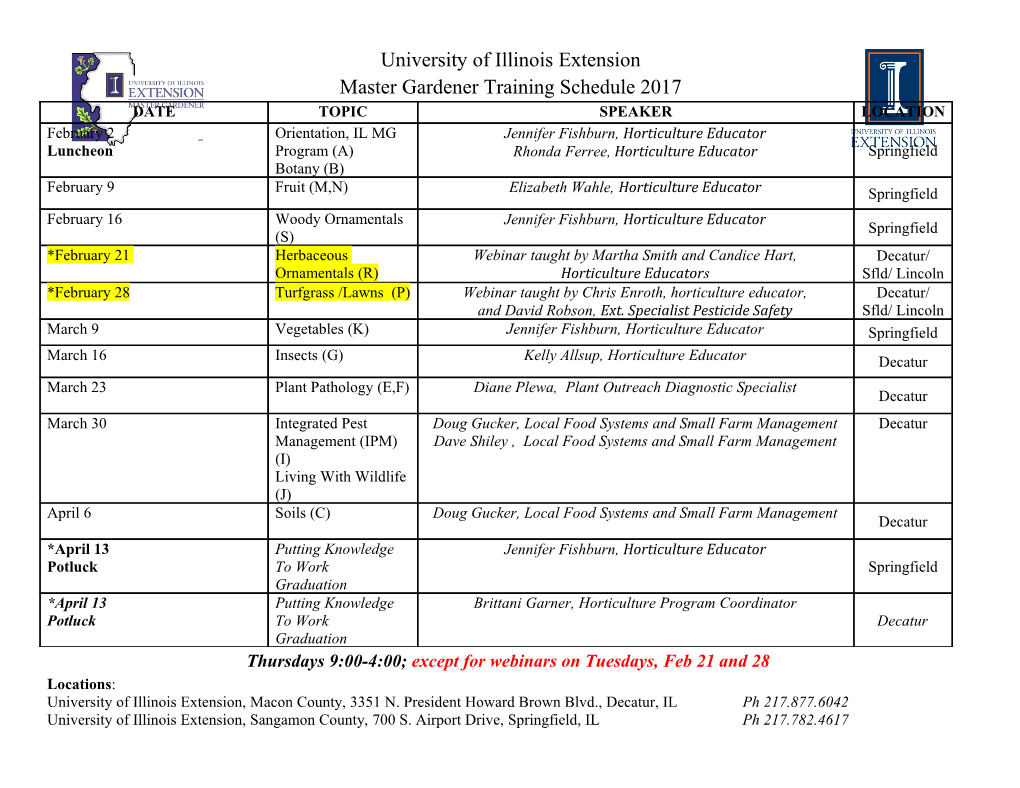
A HIGH ENERGY PROTON-ELECTRON-POSITRON COLLIDING BEAM SYSTEM* C. Pellegrini, LNF, Frascati; J. Rees, B. Richter, and M. Schwartz, SLAC, Stanford; D. Mahlt and A. Sessler, LBL, Berkeley (Presented by J. Rees) Abstract the electron ring so that it fits into the same housing re­ sults in an e-p c. m. energy of 65 GeV which is equiva­ A system of two intersecting storage rings of gross lent to 2100 GeV electrons striking stationary protons. 1 radius 260 m, one for protons and the other for electrons The desi~ luminosity is set at about 1032 cm-2 sec- and positrons, is described. The maximum energy of the for both e+ -e- and e-p collisions based on achieving a stored proton beam is 70 GeV, that of the electron and satisfactory counting rate in some of the more interest­ positron beams 15 GeV; so the center-of-mass energies ing experiments which can be done with the facility, and are 65 GeV for e-p collisions and 30 GeV for e+ - e- col­ allowing an experimental program of extraordinary lisions. The performance of the system is determined by breadth. the RF power available for the electron and positron beams and by the incoherent beam-bea!lllimit on trans­ This paper describes SOme of the physics which can verse beam density. With an available RF power for the be done with this double-ring system and presents the beams of 2.7 MW, the system yields design luminosities results of our efforts to design the rings. The experi­ in the vicinity of 1032 cm-2 sec-1 in both modes of oper­ ments described below represent only a small fraction of ation. Some important physics experiments and their the physics which can be done with the rings. The topics yields are discussed. discussed were chosen because they are of particular interest now, and they give some idea of the scope of the 1. Introduction possible experimental program. The resulting ring pa­ rameters show that the luminosity specified can be Experiments using the beams from electron and pro­ reached with conventional magnet, RF and injection tech­ ton accelerators have been the driving force in the pursuit niques. We have not attempted to optimize the design for of knowledge in elementary particle physics. Looking minimum cost, although we think the parameters are back over the last twenty years we see that what we know reasonable. of particle structure comes principally (but not exclu­ 1.1 Physics with 65 GeV (c.m.) Proton-Electron sively) from experiments done at the electron machines, Collisions while our knowledge of particle spectroscopy comes prin­ cipally (but not exclusively) from experiments at proton 1.1.1 Inelastic electron scattering. Inelastic elec­ machines, and our knowledge of particle dynamics comes tron scattering experiments have generated a great deal from experiments at both kinds of machines. The conven­ of interest in the past few years. Recent experiments at tional electron and proton machines - and now the SLAC have revealed a large inelastic cross section, electron-positron and proton-proton colliding beam ma­ giving support to the notion of a grainy substructure in the chines - exist in a kind of symbiotic relationship which proton. With the electron-proton colliding beams, stud­ allows complementary experiments, and is crucial to the ies of the reaction e+p-e+X can be extended to momentum vitality of high energy physics. The maximum center-of­ transfers and inelasticities far beyond anything available' mass (c. m.) energies of the generation of electron stor­ from other machines. age rings now nearing completion lie in the vicinity of 6 GeV. This energy range matches the c.m. energy range It is customary to describe the inelastic scattering of the present generation of conventional accelerators in terms of electrons incident on stationary protons. In (BNL, CERN, SLAC) which lies around 7 GeV. However, these terms, the e-p ring gives an equivalent electron with the advent of the NAL proton synchrotron soon to laboratory energy of about 2100 GeV. The kinematic pa­ operate with a c. m. energy of 20 to 30 GeV (200 to 500 rameters of interest are q2, the square of the invariant GeV proton beams), and with the CERN ISR operating at four-momentum transferred by the electron and v, the c.m. energies of up to 56 GeV, the proton machines have energy loss of the electron in the system where the tar­ now far outstripped both the electron-positron storage get prot~n is at rest. The maximum value of q2 is 4000 rings and the conventional electron accelerators. (GeVIc) and the maximum value of v is 2100 GeV. This can be compared to a proposed IJ.-p inelastic scattering We have been studying the feasibility of greatly in­ experiment at NAL, which gives a maximum practical q2 creasing the c. m. energy available for electron-positron of 40 (GeVIc)2 and v of 150 GeV with the apparatus colliding beams, and making use of the colliding-beam planned. technique to study electron-proton interaction at c. m. en­ ergies which do not seem accessible with conventional To get an idea of the yields which might be expected accelerators. To this end, we have set our design goals in the e-p ring, we will extrapolate from SLAC energies, in relation to the high energy physics experiments which employing the most generally used theoretical model seem most important, and we have designed a storage (scaling). In bins of dq2/ q2 and dv Iv of ±10%, we find a ring facility to meet those goals. We have chosen a c.m. yield of about 5 eventslday at the maximum momentum energy of 30 GeV for e+ - e- interactions which matches transfer of 4000 (GeV/c)2. For v=1000 GeV in the proton the c. m. energy of NAL at 500 GeV, and represents a rest system, and q2::::1000 (GeV/c)2, we get about 20 large extrapolation in energy from the present generation events per day. These events are quite easy to detect. of e+ -e- rings. Storing the protons in a ring using con­ For example, the case of q2::::1000 (GeVIc)2 and v::::1000 ventional magnets and having the same circumference as GeV gives a final electron of about 20 GeV energy coming out at nearly 900 to the storage ring beams, and there are *Work supported by the U.S. Atomic Energy Commission. few background sources which can give such large trans­ verse momenta. tpermanent address: CERN, Geneva. - 154 - 1. 1.2 Photoproduction. Strictly speaking, there is Indeed, there are models which predict even larger than no pure photoproduction in electron-proton collisions. pointiike cross sections at high energies. However, the srecial case of inelastic electron scatter­ 1.2.2 Inclusive hadronic reactions. With 30 GeV ing with q2«me can be looked at as a two-step process ­ first, the radiation of a real photon, and second, the in­ colliding beams it is possible to study the inclusive reac­ tion e++ e--4 h+ "anything" for large energy and trans­ teraction of this photon to produce hadrons. The verse momentum deposited on the one detected hadron h. Weizsacker-Wi1liams approximation gives the equivalent Some of the fundamental questions to be studied in this photon-beam intensity coming from this very low q2 in­ ~hoto­ process are the energy dependence and yields for differ­ elastic scattering, and the effective luminosity for ~,A, production reaction is Q'(k) =0. 07x 1032 (&/k)cm- soo-l, ent hadrons such as 1f, K, N, etc., and the relation of the structure functions to the analogous structure func­ where k is the photon energy. In terms of photons inci­ tions for deep inelastic scattering from protons. Besides dent on protons at rest, the maximum photon energy is these general studies, specific tests of C invariance, SU3 2100 GeV. invariance, etc. are possible. Estimates all suggest large cross sections and comfortably observable event Counting rates for photoproduction processes range rates for these processes. For example some parton or from very large to very small. For example, the total point-constituent models suggest event rates of from 1 to photon cross section for hadron production is estimated 25 events/hr for a luminosity of 1032/ cm2 sec. to give a yield of 700 (&/k) per second independent of k, while a single p-meson photoproduction gives a yield of 1.2.3 Two-body final states. Present experiments 70 (&/k), also independent of k. A typical small photo­ on two-body (exclusive) hadron production are not suffi­ production cross section would be that for single-pion ciently accurate and do not cover a wide enough range in production (yp-4 7l+N). Measurements made up to 20 GeV energy to permit a reliable extrapolation to the storage indicate the energy dependence for this cross section to ring energies considered here. On theoretical grounds, be given by k-2, where k is the photon energy in the however, we would ~xpect hadron form factors falling at proton rest system. In the range of equivalent photon least as fast as 1/E , which would give unobservable energy from 200 GeV to 500 GeV, where photoproduction counting rates at E = 15 GeV with planned luminosities for experiments will complement experiments done with pi pairs, nucleon pairs, etc. However, many other two­ hadron beams at NA L, the rate for even this small cross body channels are of great interest and should have large section varies from 15 (&/k) to 2.5 (&/k) events per counting rates.
Details
-
File Typepdf
-
Upload Time-
-
Content LanguagesEnglish
-
Upload UserAnonymous/Not logged-in
-
File Pages6 Page
-
File Size-