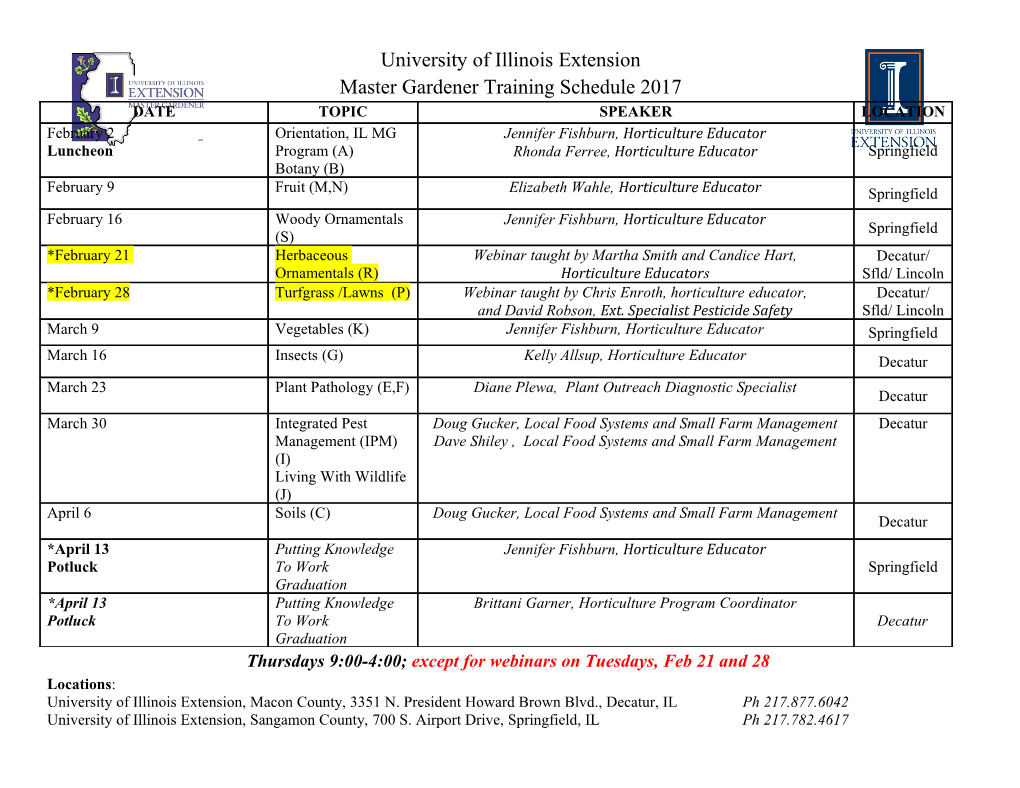
Accepted Manuscript Invited review Impact structures in Africa: A Review Wolf Uwe Reimold, Christian Koeberl PII: S1464-343X(14)00017-X DOI: http://dx.doi.org/10.1016/j.jafrearsci.2014.01.008 Reference: AES 1959 To appear in: African Earth Sciences Received Date: 11 July 2013 Revised Date: 15 January 2014 Accepted Date: 17 January 2014 Please cite this article as: Reimold, W.U., Koeberl, C., Impact structures in Africa: A Review, African Earth Sciences (2014), doi: http://dx.doi.org/10.1016/j.jafrearsci.2014.01.008 This is a PDF file of an unedited manuscript that has been accepted for publication. As a service to our customers we are providing this early version of the manuscript. The manuscript will undergo copyediting, typesetting, and review of the resulting proof before it is published in its final form. Please note that during the production process errors may be discovered which could affect the content, and all legal disclaimers that apply to the journal pertain. Dedicated to our students, collaborators and friends in Africa, without whose support we could not have successfully pursued our investigations of confirmed and possible African impact structures. Wolf Uwe Reimold Christian Koeberl Impact structures in Africa: A Review Wolf Uwe Reimold1,2 and Christian Koeberl3,4 1*Museum für Naturkunde Berlin – Leibniz Institute for Evolution and Biodiversity Research Invalidenstrasse 43, 10115 Berlin, Germany ([email protected]) 2Humboldt Universität zu Berlin, Unter den Linden 6, 10099 Berlin, Germany 3Department of Lithospheric Research, University of Vienna, Althanstrasse 14, 1090 Vienna, Austria ([email protected]) 4Natural History Museum, Burgring 7, A-1010 Vienna, Austria * Corresponding author: Wolf Uwe Reimold Museum für Naturkunde Berlin Invalidenstrasse 43 10115 Berlin Germany Tel. +49 30 2093 8470 / +49 173 778 1432 1 Table of Contents Abstract 1. Introduction 1.1 Fundamental importance of impact cratering 1.2 Beneficial impact 1.3 Why a special review in JAES dedicated to impact structures in Africa? 2. The Study of Impact Structures 2.1 The tools of impact cratering science 2.1.1 Remote sensing and geophysics 2.1.2 Geophysical data sets 2.1.3 Field work and drilling 2.1.4 Laboratory analysis 2.1.4.1 Petrography and petrology 2.1.4.2 Geochemistry 2.1.4.3 Geochronology 2.1.5 Experimental impact 2.1.6 Numerical modeling 3. The Impact Cratering Process 3.1 The three stages of impact cratering 3.2 Morphological considerations 4. Recognition Criteria for Impact Structures 4.1 Shock metamorphism 4.2 Impactites – a recommended nomenclature 4.3 Chemical tracers of extraterrestrial projectiles 5. The Terrestrial Impact Cratering Record 6. The Impact Record of Africa 6.1 Confirmed impact structures of Africa 6.2 Impact glasses and tektites 6.3 Spherule layers – remnants of distal impact ejecta 6.3.1 Neo-Archean spherule layers 6.3.2 Shock metamorphism and source craters 6.3.3 Numerical models 6.4 Proposed but not confirmed impact structures 6.5 Disproven candidates 7. Outlook 8. Acknowledgements 9. References 2 Abstract More than 50 years of space and planetary exploration and concomitant studies of terrestrial impact structures have demonstrated that impact cratering has been a fundamental process - an essential part of planetary evolution - ever since the beginning of accretion and has played a major role in planetary evolution throughout the solar system and beyond. This not only pertains to the development of the planets but to evolution of life as well. The terrestrial impact record represents only a small fraction of the bombardment history that Earth experienced throughout its evolution. While remote sensing investigations of planetary surfaces provide essential information about surface evolution and surface processes, they do not provide the information required for understanding the ultra-high strain rate, high- pressure, and high-temperature impact process. Thus, hands-on investigations of rocks from terrestrial impact craters, shock experimentation for pressure and temperature calibration of impact-related deformation of rocks and minerals as well as parameter studies pertaining to the physics and chemistry of cratering and ejecta formation and emplacement, and laboratory studies of impact-generated lithologies are mandatory tools. These, together with numerical modeling analysis of impact physics, form the backbone of impact cratering studies. Here, we review the current status of knowledge about impact cratering – and provide a detailed account of the African impact record, which has been expanded vastly since a first overview was published in 1994. No less than 20 confirmed impact structures are now known from Africa, but the 49 proposed, but not yet confirmed, possible impact structures contain at least a considerable number of structures that, from available information, hold the promise to be able to expand the African impact record drastically – provided the political conditions for safe ground-truthing will become available. The fact that 28 structures have also been shown to date NOT to be of impact origin further underpins the strong interest in impact in Africa. We hope that this review stimulates the education of students about impact cratering and the fundamental importance of this process for Earth – both for its biological and geological evolution. This work may provide a reference volume for those workers who would like to search for impact craters and their ejecta in Africa. Highlights: Comprehensive review of confirmed, proposed (possible) and disproven impact structures in Africa General introduction to impact cratering science, as well as to accepted recognition criteria for impact structures A comprehensive bibliography on impact cratering in general and impact related studies in Africa Keywords: Impact structures; Africa; impact crater record; shock metamorphism; projectile identification; terrestrial impact record 1. INTRODUCTION More than 50 years of exploration of the surfaces of planets, moons, asteroids, and even of comet nuclei, have firmly established the fundamental role that impact cratering has played as a planetary process and surface-modifying agent on nearly all solid bodies in the solar system (Fig. 1a-f), throughout its entire history of 4.56 billion years. The only body in the Solar System lacking an impact record is Jupiter‘s moon Io – likely because of recent resurfacing due to extensive volcanism. Accretion of planetary bodies today is understood to have been driven by continuous impact of ever larger particles and bodies – from tiniest dust grains to planetesimals (e.g., Taylor, 1992; Morishima et al., 2008; Hirashita, 2012). 3 However, impact is not purely a process of the distant past; in fact, several recent events have demonstrated that the danger of impact has very much persisted into the present. In July 1994 small fragments of comet Shoemaker-Levy 9 impacted successively into the atmosphere of the gas-giant planet Jupiter (Boslough et al., 1994) – impacts that were followed on TV by millions of humans. That these small, likely merely hundreds of meters sized, fragments packed gigantic punches was demonstrated impressively by the Earth-sized holes formed in the atmosphere of Jupiter. In the 1990s, the Pentagon released seismic records that proved that sizable impactors (up to several tens of meters in diameter) impacted onto Earth‘s surface repeatedly – albeit the recorded events took all place over the oceans and, thus, did not cause damage to humans. This and current efforts to safeguard Earth from catastrophic impact are, for example, discussed in Task Force on Potentially Hazardous Near Earth Objects (2000) or National Research Council (2009, 2010). In September 2007 a small bolide, not larger than a meter or two in size, impacted at Carancas in Peru, and formed a 14-m-diameter impact crater (Borovička and Spurný, 2008; Kenkmann et al., 2009a). This relatively small event did cause some concern by the local, rural population, but did not cause any bodily harm. However, even such a small event in a densely populated area (such as a metropolitan area) could have caused the loss of hundreds of lives. Not quite so dramatic, but nevertheless causing injury to 1 500 people and extensive damage to housing, are the consequences of the explosion of an originally (i.e., prior to impact) about 17 to 20 m wide meteoroid that exploded at a height of 23 km above the Russian city of Chelyabinsk (Urals) of 1 million inhabitants on 15 February 2013 (e.g., Borovička et al., 2013; Kohout et al., 2013). The explosion had an estimated magnitude of some 500 million tons of TNT. The 1908 Tunguska bolide exploded with a force of 3 to 5 megatons, but was only roughly four or five times larger than the Chelyabinsk bolide. More than 100 kg of meteorites have been recovered around Chelyabinsk, and a large piece of about 650 kg has been recently retrieved from the bottom of Lake Cherbakul. A popular review of the event was recently published by Durda (2013). Imagine if an explosion similar to Tunguska, which destroyed some 2000 km2 of forest, would today occur above a mega-city - the outcome would be an unbelievable disaster. In fact, meteor blasts in the atmosphere occur from time to time – some of remarkable magnitude essentially remaining unnoticed by the public: a 50 kiloton explosion took place over the Indonesian island of Sulawesi on 8 October 2009 (ibid). Durda reports that explosions of Chelyabinsk magnitude are thought to occur about once per century,
Details
-
File Typepdf
-
Upload Time-
-
Content LanguagesEnglish
-
Upload UserAnonymous/Not logged-in
-
File Pages281 Page
-
File Size-