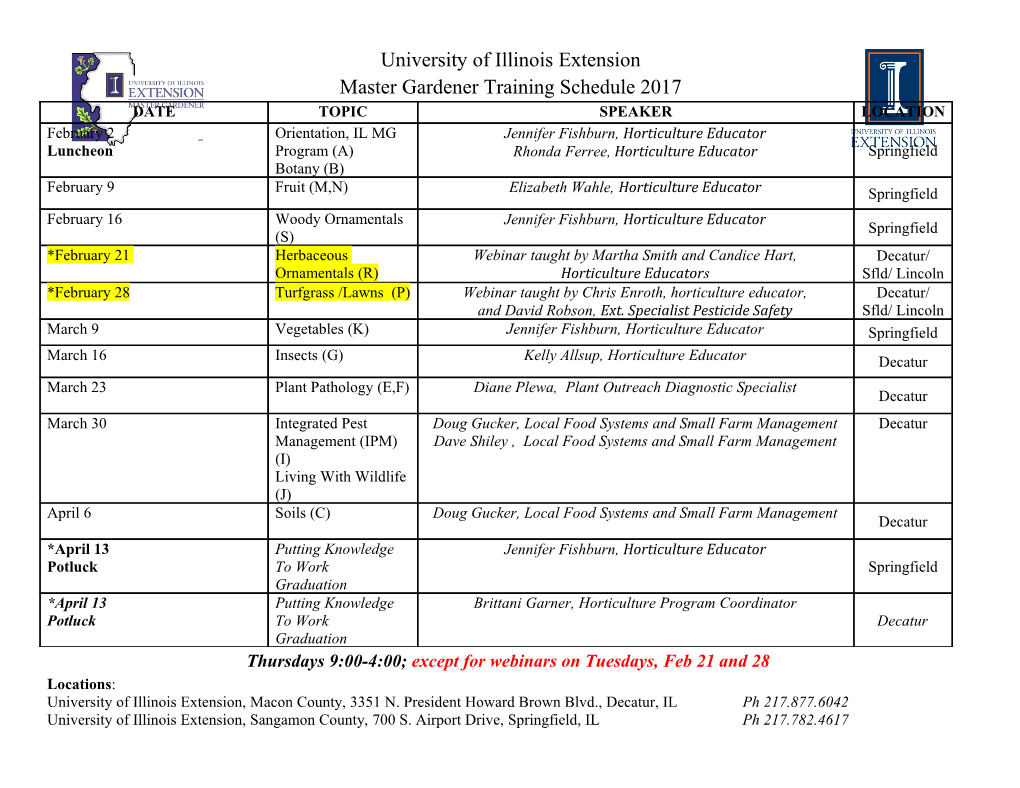
1 Vanadium Redox Flow Batteries for the storage of electricity 2 produced in wind turbines 3 Esperanza Menaa, Rubén López-Vizcaínoa, María Millánb, Pablo Cañizaresb, Justo 4 Lobatob, Manuel A. Rodrigo*b. 5 aDepartment of Chemical Engineering, Institute of Chemical & Environmental 6 Technologies, University of Castilla-La Mancha, Campus Universitario s/n, 13071 7 Ciudad Real, Spain 8 bDepartment of Chemical Engineering, Faculty of Chemical Sciences & Technologies, 9 University of Castilla-La Mancha, Campus Universitario s/n, 13071 Ciudad Real, Spain 10 11 *Corresponding Author 12 Prof. Manuel Andrés Rodrigo. Phone +34 902 204 100 Ext 3411; Fax: +34 902 204 13 130; e-mail: [email protected] 14 15 SUMMARY 16 In this work, accelerated degradation charge-discharge tests have been applied to 17 compare the performance of a bench-scale vanadium redox flow battery (VRFB), when 18 charged under galvanostatic conditions and under the highly variable conditions of 19 current produced by wind turbines. Wind speed patterns applied for the VRFB charge 20 were obtained during three representative days in winter, in Ciudad Real (Spain). The 21 accumulated and delivered charge capacities and the different efficiencies (coulombic, 22 voltage and energy) were analyzed during three charge and discharge cycles. The 23 conversion of the different vanadium species during the charge-discharge cycles, 24 indicated that the operation mode had a strong influence on the performance of the VRFB 25 and helped to explain the charge profiles obtained. Although, similar efficiencies and 1 1 charge/discharge capacities were found, the VRFB operated in wind-charging mode 2 performs slightly worse than the VFRB operated in galvanostatic mode. Increased 3 crossover of vanadium species in the negative electrolyte compartment explains the 4 differences found. Nevertheless, it can be concluded that this type of technology seems 5 to be promising for the storage of electricity produced by wind turbines. 6 7 KEY WORDS 8 Redox Flow Battery; Vanadium; Renewable Energy Source; Wind Turbine, Accelerated 9 Degradation 10 11 12 13 14 15 16 17 18 19 20 21 22 23 24 25 2 1 1. INTRODUCTION 2 The use of renewable energy sources has a great number of advantages, among 3 which two should be highlighted: the absence of pollutant emissions and the possibility 4 of using them inexhaustibly. However, there are some disadvantages related to these 5 green energies, above all, to the use of the wind as energy resource. These are its 6 instability, unpredictability and fluctuations, due to changes in the weather conditions. 7 Because of these drawbacks, the use of energy storage systems is required in order to be 8 able to maintain an uninterruptable electricity supply as it should be the required for 9 houses, hospitals or official facilities [1-3]. In other applications, such as environmental 10 powering of electrochemical devices, this necessity is not as important because of the 11 large dynamic time constants of the processes which contribute to make softer the effect 12 of fluctuations on the performance of the application [4-9]. 13 There is a great variety of energy storage systems, among which it could be 14 highlighted compressed air, pumped hydro, superconducting magnetic energy, flywheels 15 or electrochemical systems [10, 11]. Well-known lead-acid batteries are included in the 16 electrochemical systems group. This technology can be used for the storage of renewable 17 sources providing-energy. However, the environmental problems related to the use of this 18 technology represent an important drawback [12, 13]. Taking into account this 19 background, in the recent years some studies have been published about a new technology 20 for the efficient storage of energy: Redox Flow Batteries [12-15]. A flow battery consists 21 of two usually aqueous electrolytes that contain two redox couples and are pumped 22 through an electrochemical cell in which chemical energy is converted to electricity [16]. 23 These batteries can be divided into different groups, according to the phases of the 24 electroactive species involved in the system and the couples involved in the redox system 25 reactions [14, 17, 18]: liquid-RFB, where chemical energy is stored in the electrolyte; 3 1 solid-RFB, where chemical energy is stored in an active material on the electrode plate; 2 and hybrid-RFB, with solid and gaseous species involved in the redox couples. Among 3 the liquid-RFB, it is worth to mention the iron-chromium[19, 20], all vanadium (VRFB) 4 [21, 22] and other vanadium-based systems [23-26], bromine-polysulphide [27] and all 5 uranium [28] and neptunium [29, 30] and non-aqueous electrolytes system [31-33]. The 6 solid-RFB includes soluble lead-acid [34, 35], zinc-nickel [36, 37] and zinc-manganese 7 dioxide [38]. Finally, the hybrid-RFB includes several zinc-based systems [10, 39-42], 8 vanadium-air [43], organic redox couples [44, 45] and lithium flow batteries [46, 47]. 9 In this work, a redox flow battery using the different oxidation states of the 10 vanadium as energy storage system is evaluated (Vanadium Redox Flow Battery, VRFB). 11 This type of batteries was proposed in the mid-1980s by the Professor Skyllas-Kazacos’s 12 group, belonging to the University of New South Wales (UNSW) [48-52], obtaining 13 patent in 1986 (AU Patent 575247-1986). Since then, the development of this technology, 14 even for the industrial application, has been world-wide spread [53-55]. Reactions 15 involved on the performance of the all vanadium redox flow batteries include equation 1 16 on the positive electrode and equation 2 on the negative electrode, being the overall 17 reaction shown in equation 3. Discharge 2 18 VO2 2H e VO H2O E0=1.00 V (1) Charge Discharge 2 3 19 V V e E0=-0.26 V (2) Charge Discharge 2 2 3 20 VO2 V 2H VO V H2O E0=1.26 V (3) Charge 21 Taking into account these considerations, the main objective of this work is to 22 evaluate the application of vanadium redox flow battery system (VRFB) as storage of the 4 1 energy provided by a wind turbine. The most relevant property of this renewable energy 2 source is its highly random value. For this reason, a new study of the VRFB behavior 3 under a discontinuous charge produced by wind turbine has been developed. The obtained 4 results have been compared with a similar test where a galvanostatic charge process has 5 been applied using the same total electric charge. In both kind of tests, a STERION LT105 6 membrane was used to separate the positive and negative electrode compartments. This 7 membrane allows the correct performance of the VRFB, but it degrades quickly due to 8 highly corrosive electrolyte. This strategy allows us to simulate an accelerate degradation 9 in the performance of the VRFB carrying out very low number of charge/discharge cycles 10 2. MATERIALS AND METHODS 11 A scheme of experimental setup is shown in Figure 1. It is composed of a wind 12 turbine that supplies the renewable energy, the potentiostatic unit, which supplies the 13 energy according to the renewable energy profiles to the VRFB and the VRFB setup itself. 14 2.1. Wind turbine 15 Electricity production profile obtained from a Bornay 600 wind turbine (Bornay 16 Aerogeneradores, Alicante, Spain) was used. The turbine is composed of two fiberglass 17 blades (1.0 m long) controlled by an electronic regulator of 24 V and 30 A. The regulator 18 acts on the turbine as an automatic overspeed-governing system to keep the rotor from 19 spinning out of control in very high winds. In-house computer software (developed with 20 Labview, National Instruments) was used to control the process and monitoring the actual 21 wind speed. Further details can be found elsewhere [4, 5]. 22 2.2. Vanadium Redox Flow Battery 23 The experimental device used in this work has been supplied by Baltic Fuel Cells 24 and it is composed of two differentiated modules: a quick connect fixture (qCf-RFB) that 25 allow the compression of the internal components of the commercial RFB cell (cF40- 5 1 RFB) using a centric clamping vise. The fore of the vise is directly applied to the active 2 area, enabling fully reproducible test conditions. In addition, qCf-RFB provides a 3 temperature regulation by pumping of a thermal fluid (Huber P20.275.50) located in 4 external thermostatic bath (Huber). cF40-RFB is a commercial electrochemical cell with 5 an active area of 40 cm2 (w=52 mm, h=77mm). Only graphite and plastic materials are 6 exposed to the electrolyte. 7 A commercial vanadium electrolyte, supplied by Golden Energy Fuel Cell Co. Ltd. 8 (China), was used in this work. It had a 2 M vanadium concentration (VO2+ in the positive 3+ 9 electrolyte and V in the negative electrolyte) in 3 M H2SO4. At the beginning of every 10 single test, positive and negative electrolyte tanks were filled up with 200 mL of the 11 corresponding solution. A peristaltic pump (Heidolph Pumpdrive 5001) was used to 12 deliver electrolyte to the RFB cell at a constant flow rate (20 mL min-1). The negative 13 electrode tank reservoir (containing V2+, which is an instable specie, and V3+) was purged 14 with a nitrogen stream to avoid the oxidation of the V2+ due to the presence of oxygen 15 during the charge and discharge experiments. 16 Carbon soft felt, provided by SGL Group was used as electrodic material both in 17 the cathode and in the anode. Carbon felt pieces were submitted to a previous treatment 18 with boiling 1 M NaOH before being used as electrodes, in order to increase the 19 hydrophilicity of the material.
Details
-
File Typepdf
-
Upload Time-
-
Content LanguagesEnglish
-
Upload UserAnonymous/Not logged-in
-
File Pages29 Page
-
File Size-