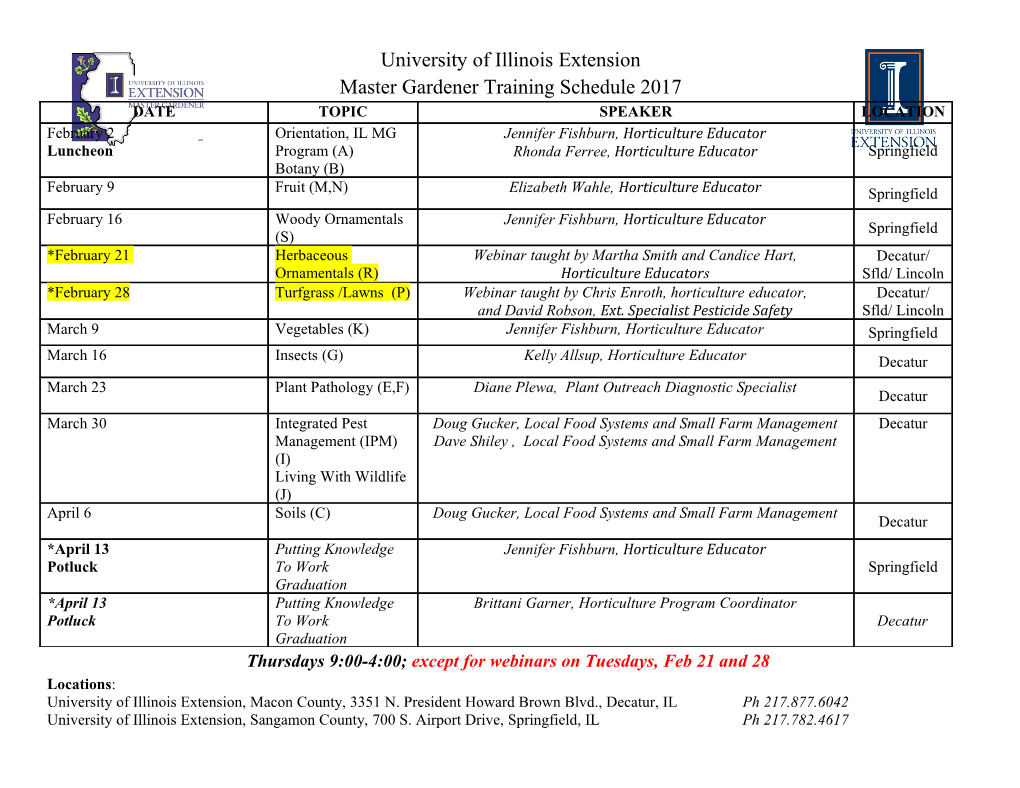
FORCES AND INTERACTIONS BETWEEN NANOPARTICLES FOR CONTROLLED STRUCTURES by PAUL R. MARK A dissertation submitted to the Graduate School-New Brunswick Rutgers, The State University of New Jersey In partial fulfillment of the requirements For the degree of Doctor of Philosophy Graduate Program in Materials Science and Engineering Written under the direction of Dr. Laura Fabris And approved by ____________________________________________ ____________________________________________ ____________________________________________ ____________________________________________ ____________________________________________ New Brunswick, New Jersey January, 2013 ABSTRACT OF THE DISSERTATION Forces and Interactions Between Nanoparticles for Controlled Structures PAUL R MARK Dissertation Director: Dr. Laura Fabris In recent years, structured nanomaterials have started to demonstrate their full potential in breakthrough technologies. However, in order to fulfill the expectations held for the field, it is necessary to carefully design these structures depending upon the targeted application. This tailoring process suggests that a feedback between theory and experiment could potentially allow us to obtain a structure as near optimum as possible. This thesis seeks to describe the theory and experiment needed to understand and control the interactions among nanoparticles to build a functional device for the efficient conversion of sunlight into energy. This thesis will discuss a simulation built from the existing theories explaining nanoparticle interactions and will present how its outcomes can be employed to describe real systems. The forces and dynamics of the nanoparticle system control the way their structure is formed. Thus, in order to understand and predict ii the formation of organized nanostructures, simulation of forces and dynamics and their corroboration with experimental results are necessary. These simulations will be extended to more complex systems, and the results will be used to provide a basis for the design of a specific nanoparticle structure, namely a linked linear chain. The envisioned application of the results achieved with the approach described is the design of a nanoparticle-based organic photovoltaic cell where linear chains of nanoparticles are tethered to the back of the device and then surrounded by a conducting polymer matrix to generate percolation pathways and improve light collection and scattering, and thus efficiency, of the device. To tether the chains in the cell, a foundation is needed to provide structure and control spacing. This foundation is designed and constructed by depositing gold nanoparticles on a substrate patterned using block- copolymer lithography to form a hexagonal array upon which the linear chains will be grown. iii Acknowledgement and Dedication I dedicate this thesis to my parents, George and Ann Mark, who have give me so much love and assistance through the years. I also dedicate it to my fiancé Kathryn Dorst, who has been by my side, helped me through all the rough parts of achieving this, and done so incredibly much for me. iv Table of Contents Abstract ii Acknowledgement and Dedication iv Table of Contents v List of Tables vi List of Illustrations vii 1. Introduction 1 1.1 History of Nanoparticles 1 1.2 Modern Uses of Nanoparticles 1 1.3 Desired Uses of Nanoparticles 2 1.4 Need for Designed Structures 4 1.5 Ways to Obtain the Desired Structure 5 1.6 Need for Simulation to Elucidate Structure 6 1.7 Statement of the Problem 7 1.8 Objectives 8 1.9 Method 9 References 11 2. Understanding nanoparticle assembly: A simulation approach to SERS-active dimers 12 Abstract 12 1. Introduction 13 1.1 Bottom-up Synthesis of Dimers 14 1.2 Modeling the Bottom-up Synthesis of Dimers 18 1.2.1 Forces 19 1.2.2 Nanoparticle Concentration 20 2. Methods 21 2.1 Theoretical Basis: Forces 21 2.1.1 Conversion of Potentials into Forces 24 2.2 Boundary Conditions 26 2.3 Kinetics of n-mer Formation 27 3. Results and discussion 29 3.1 Effect of Initial NP Concentration 29 3.2 Kinetics 36 3.3 Effect of Linker Concentration 38 4. Conclusion 41 References 43 3. Salt-induced Template-less 1D Assembly of Gold Nanoparticles 45 Abstract 45 1. Introduction 46 2. Basic Principles 48 3. Nanoparticle Interaction Model 50 4. Experimental Methods 51 5. Results and discussion 54 5.1 Modeling NP-cluster interactions 54 5.2 UV-Vis Analysis of assembly 59 5.3 SEM Analysis of Assembly 64 v 5.4 Stability of Linear Nanochains 67 6. Conclusion 69 Supporting Information 71 References 74 4. Large Ordered Arrays of Gold Nanoparticles by Block Copolymer Templating 76 Abstract 76 1. Introduction 77 2. Proposed Methodology 81 3. Methods 83 4. Results 84 Conclusions 87 Supporting Information 88 References 94 5. Conclusion 96 vi List of Tables Chapter 2 Table 1. Concentrations of NPs used in the simulations. 31 Table 2. Fitted second order rate constants corresponding to parameters in Equation 6, in units of L mol-1 s-1. 37 vii List of Illustrations Chapter 2 Figure 1. Cartoon representation of brush interpenetration (a) and compression (b) 22 Figure 2. Graphs of the radial distribution functions for (a) constant, (b) linear, (c) pseudo-homopolymer, (d) pseudotail and (e) 1/r2 functions. 23 Figure 3. Biphenyl-4,4’-dithiol (DBDT), the model linker used in the present work. 24 Figure 4. Time evolution of the interparticle separation (a, c, e) and time evolution of the number of dimers (b, d, f) averaged over 2500 steps in a system with an initial NP concentration equal to 4.8000 10-10 NP/nm3 (a,b), 7.6800 10-9 NP/nm3 (c,d), 1.2288 10-7 NP/nm3 (e,f). 33 Figure 5. Comparison of as-simulated and fitted MCMC kinetics for NP concentrations of 4.8000 10-10 NP/nm3 (a), 7.6800 10-9 NP/nm3 (b), and 1.2288 10-7 NP/nm3 (c). 35 Chapter 3 Figure 1. Cartoon representation of the process of nanochain assembly. The red area represents the region where an incoming nanoparticle is most likely to attach to the growing chain. 49 Figure 2. Methodology of sample preparation for in-situ UV-Vis measurement of NP assembly in a cuvette. 52 viii Figure 3. Diagram of the UV-Vis data fitting process. The data and the calculated fit, along with the major components of the fit are shown. The data and the calculated fit overlap and are thus not distinguishable in the graph. Components of the fit not treated here are described in the supplemental information. 53 Figure 4. Calculated center of mass force acting on a single 10 nm radius NP due to a dimer of the same NPs located at the origin. Regions in the core are repulsive, and in the shell are attractive. Scale of the forces is shown in the colorbar, units are Newtons of force. 55 Figure 5. Tangential force calculated acting on a single 10nm radius NP due to a dimer of the same NPs located at the origin. In the red/green region the force aligns the particles; in the blue region the force acts to dis-align the particles. Scale of the forces is shown in the colorbar, units are Newtons of force. 56 Figure 6. Calculated center of mass force acting on a single 10 nm radius NP induced by the presence of a trimer of the same NPs located at the origin. Regions in the core are repulsive, and in the shell are attractive. Scale of the forces is shown in the colorbar, units are Newtons of force. 58 Figure 7. Calculated tangential force acting on a single 10 nm radius NP due to a trimer of the same NPs located at the origin. In the red/green region the force aligns the particles; in the blue region the force acts to dis-align the particles. Scale of the forces is shown in the colorbar, units are Newtons of force. 59 ix Figure 8. Fit parameters for the time evolution of the UV-Vis spectra of suspensions of nanospheres induced to aggregate by the addition of salt. a) Scattering exponent, b) ratio of transverse peak amplitude to maximum for the transverse peak, c) ratio of longitudinal to transverse peak amplitude, d) longitudinal peak width. A decreasing scattering exponent indicates larger assemblies, while a decrease in the transverse peak amplitude and an increase of the transverse to longitudinal peak ratio indicate an efficient NP coupling. Smaller increases in the longitudinal peak widths can be interpreted as indicative of the formation of linear assemblies rather than large aggregates. 61 Figure 9. SEM micrographs of TEM grids coated with salt-assembled NPs: a) 4 mM, b) 20 mM, c) 40 mM salt at 10 minutes from mixing. The NP solution was aged for two weeks before collecting the SEM data. It can be observed that the level of assembly and the size of the structures increase with the increase in salt content. The black circles in the background are due to the presence of the TEM grid onto which the samples were deposited. 65 Figure 10. SEM micrographs of TEM grids coated with salt-assembled NPs: a) 4 mM, b) 40 mM salt at 30 minutes from mixing. The NP solution was aged for two weeks. 66 Figure 11. SEM micrographs of TEM grids coated with salt-assembled NPs @ 20 mM NaCl, 30 minutes from mixing. The NP solution was aged for two weeks. 67 Figure 12. Fit parameters from the UV-Vis spectra of salt induced aggregation and stabilization of nanospheres. Following dilution and an initial decrease in intensity, both the transverse and longitudinal peaks do not change as time progresses, x indicative of the fact that dilution effectively arrested the assembly. a) Transverse peak amplitude fit, b) longitudinal peak position fit. 68 Figure S1. Non-peak fit components.
Details
-
File Typepdf
-
Upload Time-
-
Content LanguagesEnglish
-
Upload UserAnonymous/Not logged-in
-
File Pages137 Page
-
File Size-