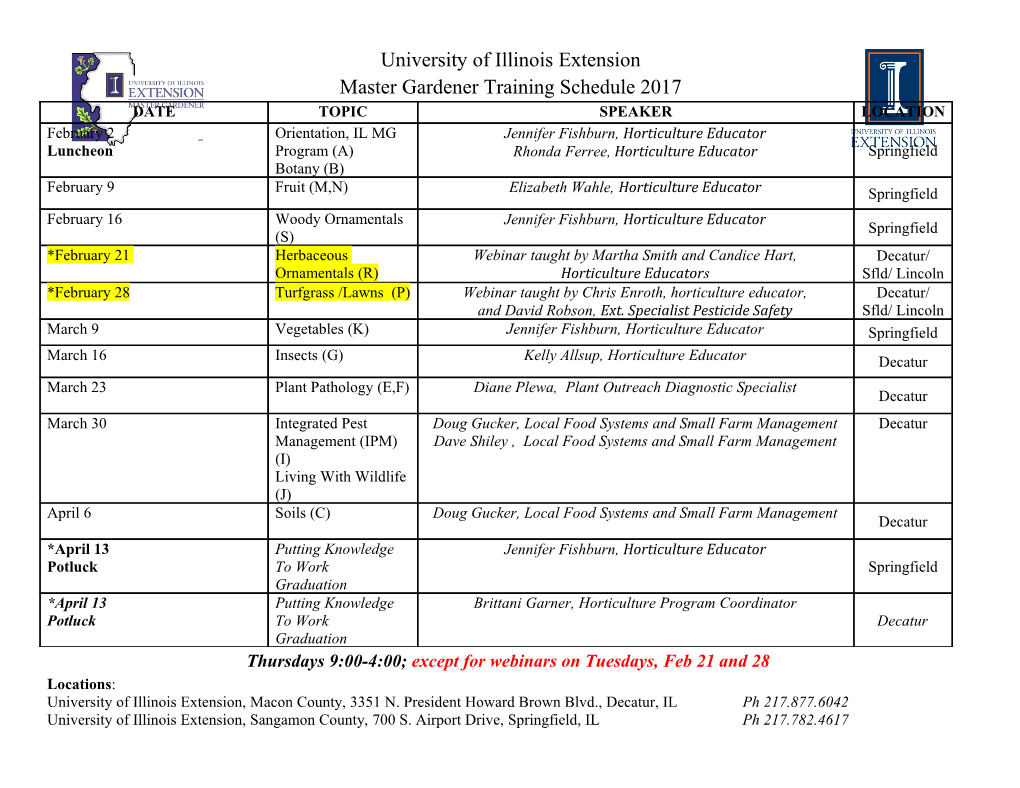
Spin of Planetary Probes in Atmospheric Flight SPIN OF PLANETARY PROBES IN ATMOSPHERIC FLIGHT RALPH D. LORENZ Lunar and Planetary Lab, University of Arizona, Tucson, AZ 85721, USA. Email: [email protected] Probes that enter planetary atmospheres are often spun during entry or descent for a variety of reasons. Their spin rate histories are influenced by often subtle effects. The spin requirements, control methods and flight experience from planetary and earth entry missions are reviewed. An interaction of the probe aerodynamic wake with a drogue parachute, observed in Gemini wind tunnel tests, is discussed in connection with the anomalous spin behaviour of the Huygens probe. Keywords: Spin, planetary probe, atmosphere, attitude control, parachute 1. INTRODUCTION The design of planetary probes is a complex matter, with many that makes meteors shine), this fraction of typically a few interesting technical challenges to which a variety of solutions percent is much more manageable. Were the entry body to be exist, e.g. [1, 2]. The purpose of this paper is to discuss some sharp-nosed, it would be more stable aerodynamically, but the aspects of the spin of probes in planetary atmospheres, and in heat loads on the nose would be unbearably intense. particular to summarize the available experience on the topic. Although it may seem a fairly straightforward matter, the con- Because heat shielding is generally heavy, it is desirable to trol of spin appears to be a rather arcane and poorly-docu- only need protection on one side of the vehicle although some mented aspect of entry and descent. Further, as evidenced by lesser shielding is still required on the back side, to protect the recent and as yet unexplained reversal of the spin of the against recirculating flow and against radiation from the hot Huygens probe during descent [3], it is not easy to get right. wake. This in turn requires the orientation at entry to be con- trolled (so-called ‘3-axis’ stabilization), by active attitude con- Before focusing our attention on spin during planetary de- trol with thrusters (as done with the Apollo capsules and the scent, a few remarks about spin prior to and during planetary Viking landers on Mars), by passive weathercock stability, or entry are appropriate. by spin-stabilization. 2. SPIN We do not consider 3-axis control further here. As for weathercock stability some Russian entry probes [1] to the 2.1 Spin Prior to Entry planet Venus had a spherical or egg shape, with heat shield material all around. The centre of mass was offset from the Many vehicles sent into the atmospheres of other planets (or centre, giving a preferred orientation. A more obvious exam- indeed back into the Earth’s atmosphere from above) have used ple of this type of stability was NASA’s two Deep Space 2 spin for stabilization. The most obvious and common require- (DS-2) Mars Microprobes [4], a pair of tiny (4 kg, 30 cm) ment is to maintain a desired orientation of the vehicle for entry shells designed to hit the ground at some 200 m/s and hypervelocity entry. bury penetrators into the ground in 1999. These lightweight entry shells had their penetrator payloads placed as far for- The kinetic energy of an object in orbit or on an interplan- ward as possible, giving the system a very strong weather- etary trajectory (with a velocity of perhaps 7 km/s – Mach 25 – cock stability. In fact, the penetrator nose was made of in the first case, or up to 50 km/s in the second) is formidable. tungsten (a metal with the remarkably high density of 19 Even in the former case, this energy is comparable with or times that of water!) for the express purpose of moving the exceeds the latent heat of evaporation of most materials. Thus centre of mass as far forward as possible for aerodynamic in order for the probe not to melt or evaporate, most of this stability. Vehicles in general must have some static stability energy must be dissipated somewhere other than the spacecraft. to remain pointing forwards: spin gives additional stiffness to keep angular excursions in the presence of disturbing The approach usually used is to make the probe or at least its torques to a minimum level. The cases above may be thought heat shield a blunt body, with a large radius of curvature. This of, then, as similar to the spin-stabilized cases that follow, blunt shape causes a strong shock wave in the hypersonic flow: but just with a nominally zero rate of spin! the relative airflow is decelerated (leading to conversion into heat) in this shockwave, rather than at the heat shield itself. 2.2 Generation of Spin and Separation While some fraction of the kinetic energy is still transferred to the body by convection and radiation (the shock layer will often The generation of spin on a planetary entry probe is usually glow brightly or even brilliantly – this is after all the process performed at, or just before, the moment of separation from the 1 Ralph D. Lorenz delivery vehicle. A number of technical approaches are there- fore possible, with spin being imparted either by the separation mechanism, or by spinning the delivery vehicle before separa- tion. An additional complication is to separate any electrical cabling – in some cases this is done with a pyrotechnic guillo- tine, but other approaches are sometimes used as we discuss below, with missions in roughly chronological order. The Pioneer Venus probes (Fig. 1) neatly exploited spin from their carrier spacecraft: the dispersed aim points at differ- ent locations were achieved [5] by releasing the probes from the carrier at precisely-controlled times, such that the probes were slung off with a desired sideways component of velocity (Fig. 2). The Large probe was ejected forwards along the bus spin axis. The three small probes were released tangentially from the bus, spinning at 48 rpm to provide the desired transverse delta-V to give entry at dispersed sites (Day, Night and North). Each probe unwound two weights on 2.4 m cables to despin to 15 rpm for entry. Although they did not reach Mars (or even escape Earth) the small stations of the Mars 96 mission were to be spin-stabilized for entry. At the time of separation from the orbiter the stations were to be spun up to approximately 12 rotations per second [6]. “There is also a kind of a wing in the rear end of the Station Fig. 1 The Pioneer Venus multiprobe spacecraft being prepared helping in stabilising the motion during the entry”. for launch at Kennedy Space Center. The ‘bus’ spacecraft spun; the large probe was simply pushed out along the spin axis of the The Galileo probe was released along the angular momen- spacecraft, while the small probes (of which two are visible) are tum vector of the Galileo orbiter, which was spun up to 10.4 released to fly out sideways using the rotation of the bus to achieve rpm prior to release. No additional spin was imparted during the desired dispersion. (NASA) the separation. Mars Pathfinder and the Mars Exploration Rovers (Fig. 3) were released from their launchers at 12 +/- 2 rpm (the space- craft/cruise stage having been slowed from 70 rpm to 12 rpm by yo-yo despin) and despun to 2 rpm for cruise. Obviously, to be stable in a spin orientation, the spin axis moment of inertia must be larger than that of the other two axes. With their cruise stages attached, these three vehicles were dynamically stable by having a moment-of-inertia ratio of 1.27. Interestingly, the sensitive accelerometers on-board recorded a slight periodic signature (Fig. 4) before Pathfinder encountered the atmos- phere of 20 or 30 micro-g amplitude (e.g. [7]). The period of the signal was 110s – comparing this with the 30s rotation period and the inertia ratio confirms that this is a slight nutation signal: ωobs = (1-Izz/Ixx)ωspin. The Genesis sample return capsule [8] was spun up to 15 Fig. 2 Illustration of the PV multiprobe releasing the large probe rpm prior to separation, a rate also used for the similar Stardust (top right) and later the three small probes. The lateral release capsule [9]. These entry bodies would experience aerodynamic (note the curved arm to the right of the carrier spacecraft) provided instabilities and so required substantial gyroscopic stiffness to the desired aimpoint distribution. prevent angle-of-attack excursions during the hypersonic entry (NASA, artwork by Paul Hudson) heating phase. The Earth return capsule for the Hayabusa mis- sion, on the other hand, has a centre of mass that is much further forward yielding more stable flight characteristics, and thus can In both the two European-led missions to date, Huygens and spin at a more leisurely 1 rpm [10]. Beagle 2, spin was generated at separation by means of a spin- eject device. In essence the spring forces to cause separation The spin rate on the Genesis capsule was effected (after were canted in a spiral to produce a couple that gives a spin pyrotechnic devices first severed the two cable bundles con- around a vector nominally aligned with the separation direc- necting the capsule with the bus) by spinning the main bus to 10 tion. This of course leads to an equal and opposite rotational rpm, re-orienting for release, and a further spin-up to 15 rpm. impulse on the ‘mother’ spacecraft. The Beagle 2 spacecraft Six push-off springs give a separation delta-V of 0.9 m/s [8] at [11] was spun at 14 rpm with a separation delta-V of 0.3 m/s, an altitude of ~63,000 km and the bus performed a divert using a very compact spin-eject device or SUEM (Spin-Up and manoeuvre 22 minutes later to avoid Earth impact.
Details
-
File Typepdf
-
Upload Time-
-
Content LanguagesEnglish
-
Upload UserAnonymous/Not logged-in
-
File Pages10 Page
-
File Size-