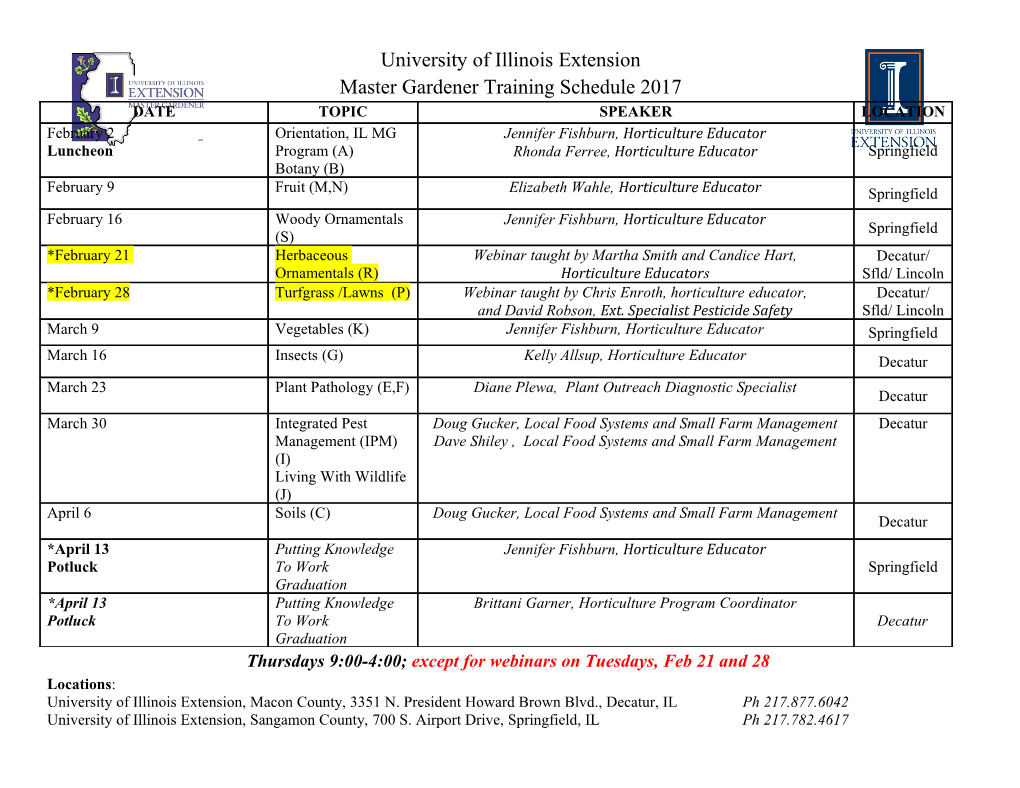
cells Review The Chromatin Response to Double-Strand DNA Breaks and Their Repair Radoslav Aleksandrov, Rossitsa Hristova, Stoyno Stoynov and Anastas Gospodinov * Roumen Tsanev Institute of Molecular Biology, Bulgarian Academy of Sciences, Acad. G. Bonchev Str. 21, 1113 Sofia, Bulgaria; [email protected] (R.A.); [email protected] (R.H.); [email protected] (S.S.) * Correspondence: [email protected]; Tel.: +359-2-979-3680 Received: 15 June 2020; Accepted: 4 August 2020; Published: 7 August 2020 Abstract: Cellular DNA is constantly being damaged by numerous internal and external mutagenic factors. Probably the most severe type of insults DNA could suffer are the double-strand DNA breaks (DSBs). They sever both DNA strands and compromise genomic stability, causing deleterious chromosomal aberrations that are implicated in numerous maladies, including cancer. Not surprisingly, cells have evolved several DSB repair pathways encompassing hundreds of different DNA repair proteins to cope with this challenge. In eukaryotic cells, DSB repair is fulfilled in the immensely complex environment of the chromatin. The chromatin is not just a passive background that accommodates the multitude of DNA repair proteins, but it is a highly dynamic and active participant in the repair process. Chromatin alterations, such as changing patterns of histone modifications shaped by numerous histone-modifying enzymes and chromatin remodeling, are pivotal for proficient DSB repair. Dynamic chromatin changes ensure accessibility to the damaged region, recruit DNA repair proteins, and regulate their association and activity, contributing to DSB repair pathway choice and coordination. Given the paramount importance of DSB repair in tumorigenesis and cancer progression, DSB repair has turned into an attractive target for the development of novel anticancer therapies, some of which have already entered the clinic. Keywords: DNA damage response; double-strand DNA break repair; non-homologous end joining; homologous recombination; chromatin dynamics in DNA repair; synthetic lethality; PARP inhibitors; cancer; anticancer drug therapies 1. Introduction DNA carries all hereditary genetic instructions dictating and regulating cellular functions and fate. Following the discovery of its notable double helical structure, DNA was considered a fairly stable molecule. However, it did not take much time before scientists discovered that DNA is subject to the damaging effects of multiple mutagens that generate a remarkable diversity of harmful lesions [1]. On the one hand, the emergence of mutations in DNA, which generate new alleles or change the position or the number of the genes in the genome, is the driving force of evolution since these mutations are the “raw material” upon which evolution exerts selection [2]. However, on the other hand, most mutations are deleterious and disrupt the function(s) of the damaged genes, leading to various pathologies. Accumulation of DNA lesions has been implicated in cell cycle arrest, cell senescence and death, aging, tumorigenesis, diverse developmental defects, and neurodegenerative diseases [3,4]. It has been estimated that every nucleated cell in the human body suffers approximately 70,000 DNA lesions every day, and external sources of mutation only increase this number. Taking into account the huge number of mutations arising constantly and their potential to inflict detrimental effects, it is logical that for billions of years of evolution, cells have been developing mechanisms for the detection and repair of DNA lesions [2,3,5]. Cells 2020, 9, 1853; doi:10.3390/cells9081853 www.mdpi.com/journal/cells Cells 2020, 9, 1853 2 of 45 Research in many laboratories over the years revealed an intricate network of hundreds of proteins and protein complexes organized in discrete pathways that recognize and repair specific types of DNA lesions [6]. These DNA repair pathways are precisely coordinated with the progression of the cell cycle and function in the immensely complicated and dynamic context of the chromatin [7–9]. The importance of the DNA damage response (DDR) is demonstrated by the fact that almost all types of cancer exhibit some kind of DNA repair deficiency [10] and mutations in genes coding for essential DNA repair factors are often associated with considerably increased predisposition to cancer and/or premature aging [3]. In this review, we discuss the mechanisms that eukaryotic cells employ to repair double-strand DNA breaks (DSBs)—the most toxic type of DNA lesions. Especially, we focus on the elaborate network of dynamic chromatin modifications and extensive chromatin remodeling, which facilitate and regulate DSB repair pathway choice and implementation. Being of paramount importance to the process of tumorigenesis, DSB repair mechanisms and DSB repair-associated chromatin changes have turned into an attractive target for the development of novel therapeutic strategies for treating cancer patients [11,12]. We consider the numerous tactics that have emerged to target DSB repair pathways with an emphasis on the therapeutic paradigm of synthetic lethality. 2. Tying up Loose Ends—Overview of DNA Double-Strand Break Repair Pathways 2.1. Sources of Damage Mutagens can broadly be divided into endogenous and exogenous by their source of origin. Endogenous mutagens are generated inside the cells of the organism and are generally chemical in nature comprising Reactive Oxygen Species (ROS) generated in the oxidative reactions of the metabolism and other highly reactive byproducts of certain enzymatic reactions such as formaldehyde formed during histone demethylation [13,14]. Exogenous mutagens include a large cohort of DNA-damaging compounds and high energy electromagnetic radiations such as UV-, X-ray, and gamma-radiation that are able to damage DNA either directly or indirectly [3]. It has been shown that ROS generated during oxidative metabolism are among the primary sources of double-strand DNA breaks in eukaryotic cells. Oxygen-containing radicals such as hydroxyl radicals ( OH) and superoxide anion radicals ( O ) are extremely reactive particles capable of • • 2− damaging molecules in their immediate vicinity, including DNA, creating a significant DSB burden if their production is left unattended [13,15,16]. In addition, tumorigenesis requires the activation of oncogenes, which in turn stimulate the uncontrolled proliferation of the cells. To achieve this, oncogenes increase DNA replication origin firing leading to replication stress due to altered nucleotide levels and replication fork speed. Replication stress may cause replication fork stalling and collapse, generating copious amounts of DSBs during the S phase of the cell cycle [17,18]. Moreover, increased replication rates could lead to transcription-replication conflicts resulting in DNA breaks that fuel genomic instability [19]. Both DNA and RNA polymerases lead to the generation of superhelical strain in DNA during transactions such as DNA replication, repair, and transcription. This tension is relieved by the action of a specific group of enzymes dubbed topoisomerases, which induce the formation of either single- or double-strand breaks in DNA to relax the strained DNA molecules [20]. Lesions that are present on DNA molecules during DNA-based processes increase the probability for abortive topoisomerase reactions that could lead to the formation of DSBs [2,20,21]. Certain cellular processes include the controlled formation of DNA breaks. For example, V(D)J and immunoglobulin heavy chain class switch recombination entail the formation of DSBs in order for B- and T-lymphocytes to form their extensive repertoire of antibodies or cell surface antigen receptors [22]. Meiotic crossing over, one of the main processes creating genetic diversity during sexual reproduction, also involves the regulated induction of DNA breaks in order for homologous chromosomes to exchange genetic material in gametogenesis [23]. Cells 2020, 9, 1853 3 of 45 Environmental factors such as ionizing radiation and certain chemicals can induce the formation of excessive numbers of DSBs. High energy X-ray and gamma-radiation can lead to DSBs either directly or indirectly through the generation of ROS. Low doses of radiation received during procedures such as medical imaging or even air travel can inflict damage and increase the basal levels of DSBs [3]. Mutagenic chemical compounds contained in cigarette smoke (benzo[a]pyrene) or biological toxins such as the aflatoxins that are present in the spores of Aspergillus fungi covalently bind to DNA and induce the formation of DSBs following replication fork stalling and collapse [24,25]. Acetaldehyde produced during ethanol oxidation can induce inter-strand DNA crosslinks (IPCs) and DNA-protein crosslinks (DPCs) that may lead to DSB formation during DNA repair or transcription [14,26]. Having in mind the complexity of DSB induction and the consequences organisms suffer from untimely and mutagenic DSBs, cells must have evolved specific molecular mechanisms to cope with the genomic instability posed by double-strand DNA breaks. 2.2. Pathways for Double-Strand DNA Breaks Repair Four different DSB repair pathways have been described thus far (Figure1). Although these pathways are very specific in their mechanisms and outcomes, they share many common proteins and are substantially interdependent [18]. The two main DSB repair pathways are the classical non-homologous end joining (c-NHEJ) pathway and homologous recombination (HR). c-NHEJ proteins are rapidly assembled
Details
-
File Typepdf
-
Upload Time-
-
Content LanguagesEnglish
-
Upload UserAnonymous/Not logged-in
-
File Pages45 Page
-
File Size-