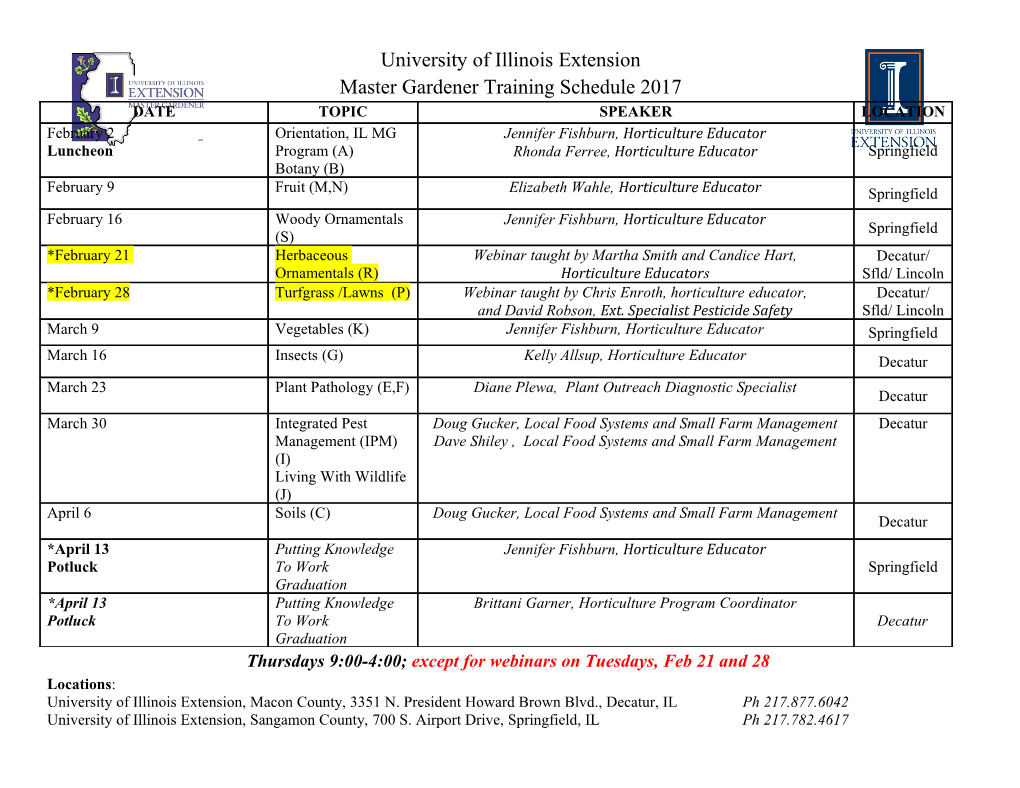
Chapter 11 Alternative Separation Methods: Flocculation and Precipitation James M. Van Alstine*,†, Günter Jagschies‡, Karol M. Łącki§ *JMVA Biotech AB, Stockholm, Sweden, †Royal Institute of Technology, Stockholm, Sweden, ‡GE Healthcare Life Sciences, Freiburg im Breisgau, Germany, §Karol Lacki Consulting AB, Höllviken, Sweden 11.1 INTRODUCTION Precipitation refers to phenomena where substances, which are dissolved in solution, come out of solution, often due to the actions of a precipitant. In flocculation, colloids suspended in a liquid come out of suspension due to a spontaneous pro- cess such as weak association and aggregation over time, or more rapid association promoted by the action of an operation (e.g., temperature increase), or addition of a flocculating agent such as a polymer that “bridges” between colloids, creat- ing larger aggregates. Table 11.1 provides a SWOT analysis of precipitation and flocculation related to their usefulness in bioprocesses. Flocculation may be used to enhance the efficiency of centrifugation or filtration steps to remove cell debris from a solution. Precipitation may also be used to remove contaminants. In some cases, proteins that precipitate out of a solution may further associate and flocculate. This has led to a tendency in bioprocessing to use the terms interchangeably. Precipitation may be used to remove contaminants (e.g., caprylic acid precipitation in antibody processing) or to precipitate targets (e.g., cold-ethanol fractionation of plasma proteins). Precipitation can offer significant selectivity—though it may not be able to resolve challenges such as minor posttranslational, or bioprocess- related target modifications (e.g., deamida- tion, oxidation occurring during the process). Chromatography may offer more effective target resolution due to its inherent large number of equilibrium separation events (theoretical plates); however, mass transport effects and nonspecific fouling can offer their own challenges. Precipitation often involves rapid equilibrium events and can be run in continuous multiex- traction formats. Over the past two centuries, a very large number of methods have been explored for precipitating proteins, including salting-out, isoionic precipitation, organic co-solvent based precipitation, as well as the use of hydrophilic neutral polymers or osmolytic agents, use of di- and tri-valent ions, or polyelectrolytes, to enhance protein-protein association, or methods based on enhancing protein-protein interactions via the use of affinity or hydrophobic ligands. 11.2 CLARIFICATION AND PRIMARY RECOVERY CHALLENGES Advances in cell line development and culture have resulted in higher product titers (>5 g/L), cell culture densities (e.g., 20% packed cell volume), and contaminant levels (Table 11.2). This has placed significant challenges on existing “ workhorse” methods such as of disk-stack centrifugation and depth filtration in regard to isolating recombinant targets from cell debris [11–15]. The situation has been exacerbated by the larger scales of modern fermentation as well as the ad- vent of various culture and other methods that enhance cell densities beyond 107 cells/mL. The end result is larger amounts of more viscous and turbid (e.g., 1000 nephrelometric turbidity units or NTUs), feed that must be clarified. The tendency is to push technologies to their limit of throughput and clearance. Under such conditions classical clarification methods such as centrifugation and microfiltration can result in poorly clarified supernatants, especially when taxed to process large fermentations in single work shifts. This can affect downstream filtration and chromatography column performance, cleaning requirements, and cycle lifetime. Use of more technically involved clarification approaches, (see Chapter 9 and Refs. [4,14,15]) may partially offset such challenges, as may the classic chemical engineering approach of adding “other” reagents to promote contaminant precipitation or flocculation. Fig. 11.1 shows a map of bioreactor volumes, cell densi- ties, and possible clarification strategies, and is intended to be suggestive, not definitive, over a wide range of condi- tions. The non-horizontal nature of the lines representing filtration are intended to reflect existing economic challenges (which new filter designs may eliminate). Fig. 11.2 places flocculation-based clarification in context with other approaches. Biopharmaceutical Processing. https://doi.org/10.1016/B978-0-08-100623-8.00011-6 © 2018 Elsevier Ltd. All rights reserved. 221 222 SECTION | III Recovery Processes, Principles, and Methods TABLE 11.1 SWOT Summary for Precipitation and Flocculation Traits Comments Strengths • Reasonably well understood at molecular level (e.g., [1]) • Possible use in continuous processing • Ease of scalability and use in bioprocessing demonstrated • Robust, can be used for clarification (flocculation), target capture, contaminant capture • Caprylic (octanoic) acid precipitation can be used for viral and host cell protein (HCP) load reduction in antibody (Ab) processing • Biocompatible, may provide some storage stability • Ideal for high throughput screening methods • Wide range of targets (proteins, nucleic acids, viruses, cells) • Inexpensive liquid-solid (L-S) nature is ideal for single use • May take advantage of responsive polymers • Can employ neutral polymers or other polymers already used in bioprocessing as antifoaming agents or formulation excipients Weaknesses • May introduce new impurities and quality control (QC) requirements to a process • Resulting complexes may require dilution or pH change to dissolve • May be strongly affected by contaminant ions such as divalent ions • No vendor specializing in technology or optimized single use devices (although see Ref. [2]) • Industry knowledge was weak, but improving significantly (e.g., [3,4]) Opportunities • Predictive modeling based on primary structure possible • Continuous processing possible • New systems offering less costly polymers • New systems with kosmotropic salts offer robust processing and easy to dissolve precipitates • Utilization of polymers approved for processing and drug delivery Technological threats • Other novel L-S approaches to bioseparation (e.g., crystallization) • Improved clarification by centrifugation and filtration TABLE 11.2 Bioprocess Target and Contaminant Net Charge Considerationsa Substance Typical pI or pI Range Net Charge at pH >6.5 Polyacid polymers 2–6 Negative Polycationic polymers 8–10 Positive Human IgGs and fabs (a,b) 6.5–9.5 Positive Human IgAs or IgMs (b) 4.5–6.5 Negative BSA and HSA 4.5–4.8 and 5.5 Negative Transferrin and insulin 5.8 and 5.3 Negative Protein A ligands ≈5 Negative b CHO HCPs 4–9, most <6 Often negative E. coli HCPs 4–10, many <8 Often negative DNA, RNA 2–3, polyacid Negative Endotoxin 1–4, polyacid colloid Most negative Virus polyacid colloid Most negative Cell debris polyacid colloid Negative a Table concept from U. Gottschalk [5] and Singh et al. [4] values abstracted from various sources including [6–9]. b Many rPlant and rMilk feeds are expected to show similar properties [10]. CHO, Chinese hamster ovary cells; HCPs, host cell proteins. Alternative Separation Methods: Flocculation and Precipitation Chapter | 11 223 40 EOPO single polymer or ATPE 30 system Filtration + other 20 Centrifugation + other Cell mass (% v/v) 10 Centrifugation Filtration 0 0 2.5 5 10 +20 Fermenter volume (× 1000 L) FIG. 11.1 Approximate useful operating regions for bioreactor feed clarification methods. ATPE two- polymer systems are noted in Chapters 10 and 12. “Other” refers to the use of flocculation or related methods to enhance clarification efficiency. The x-axis scale is not linear. Bioreactor Primary clarification (Centrifugation, TFF, depth filtration, Flocculation or ATPE) Depth filtration Bioburden reduction (0.2 or 0.45 µm) Purification FIG. 11.2 Options for clarification of mammalian cell culture. Alternative separation methods are underlined. For more detail see Ref. [16]. The clarification ability of aqueous polymer two-phase systems (see Chapter 12) relies on interfacial tension rather than specific chemical interactions to associate materials and promote their flocculation. As such, it is not dependent on match- ing phase forming polymers to cell mass and can be a robust methodology (Section 10.2.4 below). Table 11.3 summarizes current experience in regard to the use of different flocculation reagents for clarification, as well as for primary target recovery from a clarified feed. If one considers the general net charge of bioprocess targets and typical process feed contaminants, many (but not all) contaminants will be net negatively charged under the conditions of clarification, recovery, and primary purification (Table 11.2). When target proteins are net positively charged, this creates an opportunity to use cationic or poly-cationic flocculating agents to aid removal of net negatively charged cellular debris and nontarget molecular entities. In the case of negatively charged proteins, it may be possible to choose pH ranges where the target protein is net positive, or flocculating agents which may otherwise selectively interact with contaminants. Industry initially considered enhancing the efficiency of clarification processes via the use of classic flocculants (see Table 11.3, and [4,66]) including polyethyleneimine (PEI) or polyacrylamides (which may have some monomer toxicity issues, [14,17,67]). More natural flocculation agents such as chitosan or charge-modified
Details
-
File Typepdf
-
Upload Time-
-
Content LanguagesEnglish
-
Upload UserAnonymous/Not logged-in
-
File Pages20 Page
-
File Size-