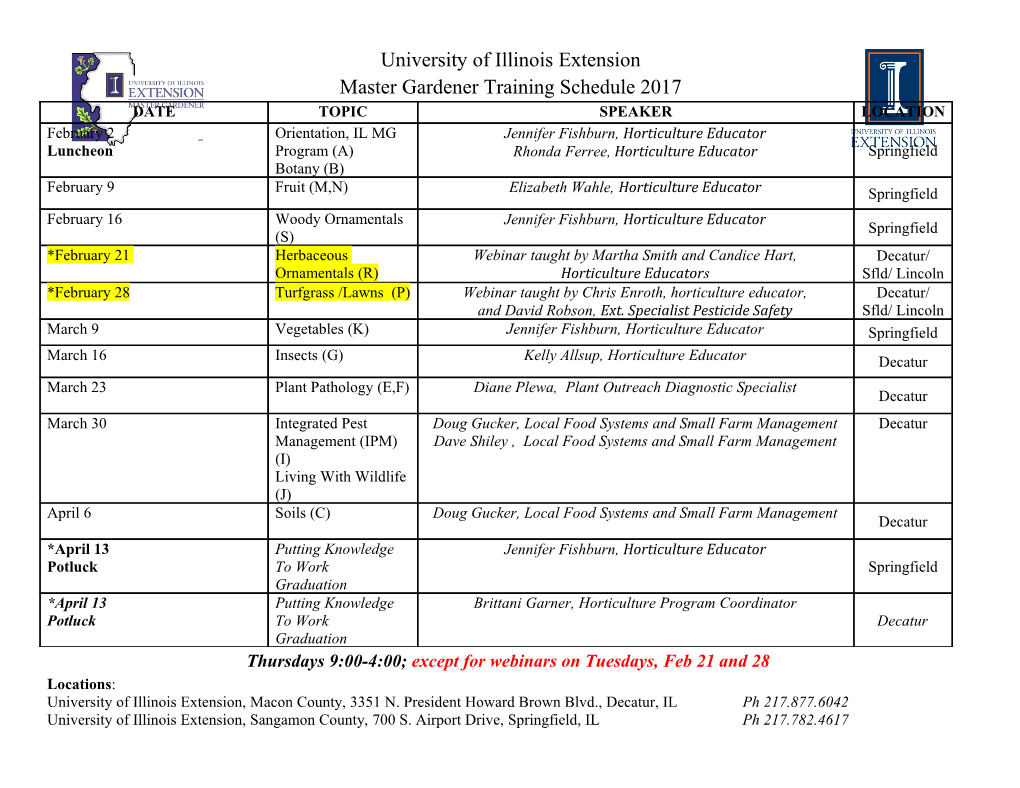
bioRxiv preprint doi: https://doi.org/10.1101/172130; this version posted January 14, 2018. The copyright holder for this preprint (which was not certified by peer review) is the author/funder. All rights reserved. No reuse allowed without permission. X10 Expansion Microscopy Enables 25 nm Resolution on Conventional Microscopes Sven Truckenbrodt,*,†, ‡ Manuel Maidorn, †,‡ Dagmar Crzan, † Hanna Wildhagen, † Selda Kabatas, † Silvio O. Rizzoli*,† †Institute for Neuro- and Sensory Physiology, Center for Biostructural Imaging of Neurodegeneration, University Medical Center Göttingen, Cluster of Excellence Nanoscale Microscopy and Molecular Physiology of the Brain, Göttingen, Germany. ‡International Max Planck Research School for Molecular Biology, Göttingen, Germany. *correspondence to: Sven Truckenbrodt ([email protected]) and Silvio O. Rizzoli ([email protected]). KEYWORDS: super-resolution, nanoscopy, STED, STORM, expansion microscopy 1 bioRxiv preprint doi: https://doi.org/10.1101/172130; this version posted January 14, 2018. The copyright holder for this preprint (which was not certified by peer review) is the author/funder. All rights reserved. No reuse allowed without permission. ABSTRACT: Expansion microscopy is a recently introduced imaging technique that achieves super-resolution through physically expanding the specimen by ~4x, after embedding into a swellable gel. The resolution attained is, correspondingly, approximately 4-fold better than the diffraction limit, or ~70 nm. This is a major improvement over conventional microscopy, but still lags behind modern STED or STORM setups, whose resolution can reach 20-30 nm. We addressed this issue here by introducing an improved gel recipe that enables an expansion factor of ~10x in each dimension, which corresponds to an expansion of the sample volume by more than 1000-fold. Our protocol, which we termed X10 microscopy, achieves a resolution of 25-30 nm on conventional epifluorescence microscopes. X10 provides multi-color images similar or even superior to those produced with more challenging methods, such as STED, STORM and iterative expansion microscopy (iExM), in both cell cultures and tissues. 2 bioRxiv preprint doi: https://doi.org/10.1101/172130; this version posted January 14, 2018. The copyright holder for this preprint (which was not certified by peer review) is the author/funder. All rights reserved. No reuse allowed without permission. The resolution of fluorescence microscopes has been limited by the diffraction barrier to approximately half of the wavelength of the imaging light (in practice, 200-350 nm). This barrier has been lifted by several microscopy concepts, for example by using patterned light beams to determine the coordinates from which fluorophores are permitted to emit, as in the stimulated emission depletion (STED) family,1,2 or by determining the positions of single fluorophores that emit randomly, as in photo-activated localization microscopy (PALM),3 stochastic optical reconstruction microscopy (STORM and dSTORM),4,5 or ground state depletion microscopy followed by individual molecule return (GSDIM).6 Although such technologies have been applied to biology for more than a decade, their general impact on biomedical research is still relatively limited. This is mainly due to the fact that accurate super-resolution is still available only for selected laboratories that are familiar with the different tools, are able to apply the appropriate analysis routines, and/or possess the often highly expensive machinery required. The ideal super-resolution tool for the general biologist needs to be easy to implement, without specialized equipment, and without the need for complex imaging analysis. At the same time, such a technique would need to be highly reliable, and should be easy to apply to multiple color channels simultaneously. The expected resolution should be at least on the size scale of the labeling probes used. This would be ~20-30 nm for normal immunostaining experiments, since these rely on identifying the epitopes via primary antibodies that are later detected through secondary antibodies, each of which are ~10-15 nm in size. Expansion microscopy, a technique introduced by the Boyden laboratory,7-10 is an important step in this direction. Expansion microscopy entails that the sample of interest is first fixed, permeabilized and immunostained, and is then embedded in polyelectrolyte gels which expand strongly when dialyzed in water. To ensure that no disruption of the sample aspect ratio occurs, the sample is digested using proteases 3 bioRxiv preprint doi: https://doi.org/10.1101/172130; this version posted January 14, 2018. The copyright holder for this preprint (which was not certified by peer review) is the author/funder. All rights reserved. No reuse allowed without permission. after embedding, but before the expansion step. The fluorophores, which are covalently bound to the gel, thus maintain their relative positions, although they are now positioned a few-fold farther away from each other than in the initial sample. The preparation can then be imaged in a conventional microscope. This renders expansion microscopy the simplest approach, to date, that is able to produce super-resolution images. However, the initial implementations of this approach were performed with gels that expanded, on average, about 4-fold, which resulted in lateral resolutions of ~70 nm, i.e. not as high as that of modern STED or STORM microscopes.7 The only solution proposed so far to this problem has been to use complex procedures consisting of multiple successive expansion steps (iterative expansion), which would require the embedding, expansion, re-embedding, and re-expansion of the sample. We set out here to solve this problem, by generating a protocol that uses only one embedding and expansion step, but still obtains a resolution of the required value (20-30 nm), in multiple color channels, without any difficult techniques, tools or analysis routines. Our protocol expands the sample by 10-fold, and we therefore termed it X10 microscopy. It achieves a resolution of 25-30 nm on conventional epifluorescence microscopes, and does not even require confocal imaging for accurate nanoscale analyses. We compared X10 microscopy with state-of-the-art commercial implementations of both STED and STORM, and found it to be superior to both. Judging from the available literature, it is clear that self-build super-resolution microscopes, operated and optimized by specialists, could provide images that are superior to our X10 implementations on epifluorescence setups. In spite of this, the fact that X10 is the simplest and cheapest super- resolution technique currently available, with a resolution performance that is superior to what is available to the general biologist (i.e. the commercial implementations of these techniques), 4 bioRxiv preprint doi: https://doi.org/10.1101/172130; this version posted January 14, 2018. The copyright holder for this preprint (which was not certified by peer review) is the author/funder. All rights reserved. No reuse allowed without permission. should render it the tool of choice for the implementation of super-resolution in the general biology laboratory. RESULTS AND DISCUSSION To obtain a resolution of 20-30 nm within a one-step expansion procedure, we generated a protocol that enables the use of a superabsorbent hydrogel designed for excellent mechanical sturdiness11 for the expansion of biological samples. This gel uses N,N-dimethyl-acrylamide (DMAA) for generating polymer chains, which are crosslinked with sodium acrylate (SA) to produce a swellable gel matrix (Figure 1). The gelation free radical polymerization reaction is catalyzed by potassium persulfate (KPS) and N,N,N’,N’-tetramethyl-ethylene-diamine (TEMED; Figure 1A), and produces a gel that can expand >10x in each dimension when placed in distilled water (Figure 1B-C). Protein retention in the gel is achieved via the previously described anchoring approach,8,10 by employing Acryloyl-X. This uses NHS-ester chemistry to covalently attach to proteins, while a second reactive acrylamide group integrates into the polymerizing gel matrix. The different steps of the gel formation and protein retention reactions were initially difficult to optimize, and therefore required extensive testing and fine-tuning. Nevertheless, the final version of the protocol is trivially simple, and is highly reproducible. We present the critical steps in red in Figure 1, and we have included a complete protocol in the Supplementary Methods. Briefly, the main issues are the following. First, the reactions are extremely fast, and therefore low temperature and high speed of application are essential, unlike in the gels used for 4x expansion. Second, oxygen inhibits polymerization, and therefore needs to be carefully eliminated by 5 bioRxiv preprint doi: https://doi.org/10.1101/172130; this version posted January 14, 2018. The copyright holder for this preprint (which was not certified by peer review) is the author/funder. All rights reserved. No reuse allowed without permission. bubbling with N2. This is a trivial procedure, which requires no specialized setup (other than a tube to conduct the N2 gas from a pressured gas container into the reaction mixture). Third, the gelation is initially rapid (it only takes minutes for the initial hardening), but does not continue with the same speed, and therefore care must be taken that the gel is allowed to polymerize for 24-48 hours. 6 bioRxiv preprint doi: https://doi.org/10.1101/172130;
Details
-
File Typepdf
-
Upload Time-
-
Content LanguagesEnglish
-
Upload UserAnonymous/Not logged-in
-
File Pages22 Page
-
File Size-