fig. 2). Whereas the down-to-the-east compo- in the Feather River drainage to N25°W in the with 31–23 Ma rhyolitic ash fl ow tuff deposi- nent of deformation across the FFS fault strands southern Yuba River and American River drain- tion in channels crossing the Sierra (Garside results in prominent east-facing topographic ages, to N40°W from the Stanislaus River south- et al., 2005; Henry and Faulds, 2010; Henry escarpments ranging from 500 to 3000 m in ward. This along-strike change in the range axis et al., 2012), and culminated with widespread height, dextral shear predominates along this orientation mirrors along-strike changes in the and voluminous andesitic volcanism from 16 transtensional fault system (Unruh et al., 2003). strike of the Frontal fault system (Fig. 2) (Waka- to 3 Ma, associated with the ancestral Cascades The FFS separates the Sierra Nevada microplate bayashi and Sawyer, 2001; Unruh et al., 2003).
Details
-
File Typepdf
-
Upload Time-
-
Content LanguagesEnglish
-
Upload UserAnonymous/Not logged-in
-
File Pages25 Page
-
File Size-