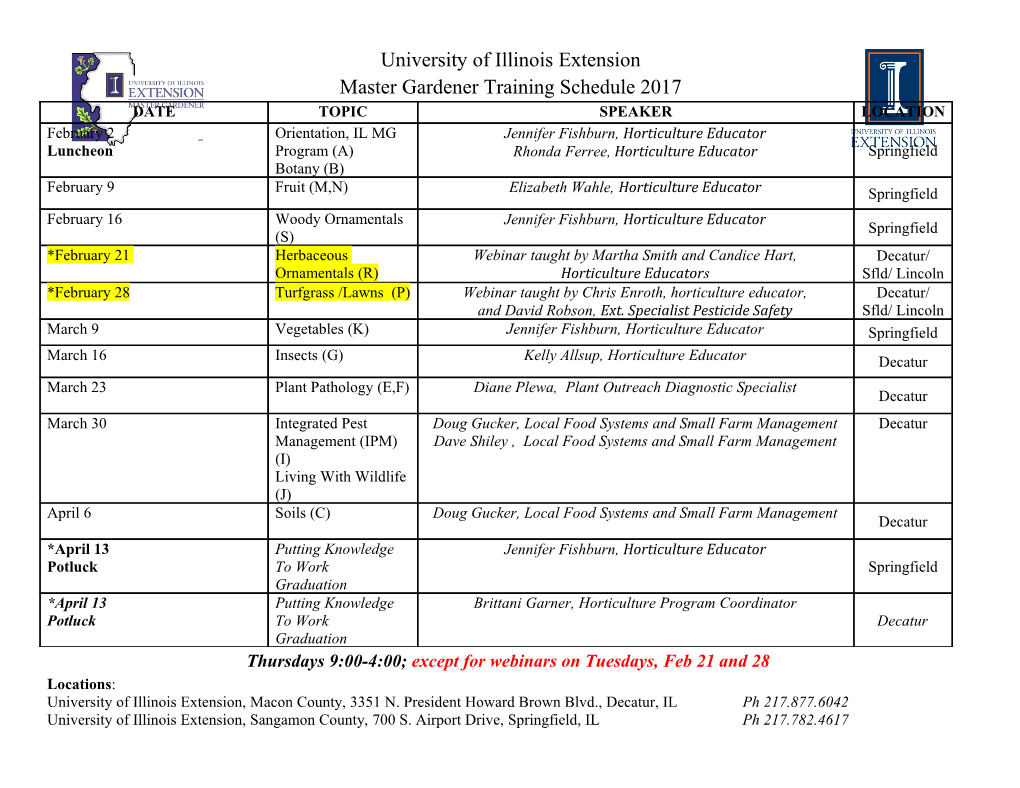
VOLUME 46 JOURNAL OF APPLIED METEOROLOGY AND CLIMATOLOGY FEBRUARY 2007 Predicting Tephra Dispersion with a Mesoscale Atmospheric Model and a Particle Fall Model: Application to Cerro Negro Volcano MARC A. BYRNE Department of Geography, University of South Florida, Tampa, Florida ARLENE G. LAING National Center for Atmospheric Research,* Boulder, Colorado CHARLES CONNOR Department of Geology, University of South Florida, Tampa, Florida (Manuscript received 8 October 2005, in final form 9 May 2006) ABSTRACT Models of volcanic ash (tephra) fallout are increasingly used to assess volcanic hazards in advance of eruptions and in near–real time. These models often approximate the wind field using simplistic assump- tions of the atmosphere that do not account for four-dimensional variations in wind velocity. The fifth- generation Pennsylvania State University–National Center for Atmospheric Research Mesoscale Model (MM5) is used to improve forecasts of tephra dispersion. MM5 is a 3D model that can predict circulations in areas with sparse meteorological observations and complex terrain, such as volcanic plateaus. MM5 is applied to the 1995 eruption of Cerro Negro, Nicaragua. Validation of MM5 is achieved by comparing the simulated winds with rawinsonde observations. Estimates of diffusivity and particle settling velocities are used in conjunction with MM5-generated wind fields to forecast the major axes of the tephra dispersion. The predicted axes of dispersion derived from the MM5 winds approximate very closely the observed bilobate tephra accumulation and tephra plumes observed in satellite images. MM5 winds provide far more accurate spatial and temporal forecasts than do the wind assumptions that had been used previously to assess Cerro Negro tephra hazard. 1. Introduction 1994; Scott et al. 1995; Newhall and Punongbayan 1996). Tephra often causes water contamination, and Tephra, commonly known as volcanic ash, is defined airborne tephra can cause adverse effects on respiration as airborne volcanic ejecta of any size (Tilling et al. and irritation of eyes and nose (Latter 1989; Baxter 1987). It is a frequent product of volcanic eruptions. 1990). Deadly mudslides known as lahars, made of Volcanoes create numerous natural hazards that pose a tephra and water, often pick up more debris as they risk to society, but wind-driven tephra threatens far travel, and can move over 10 m sϪ1 (Iverson 1997). larger areas than any other volcanic hazard. Tephra Tephra that reaches the stratosphere affects climate— deposited on roofs can easily cause building collapse for example, lower surface temperatures following the (Wolfe 1992). Tephra can severely damage airplanes, 1991 eruption of Mount Pinatubo (Robock 2002). automobiles, and electrical equipment (Casadevall Because tephra poses numerous hazards, volcanolo- gists have modeled its dispersion for just over two de- cades. Although tephra models have increased in com- * The National Center for Atmospheric Research is sponsored plexity over time, their meteorological component has by the National Science Foundation. been conventionally simple, often out of necessity. As demand grows for real-time prediction and scenario- Corresponding author address: Arlene Laing, NCAR/MMM, based hazard assessment of tephra deposition, im- P.O. Box 3000, Boulder, CO 80307. provements to the atmospheric component of tephra E-mail: [email protected] modeling are essential. There is an important need for DOI: 10.1175/JAM2448.1 © 2007 American Meteorological Society 121 Unauthenticated | Downloaded 10/06/21 12:57 PM UTC JAM2448 122 JOURNAL OF APPLIED METEOROLOGY AND CLIMATOLOGY VOLUME 46 FIG. 1. (a) The 1995 eruption of Cerro Negro viewed from the northwest (photograph by B. Hill). (b) Distribution of tephra-fall deposits for November–December 1995 Cerro Negro eruption, based on field measurements by M. Kesseler (University of Geneva). Isopachs are in centimeters. Dashed line indicates the uncertainty in the location of the 0.1-cm isopach. Ruled and stippled patterns show the lava and cone. Topographic contours (100 m) are derived from 93-m-resolution digital elevation data. Coordinates are in universal transverse Mercator (UTM) coordinates, zone 16, WGS-84 spheroid. Inset map shows locations of major Nicaraguan volcanoes (from Hill et al. 1998). volcanic eruption hazard mapping at many volcanoes generation Pennsylvania State University–National around the world under different wind regimes. Many Center for Atmospheric Research (NCAR) Mesoscale volcanoes are located in countries that do not have the Model (MM5) are applied to the eruption of Cerro resources required to undertake detailed hazard map- Negro (Fig. 1), Nicaragua, that occurred on 19 Novem- ping. Atmospheric models in combination with tephra ber–6 December 1995. dispersion models can provide very valuable informa- tion to satisfy this requirement. a. Tephra modeling This study incorporates winds from a high-resolution atmospheric model to predict the major axes of tephra To understand tephra plumes better, many volca- dispersion and deposition. Winds produced by the fifth- nologists have numerically simulated volcanic eruptions Unauthenticated | Downloaded 10/06/21 12:57 PM UTC FEBRUARY 2007 B Y R N E E T A L . 123 This assumption may be made for simplicity’s sake, or because wind data are not easily accessible and, in many cases, are incomplete. Wind data are extrapo- lated sometimes from a single observation from a weather station tens of kilometers away (Barberi et al. 1990; Macedonio et al. 1994; Hill et al. 1998). This is a serious problem because the simplistic wind assumption neglects a major impact on deposition location. Much of the research on tephra dispersion involving atmospheric models has focused on aviation interests. The Volcanic Ash Forecast and Transport and Disper- sion model (VAFTAD, Heffter and Stunder 1993); the Canadian Emergency Response Model (CANERM), a FIG. 2. A weak tephra plume advected by wind (from Bona- 3D Eulerian model (Simpson et al. 2002); and “PUFF” donna and Phillips 2003). Here X represents the distance that a w (Searcy et al. 1998) are designed for real-time tracking particle is transported by wind during particle fall and is propor- tional to the ratio between wind speed and particle terminal ve- of volcanic ash clouds. These three models are intended locity, Hb is the level of neutral buoyancy, and Ht is the top of the for tracking middle-to-upper-tropospheric airborne plume. In vertical fallout, a particle falling from the current base ash. They do not predict low-tropospheric dispersal. at a point A will reach the ground at a point B1; if a plume Atmospheric dispersion models applied to surface develops in a wind field, a particle is likely to reach the ground at tephra accumulation have not been as widely investi- a point B2 depending on the wind velocity and the particle termi- nal velocity. gated as those used for aviation. Suzuki (1983) was the first to model tephra transport and deposition numeri- cally. He used a diffusion–advection equation for hori- (Suzuki 1983; Carey and Sigurdsson 1982; Connor et al. zontal particle motion in a temporally and spatially uni- 2001; Bonadonna and Phillips 2003). Physical assump- form wind field. The Suzuki model has been adjusted to tions are made about the eruption, eruption column, represent increasingly intricate volcanic eruptions and tephra plume. Some eruption parameters are esti- (Glaze and Self 1991; Armienti et al. 1988; Connor et al. mated. For example, satellite-estimated heights of 2001; Bonadonna et al. 2002; Bonadonna and Phillips eruption plumes can be prone to error (Tupper et al. 2003) and modified for parallel computation in the 2004). Geologists often attempt to reconstruct eruption “TEPHRA” model (Bonadonna et al. 2005). Although dynamics from ancient deposits. This activity is essen- TEPHRA is sophisticated in its representation of vol- tial in volcanic hazard assessment. However, it is not canic eruptions, it uses a single wind velocity profile as always clear which aspects of the deposit reflect volca- its input and assumes uniformed winds over each x–y nological conditions, such as eruption column height, domain for each altitude. and which aspects of the deposit can be explained by In a study similar to ours, Turner and Hurst (2001) meteorological conditions. used atmospheric and dispersion models to forecast Modeling tephra movement in the atmosphere is cru- tephra deposition from Mount Ruapehu, New Zealand. cial for modeling tephra accumulation. Once tephra They compared “ASHFALL” (Hurst and Turner particles in the eruption column have achieved their 1999), a simplified Suzuki model, with a combination of maximum height, their movement depends upon their the Regional Atmospheric Modeling System (RAMS) characteristics (density, shape, terminal fall velocity) and the Hybrid Particle and Concentration Transport and, very important, wind. For most eruptions, tephra model (HYPACT). Although HYPACT had merit in plumes are advected by winds and become more diffuse tracking tephra plumes, it was not suitable for quanti- with time and distance downwind (Bursik 1998). In fying tephra deposition depth because of shortcomings general, ballistic ejecta reach the ground very quickly, related to the distribution of particle fall velocities. whereas the finest tephra settles last (Fig. 2). Vertical However, the RAMS–HYPACT suite produced more wind shear can create irregular deposition patterns. Al- accurate forecasts than did ASHFALL and demon- though
Details
-
File Typepdf
-
Upload Time-
-
Content LanguagesEnglish
-
Upload UserAnonymous/Not logged-in
-
File Pages15 Page
-
File Size-