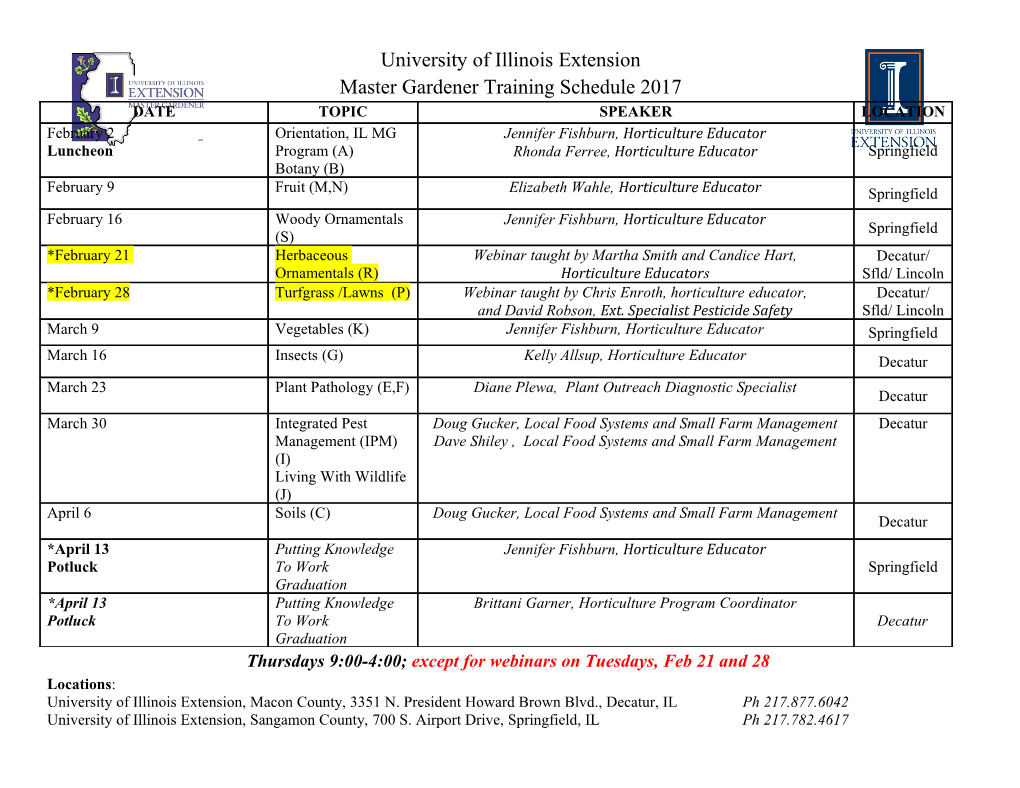
www.nature.com/npjquantmats ARTICLE OPEN Observation of two superconducting domes under pressure in tetragonal FeS Jun Zhang1, Feng-Liang Liu1,2, Tian-Ping Ying1, Na-Na Li2, Yang Xu1, Lan-Po He1, Xiao-Chen Hong1, Yun-Jie Yu1, Ming-Xiang Wang1, Jian Shen1,3, Wen-Ge Yang2,4 and Shi-Yan Li1,3 We investigate the evolution of superconductivity and structure with pressure for the new superconductor FeS (Tc ≈ 4.5 K), a sulfide counterpart of FeSe. A rapid suppression of Tc and vanishing of superconductivity at 4.0 GPa are observed, followed by a second superconducting dome from 5.0 to 22.3 GPa with a 30% enhancement in maximum Tc. An onsite tetragonal to hexagonal phase transition occurs around 7.0 GPa, followed by a broad pressure range of phase coexistence. The residual deformed tetragonal phase is considered as the source of second superconducting dome. The observation of two superconducting domes in iron-based superconductors poses great challenges for understanding their pairing mechanism. npj Quantum Materials (2017) 2:49 ; doi:10.1038/s41535-017-0050-7 INTRODUCTION high-pressure works,31 but superconductivity had not been observed As the second family of high-T superconductor, the iron-based until high-quality stoichiometric tetragonal FeS was successfully c 30 superconductors (IBSs) have been extensively studied in recent synthesized by low-temperature hydrothermal method. The tetra- years.1–3 Among those IBSs, the tetragonal FeSe with the simplest gonal FeS has the same crystal structure as the tetragonal FeSe, and structure has attracted much attention recently.4 Although the T their electronic structures are also quite similar based on first principle c 32 of bulk FeSe is modestly low (≈8 K),4 it can be enhanced through calculation. A slight difference between them is that FeSe under- goes a phase transition to orthorhombic structure at 90 K,33 while FeS various methods. By applying high pressure, the Tc of bulk FeSe 34 can reach up to 36.7 K.5, 6 Through intercalation, surface K dosing, remains its tetragonal structure down to 10 K. Interestingly, recent 7–11 thermal conductivity and specific heat measurements suggested or ionic liquid gating, one can also enhance the Tc to above 40 K. nodal superconductivity in FeS.35, 36 Surprisingly, the Tc can be further enhanced above 60 K by 12–16 Here we present in situ high-pressure electrical transport and growing monolayer FeSe thin film on SrTiO3 substrate, and 17 synchrotron X-ray diffraction (XRD) measurements on tetragonal even above 100 K on Nb-doped SrTiO3 substrate. It was believed that the enhanced electron–phonon coupling at interface is FeS single crystals. Upon applying pressure, two superconducting 18, 19 domes are observed. The first dome manifests a continuous crucial in the further enhancement of Tc. The angle-resolved photoemission spectroscopy studies reveal decrease of Tc with increasing pressure, ending ~4.0 GPa. Then a two distinct electronic band structures for these FeSe-based second superconducting dome emerges from 5.0 GPa and lasts to 22.3 GPa, with an over 30% increasing in Tc (≈6.0 K) from the superconductors. The Fermi surface of the undoped bulk FeSe fi Γ highest Tc in the rst dome. Comparing to the two super- consists of hole pockets around and electron pockets around 37 15, 20–23 conducting domes of (K/Tl/Rb)xFe2-ySe2 reported earlier, the M. However, for intercalated (Li0.8Fe0.2)OHFeSe, monolayer FeSe thin film, and surface K dosed FeSe single crystal or film, the superconducting pressure range is much wider, meaning the Fermi surface consists of only electron pockets, which apparently superconducting phase is much more sustainable with pressure in results from electron doping.7, 13–16, 24–27 For undoped bulk FeSe, FeS. For its crystal structure, a hexagonal phase starts to set in at ~7.0 GPa, and there is a large coexisting pressure range of the superconducting pairing symmetry is most likely s ± -wave tetragonal and hexagonal phases. On the basis of the drop in R(T) with sign reversal between the hole and electron pockets,28 while curves and the structure refinement results of mixture phase for monolayer FeSe/SrTiO , plain s-wave superconductivity was 3 region, we believe that the second superconducting dome is suggested by scanning tunneling microscopy (STM) study.29 originated from the residual deformed tetragonal phase of FeS. Because of the large variety of FeSe-based superconductors with a wide range of Tc, clarifying the superconducting mechanism will be a major step towards solving the issue of high-temperature superconductivity in IBSs. RESULTS Very recently, superconductivity with Tc ≈ 4.5 K at ambient pressure Characterization at ambient pressure was reported for FeS,30 the sister compound of FeSe. As an important The inset of Fig. 1a is a photo of as-grown FeS single crystals. Earth Science material, FeS has been extensively studied including Figure 1a shows a typical XRD pattern, in which only the (00l) 1State Key Laboratory of Surface Physics, Department of Physics, and Laboratory of Advanced Materials, Fudan University, Shanghai 200433, China; 2Center for High Pressure Science and Technology Advanced Research (HPSTAR), Shanghai 201203, China; 3Collaborative Innovation Center of Advanced Microstructures, Nanjing 210093, China and 4High Pressure Synergetic Consortium (HPSynC), Geophysical Laboratory, Carnegie Institution of Washington, Argonne, IL 60439, USA Correspondence: Wen-Ge Yang ([email protected]) or Shi-Yan Li ([email protected]) Jun Zhang, Feng-Liang Liu and Tian-Ping Ying contributed equally to this work Received: 2 November 2016 Revised: 2 July 2017 Accepted: 11 July 2017 Published in partnership with Nanjing University Observation of two superconducting domes J Zhang et al. 2 Fig. 1 a Typical XRD pattern of FeS single crystals. The inset shows a photo of the as-grown FeS single crystals. b Single crystal XRD spots of an FeS sample. The inset is an SEM image of the surface. c Low-temperature dc magnetization measured in the ZFC mode at H = 10 Oe parallel to the c axis. d Temperature dependence of the resistivity ρ(T). The inset is an enlarged view of the superconducting transition. ZFC, zero-field- cooled Bragg peaks show up, indicating the crystals are well oriented resistance at 15 K is shown in Fig. S1 in the Supplemental along the c axis. Further XRD measurement on these crystals Materials. Initially, the Tc is suppressed rapidly with increasing shows sharp single crystal diffraction spots (Fig. 1b). Flat and pressure, consistent with previous measurements below 2.2 38, 39 grain-boundary-free surface was observed with SEM (inset of GPa. Here, we observe Tc eventually disappears at 4.0 GPa. Fig. 1b). Therefore, the single crystalline nature of our FeS samples The superconductivity in this region is so sensitive to pressure that is confirmed. the transition broadens and the resistance does not drop to zero Figure 1c shows a typical low-temperature dc magnetization even under 0.86 GPa. Figure 2b, c show the normalized resistance of FeS single crystals, from which the superconducting transition is curves above 5.0 GPa. The drop of resistance re-emerges below at ~4.1 K. The temperature dependence of resistivity ρ(T)at 4.5 K at 5.0 GPa, implying the arise of another superconducting ambient pressure is plotted in Fig. 1d. The absence of a phase. This resistance drop exists in a wide pressure range from resistivity anomaly in the normal state suggests no structural 5.0 to 22.3 GPa, and maximum Tc reaches 6 K, a 30% enhancement 33 phase transition, which is different from FeSe single crystal. The from the highest value in the first dome. With further increasing low-temperature resistivity between 5 and 50 K can be pressure, the resistance drop vanishes and the R(T) curve exhibits 2 well described by the Fermi liquid theory, ρ(T)=ρ0 + AT, giving a semiconducting behavior. −3 2 ρ0 = 6.07 μΩ cm and A = 2.0 × 10 μΩ cm/K . The residual resistiv- To make sure the resistance drop under high pressure ity ratio, RRR = ρ(298 K)/ ρ0 = 40, is much larger than that reported represents a superconducting transition, we applied magnetic previously for FeS flakes.30 The inset of Fig. 1d displays an field to the low-temperature resistance measurements at 19.0 GPa. enlarged view around the superconducting transition, from which As shown in Fig. 2d, the resistance drop is gradually suppressed to onset zero onset Tc ≈ 4.7 K and Tc ≈ 4.3 K are obtained. Tc is determined lower temperature with increasing field, which demonstrates that as the temperature where the resistivity deviates from the normal- it is indeed a superconducting transition. The inset of Fig. 2d plots zero state behavior, while Tc as the temperature where the the reduced temperature T = Tc dependence of the upper critical resistivity drops to zero. In the following discussions, we use field Hc2. The data can be fitted to the generalized onset 2 2 Tc as Tc. Ginzburg–Landau model: Hc2(T)=Hc2 (0) (1−t )/(1 + t ), where t = T/Tc. According to the fit, Hc2 (0) ≈ 0.81 T is obtained. Tc evolution under pressure The temperature dependence of resistance up to 4.0 GPa is Crystal structure evolution under pressure plotted in Fig. 2a, where the resistance is normalized to the value In situ synchrotron powder XRD measurements were utilized to at 15 K for each pressure. The pressure dependence of the study the structural evolution of FeS with pressure. Figure 3a npj Quantum Materials (2017) 49 Published in partnership with Nanjing University Observation of two superconducting domes J Zhang et al.
Details
-
File Typepdf
-
Upload Time-
-
Content LanguagesEnglish
-
Upload UserAnonymous/Not logged-in
-
File Pages6 Page
-
File Size-