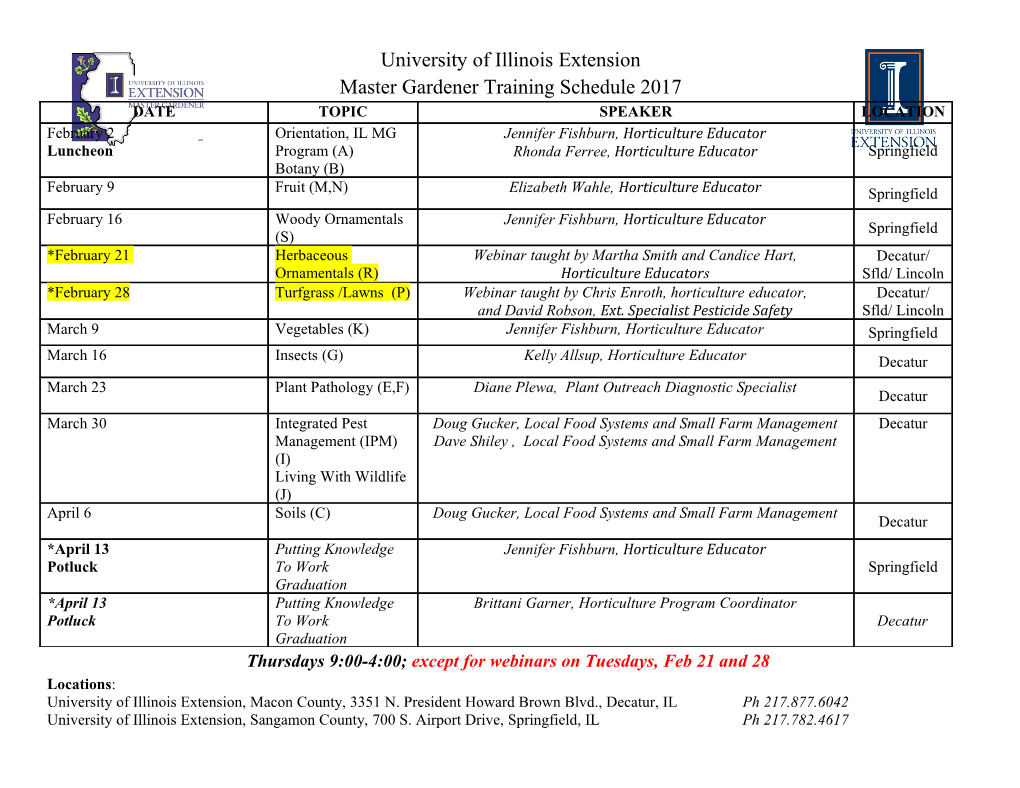
Harnessing Biotechnology: A Practical Guide Biotechnology is increasingly proving its ability to address chemical industry challenges. An engineering-focused approach — bioengineering — is vital to successful industrial application Steve Weiss Genomatica IN BRIEF WHOLE-PROCESS DESIGN EXTEND TECHNO- ECONOMIC ANALYSIS RETHINK ECONOMICS BIOENGINEERING IS DIFFERENT EVALUate SERVICES CLOSING THOUGHTS FIGURE 1. At Cargill’s biorefinery in Blair, Neb., companies such as Corbion, Evonik and Natureworks produce bio-based products or those undertaking overall process panediol biologically, after DuPont deter- design and implementation for in- mined that bio-based production would cost termediate and specialty chemicals, less than chemical routes to that product. Fthe incorporation of biotechnology- BASF SE (Ludwigshafen, Germany; www. based processes has become a viable op- basf.com) produces vitamin B2 (riboflavin) tion for the chemical process industries (CPI). biologically and has replaced its established Bioprocesses have the potential to deliver chemical route. Lysine is another example of benefits in cost, quality and sustainability. a large-volume chemical produced biologi- Many well-known chemicals are already cally. And 1,4-butanediol (BDO) is the next produced biologically. For example, Cargill bio-based commercial process on the ho- Inc. (Minneapolis, Minn.; www.cargill.com) rizon: BASF and Novamont Sp.A. (Novara, produces lactic acid for polymer applications Italy; www.novamont.com) have licensed at petrochemical scale at its Blair, Neb. site technology from the author’s company to (Figure 1) using a proprietary, low-pH, yeast- produce BDO biologically. Note: Chemical Engineering based fermentation process. DuPont Tate & This article is not intended as an introduc- is the exclusive publisher and Lyle Bioproducts Co. (Loudon, Tenn.; www. tion to biology, nor a recap of bioprocess ad- distributor of this article. duponttateandlyle.com) produces 1,3-pro- vantages or case studies (see Chem. Eng., 38 CHEMICAL ENGINEERING WWW.CHEMENGONLINE.COM APRIL 2016 November, 2015, p. 40). Rather, the article TABLE 1. BETTER PROCESS PERFORMANCE THROUGH is intended as a practical guide for how to WHOLE-PROCESS THINKING think about, plan for, and integrate biopro- cess technologies into chemical production “Bio-centric” approach “Whole-process” approach facilities. To do this, the article highlights sim- Focus: Maximize microorganism performance Focus: Design microorganism and overall pro- (for example, titer, rate and yield) cess to minimize total production cost, meet ilarities and differences between bioprocess all specifications and maximize operational and conventional chemical process designs, robustness equipment and operations, while strongly Priority: Laboratory-scale strain and fermenta- Priority: Choose microorganisms and meta- emphasizing cost economics and overall tion development is prioritized, supported by bolic pathways that are compatible with the process analysis. computational tools and iterative strain de- lowest-cost operating conditions (for example, sign, testing and metric optimization pH, temperature, aerobic versus anaerobic, co-product value). Design the microorganism Principle 1: Whole-process design for the process, not the other way around A well-known idiom states: “begin with the Process design: Process is designed sub- Process design: Pay close attention to manag- end in mind.” This suggestion — in the cur- sequently to fit the needs, capabilities and ing process impurities (raw material residuals, rent context, designing a process with the limitations of the microorganism metabolic byproducts, chemical reaction prod- whole system in mind — is appropriate. ucts) that could otherwise increase capital and operating costs, or compromise product The first part of our framework recognizes quality a key idea: chemical engineers had it right Process performance: Performance in the Process performance: Develop the microor- all along. Chemical engineers have decades laboratory may not be realized at large scale ganism and process at laboratory scale under of experience in designing and optimizing due to overlooked, scale-dependent param- anticipated large-scale conditions (time and integrated, large-scale processes to convert eters. Or, high performance at large scale may temperature profiles, mixing times, pressures, raw materials into useful products. In its best require special (expensive) operating condi- recycles). Characterize key process sensitivi- tions, be more sensitive to process upsets ties. Demonstrate the whole process at an ap- application, considerable effort is invested (less robust), more subject to contamination propriate scale to document its performance upfront, in the form of conceptual designs, by foreign microbes (plant shutdown), or incur with engineering data. Use those data as the even before the associated experimental higher downstream processing costs basis of detailed plant design program is launched. Downstream processing: Downstream pro- Downstream processing: Microorganism is By contrast, many of the headlines as- cessing is not addressed until the late stages designed for the process, to enable least of development (when the microorganism expensive operating conditions (includes pH, sociated with the rise of biotechnology- and fermentation are nearly finished). It is aerobic versus anaerobic, fermentation time based processes have focused on specific the responsibility of downstream processing and so on) technologies, tools and advances. Among to identify and solve any problems that have the favorite topics are the tools for design- arisen upstream. This invariably adds cost. ing microorganisms (sometimes referred to Plant: Large-scale plant is designed and built Plant: The whole-process approach translates informally as “bugs”). For the purposes of to accommodate the laboratory-scale process directly to design of large-scale plant, mini- mizing changes and added costs this article, the specific biology is not impor- tant — these engineered microorganisms can be thought of as fancy, biology-based “super-catalysts” that can perform multiple We refer to this whole-process discipline unit operations, such as all the steps needed as bioengineering. This approach better to convert sugar into a desired target chemi- captures the intimate intertwining of, and co- cal with high specificity and minimal byprod- optimization across, multiple disciplines. ucts. While the progress has been impres- While everyone can surely appreciate — sive in using new biological tools to shorten and even marvel at — the continued ad- the timelines to engineer an organism suit- vances in specific parts of the biology toolkit, able for a proof of concept, the reality is that the best practice is to take a whole-process commercializing a bio-based technology is view when exploring how to harness bio- not just about the bug. based processes as a component of a plant Instead of thinking about bio-based pro- construction project. Product customers cess design as a linear sequence (first, de- may also marvel at the science underlying sign the bug, then figure out the unit opera- a particular microorganism, but ultimately, tions to best separate and purify its output, they are really looking for ways to lower and then loop back to tune the design of the costs, raise product quality and improve the microorganism), a better approach is to “co- sustainability profile. develop” and “co-optimize” the microorgan- ism in conjunction with the overall process. Principle 2: Extend TEA Table 1 illustrates the differences between The second principle in evaluating and de- these two approaches — the “bug-first” ap- ploying biotechnology is to apply one of the proach is designated as “bio-centric” in the most favored tools from conventional pro- table, and the co-optimization approach is cess technology: techno-economic analy- designated as “whole-process.” sis (TEA). CHEMICAL ENGINEERING WWW.CHEMENGONLINE.COM APRIL 2016 39 TABLE 2. CAPITAL COSTS PER TON OF PRODUCT FOR CONVENTIONAL AND feedstock price for a bio-based process. BIO-BASED PLANTS (EXAMPLES) The first line to draw is where total produc- Conventional Bio-based Comparison tion costs are equal for the two competing process technologies. The region on one 100,000-ton/yr plant $300 million total $230 million total Bio is 23% lower $3,000 per ton $2,300 per ton side of that line shows all the feedstock price combinations where one process 50,000-ton/yr plant $200 million total $140 million total Bio is 30% lower $4,000 per ton $2,800 per ton technology is lower cost; and the other side of that line shows the price combinations Source: Genomatica estimates, based on industry analyst data, company data and discussions where the other process is favored. Next, with chemical producers. Assumes scaling exponent of 0.6 for conventional and 0.7 for bio-based at these ranges of capacity map historical data points, and see which process technology would have delivered Many readers are likely already familiar with lower costs given actual feedstock prices TEA, and may use it to better understand over time (as shown by the Xs and circles the overall economics of potential process in Figure 3). Lastly, draw additional lines to designs for conventional chemistry-based highlight the relative feedstock pricing when processing. The idea is to apply the same one process delivers, for example, a 25% concepts of TEA to bio-based processes cost advantage. By doing so, you can get a (see sidebar,
Details
-
File Typepdf
-
Upload Time-
-
Content LanguagesEnglish
-
Upload UserAnonymous/Not logged-in
-
File Pages6 Page
-
File Size-