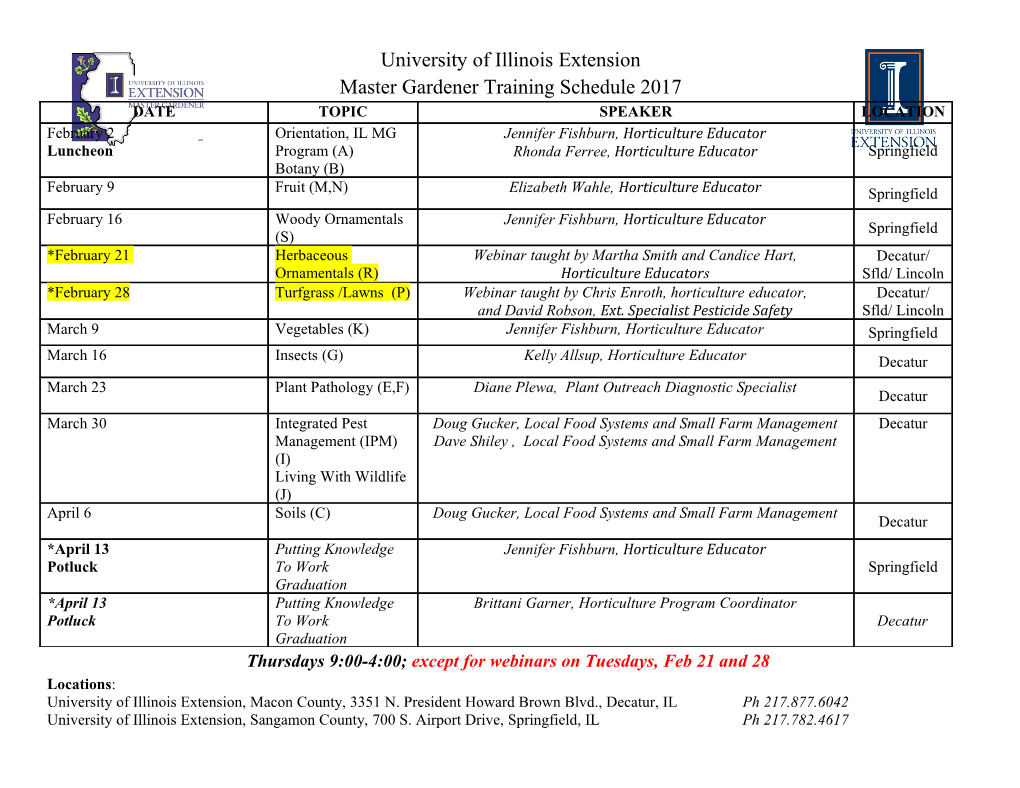
INSPACE PROPULSION--WHERE WE STAND AND WHAT'S NEXT Robert L. Sackheim Assistant Director and Chief Engineer for Propulsion NASA Marshall Space Flight Center, Marshall Space Flight Center, Alabama USA GBSTRGCT launch vehicles, many spacecraft suppliers, such as Baeing (formerly Hughes), Loral, and Northrop- The focus of this paper will be on the three stages Grumman (formerly TRW), have developed integral of in-space transportation propulsion systems, now propulsion systems (IPSs) on board their spacecraft commonly referred to as in-space propulsion (ISP); to perform many of the more conventional orbit i.e., the transfer of payloads from low-Earth orbits transfer functions. Some examples include the into higher orbits or into trajectories for planetary Hughes 601 and 702 commercial series of satellite encounters, including planetary landers and sample buses and the Northrop-Grumman IPS for the return launchers, if required. Functions required recent Chandra final orbit insertion functions. There at the operational location where ISP must provide have only been three complete upper stages devel- thrust for orbit include maintenance, position oped over the last 30 years: (1) The basic Centaur control, stationkeeping, and spacecraft altitude con- and all the associated upgrades, (2) the advanced trol; i.e., proper pointing and dynamic stability in Centaurs for the Delta 3 and 4 and the Atlas 3 and 5, inertial space; and the third function set to enable and (3) the inertial upper stage which is the only operations at various planetary locations, such upper stage that is currently certified for launch as atmospheric entry and capture, descent to the in a shuttle. This has forced spacecraWpayload sup- surface and ascent, back to rendezvous orbit. The pliers to develop advanced onboard Ipssto economi- discussion will concentrate on where ISP stands cally meet their needs, primarily with their own today and some observations of what might be next financial investments. Unfortunately, major depen- in line for new ISP technologies and systems for near- dence on in-house development funding has mini- term and future flight applications. The architectural mized the capability of IPSs as well as significantly choices that are applicable for ISP will also be increased their operational risk in many cases. described and discussed in detail. OF CURRENT STATUS After the payload is placed in a low-Earth park- ing orbit by the launcher, an upper stage is frequently Table 1lists the primary upper stage and space- used to transfer the payload to its operational orbit. craft (in-space) propulsion options. Cold gas Upper stage designs, especially their weight and size, propulsion systems are inexpensive, low- are strongly driven by the performance and weight performance systems that are rarely used unless the= efficiency of theii primary propulsion system. The is an overriding requirement to avoid the hot gases specific impulse (I ), propellant density, and over- and/or perceived safety concerns for liquid and solid sp. all stage mass fiactlon of the primary propulsion systems. Solid propellant-based systems have been system are key parameters. However, because of the used extensively for orbital insertion, but the lack of development of higher performance upper spacecraft's propulsion subsystem must be stages, especially those that would be compatible augmented with another technology to provide with reusable launch vehicles as well as expendable orbital maintenance maneuvering, velocity control, 1 ,', 1 .> , .. Table 1. Principal options for spacecraft propulsion subsystems. Figure 1. Secondary combustion augmented thnrster-bimodal spacecraft attitude and velocity control RCS thruster. and attitude control. Liquid systems are divided into to those that are readily available, storable, and easy monopropellant and bipropellant systemswith a third to handle. Also, we must weigh the lead time needed alternative, dual mode; i.e., a bipropellant derivative. to develop new hardware against any limitations hm Monopropellantsystems have successfully provided using a combination of existing components or orbital maintenance contml and attitude control func- stages. Finally, limits on payload acceleration may tions for hundreds of spacecraft but lack the perfor- dictate the maximum permissible thrust levels. mance to provide high-efficiency, large AV maneuvers required for orbital insertion. Bipmpel- Liauid Rockets for UDper Stape - and Smcecraft lant systems are attractive because they can provide Propulsion Svstems all three functions with one higher performance sys- tem, but they are more complex than the historic solid In a liquid rocket system, propellants are stored rocket and monopropellant combined systems. as liquids in tanks and fed on demand into the com- Dual-mode systems are integrated monopropel- bustion chamber by gas pressurization or a pump. lant and bipropellant systems fed by common fuel Bipropellant engines chemically react a fuel and an tanks. These systems are actually hybrid designs that oxidizer, and monopropellant engines catalytically use hydrazine ("4)as a fuel for high-performance decompose a single propellant. Bipropellant engines bipropellant engines; i.e., nitrogen tetroxidehydra- deliver a .higher Zsp but involve additional system zine (N204/N2H4), and as a monopropellant with complexity and cost. Table 2 shows liquid rocket conventional low-thrust catalytic tbrusters. The N204 engines currently in use for upper stages or PSs on feeds into the bipropellant engines and the mono- spacecraft. For up-to-date and more detailed propellant thrusters from a common fuel tank. In this information, contact the respective developers. manner, they can provide high Zsp for long AVburns at high thrust €?om a single propulsion system; e.g., Electric Propulsion apogee circularization, and reliable, precise, mini- mum-impulse bums by the monopropellant thrust- Electric propulsion (FP) uses externally provided ers for attitude control and tight pointing. An electrical power, either from the Sun (converted additional capability to enhance dual-mode propul- through photovoltaic solar arrays-100% to date) or sion is the development of a bimodal-thrust device, from nuclear and thermodynamic conversion ther- which can operate in a bimodal manner, either as a mal engines (to accelerate the working fluid to pro- simple catalytic-monoprop&llant thruster or as a high- duce useful thrust). For example, in an ion engine, performance, bipropellant thruster, known as the an electric field accelerates charged particles that exit secondary combustion augmented thruster (SCAT) at high velocity. Alternatively, in a magnetoplasma- shown in Figure 1. dynamic thruster, a current-carrying plasma In the propulsion system selectionprocess, prac- interacts with a magnetic field, resulting in abrentz tical consideration may restrict the propellant choices acceleration to expel the plasma. 2 thruster and power processor. (The masses of the into the future for a variety of medium to high propellant subsystem, gimbals, and other mission energy propulsion functions. specifics are not included.) Pulsed plasma thrusters (PPTs) are inherently Resistojets have been used for North-South pulsed devices that operate at -847-s ZSp. They were stationkeeping and orbital insertion of communica- built in the Applied Physics Laboratory at Johns tion satellites in the United States (U.S.) and for or- Hopkins University and have successfully main- bital control and attitude control system (ACS) tained precision control of three NOVA spacecraft functions on Russian spacecraft, respectively. Pro- for many years. PPTs feature very small (14x10"5Ns) pellant temperatures are fundamentally determined impulse bit capability, use of a solid propellant by material limits in resistojets, which implies mod- (Teflon@by DuF'ont@), and the ability to operate at est (propellant-specific) maxima for Zsp of -300 s near constant performance over large power ranges. for the 0.5- to 1-kW class resistojets. An improved version PPT that operates at =1,200-s Resistojets have several desirable features, Zse (Table 3) was developed under NASA leader- including (1) values of thrust and power far higher ship, and a flight test was recently c!onducted on the than other EP options, due to their high efficiencies Earth Observer 1 spacecraft that very successfully and modest Zsp; (2) the lowest EP system dry masses, demonstrated propulsive ACS. The characteristics primarily due to the lack of a requirement for a power of PPTs will likely limit their power operating range processor; and (3) uncharged (benign)plumes. These to under a few hundred watts and, as suggested by features will continue to make resistojets attractive Table 5, they have large specific masses. Within their for low to modest energy applications, especially operating capability, however, PITSpromise a com- where power limits, thrusting times, and plume im- bination of low power, high ZSp, and a small impulse pacts are mission drivers. In addition, resistojets can bit that is unique. Based on recent successes on flight operate on a wide variety of propellants, which leads vehicles, it is expected that PPTs' use for ACSs and to their proposed use as a propulsion and waste gas for modest energy AV applications in small space- management concept on the International Space craft where the power and thrust limitations of PPTs Station (ZSS) and as an Earth-orbit insertion system are acceptable and,desirable. (operated on hydrogen). Hall effect thrusters
Details
-
File Typepdf
-
Upload Time-
-
Content LanguagesEnglish
-
Upload UserAnonymous/Not logged-in
-
File Pages10 Page
-
File Size-