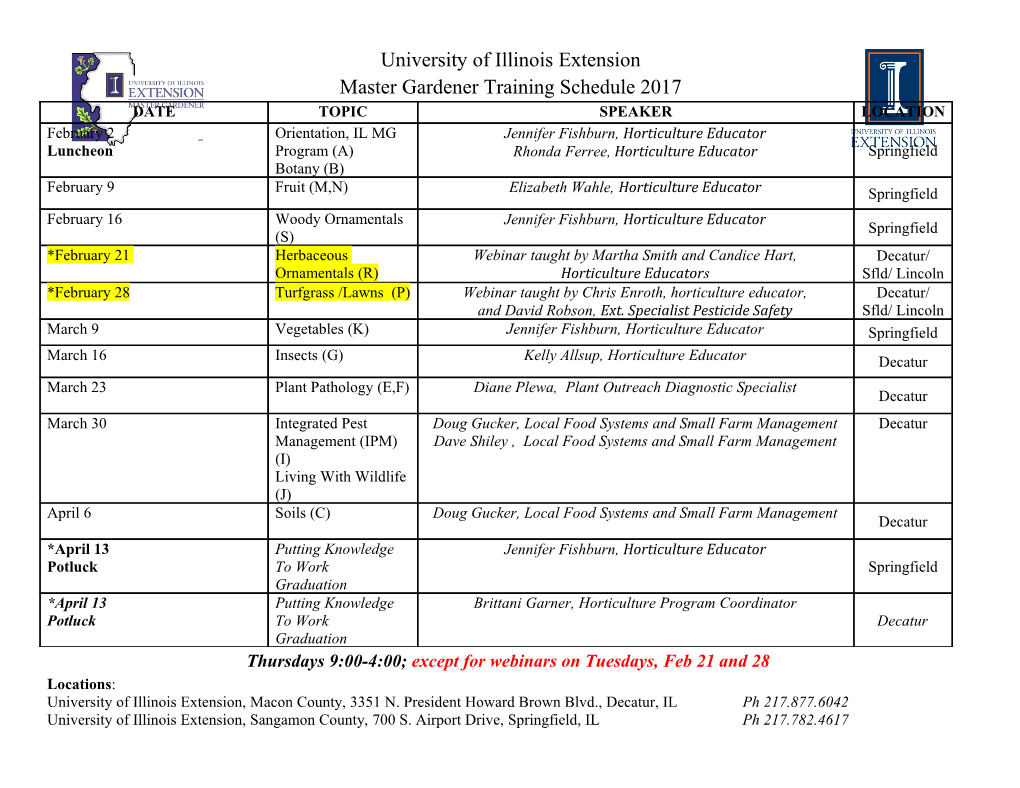
Oligo 2017 Oxford Chemical ligation of DNA, biocompatibility and applications of artificial backbones Tom Brown Click Chemistry: DNA strand ligation Azides and alkynes can be easily attached to nucleic acids They are unreactive towards the functional groups in nature; they react only with each other The CuAAC click reaction works well in water The CuAAC reaction can be switched on after annealing DNA strands by the addition of Cu I The resultant triazole unit is extremely stable, and is not toxic Applications of artificial DNA linkages The DNA ligase enzyme repairs single- stranded discontinuities in double stranded DNA molecules DNA ligases have evolved to assist in DNA repair and DNA replication Chemical ligation? A. H. El-Sagheer and T. Brown, J. Amer. Chem. Soc. , 2009, 131, 3958-3964. Alternative to phosphodiester-triazole linkage For chemically modified O B O DNA to have maximum utility it should behave like O normal DNA As in normal DNA PCR N Can a triazole linker be designed to resemble a N normal phosphodiester? N B O As in normal DNA i.e. be tolerated by DNA polymerase enzymes? O triazole DNA template 4 Ligation of DNA Strands – Applications Chemically modified gene synthesis e.g. epigenetics PCR amplification of triazole DNA is successful Lane 1: DNA ladder Lane 2: PCR product peakdale .com PCR reads through triazole linkages correctly TGTCC TGG TGCC GCG also C-tz-T gives C-p-T and T-tz-T gives T-p-T peakdale .com Rolling circle amplification Reversed- Cyclisation phase mass HPLC of spectrum and RCA of cyclic 100- of cyclic 5’-azide-3’- mer 100-mer 3’-alkyne 100-mer lanes 2 and 3; RCA of cyclic triazole ODN-31 and cyclic normal DNA using phi29 polymerase El-Sagheer AH et. al. (2011) PNAS 108:11338–43. Linear copying of DNA (replication) and transcription to make RNA Linear copying of 81-mer click- ligated triazole DNA template using DNA Polymerase I, Klenow Fragment Transcription of triazole DNA template to produce 60-mer RNA Lane 1: 18-mer 5’-fluorescein primer transcript using T7 RNA Polymerase Lane 2: copying of the triazole template Lane 1: reaction with unmodified DNA template Lane 3: copying of the normal template after 3 minutes Lane 2: reaction with triazole DNA template Native and triazole DNA templates produced similar quantities El-Sagheer AH et. al. (2011) Chem Commun 47:12057-8. of RNA transcripts of the correct length Mass spec data on transcripts Transcript Transcript from normal from triazole template template El-Sagheer AH, Brown T. (2011) Chem Commun 47:12057-8. Is the triazole linker functional in vivo? With Ali Tavassoli and Pia Sanzone Southampton University Triazole linkages 80 bases apart c Insert double stranded DNA into essential BLA gene that contains a triazole linkage in each strand Biocompatibility Growth of colonies in repair-competent strain of E. coli 92% 96% Growth of colonies in the UvrB deficient strain of E. coli JW0762-2 with Ali Tavassoli Southampton El-Sagheer, A.H. et. al. Proc. Natl. Acad. Sci. USA 2011, 108(28): 11338–11343. Biocompatibility no selection pressure Biocompatability of click DNA in E. coli. (A) Left: transformants from amplification with native primers, Right: transformants of SDM with the triazole primers. 10 replicates, all colonies showing expected mCherry fluorescence. (B) Comparison of the number of colonies in water (W), native (N), and triazole (T) plates. Triazole plates contain 93 ± 8 % of the colonies on the native plates, negative control contains < 1 %. Sanzone AP, et.al. (2012). Nucleic Acids Res, 40, 10567–10575 Biocompatibility no selection pressure (A) and (B) Sequencing data of mCherry gene from colonies on triazole DNA plates. Forward strand in (A), complementary strand in (B), location of triazole backbone shown. (C) Lane 1 is DNA ladder, lane 2 BamHI attempted digestion of native pRSET mCherry plasmid, lane 3 is digestion of the progeny of the click-modified variant. The presence of the additional BamHI watermark incorporated by the click-SDM primers results in the highlighted 229 bp fragment in lane 3. Triazole linkage functional in human cells With Ali Tavassoli and Pia Sanzone Southampton University Human cells are shown to correctly transcribe through a non-natural, DNA backbone-linker. -challenging the dogma that a phosphodiester backbone is essential for biological function of DNA This is the first example of a non-natural DNA linkage being functional in human cells Birts, C.N. et.al. (2014) Angew. Chem. Int. Edit. 53, 2362-2365. NMR structure of a DNA duplex with a triazole linkage Normal DNA in orange, Triazole-containing DNA in blue, Triazole in green. Triazole DNA has normal B-DNA Structure with some distortion at triazole linkage With Andre Dallmann Dallmann A, et.al. (2011) Chem. Eur. J., 17, 14714-14717 Structural basis for biocompatibility Longer triazole backbone Normal DNA in orange, Triazole- has to allow base stacking, containing DNA in blue, Triazole in green. so 5’-carbon of deoxyribose is displaced. Triazole N3 mimics phosphate oxygen Dallmann A, et.al. (2011) Chem. Eur. J., 17, 14714-14717 Explanation of Biocompatibility Stability of duplexes containing triazole linkages 19 Stability of Triazole G-clamp duplex Triazole G-clamp base paired with guanine Triazole stabilizes DNA:DNA and DNA:RNA duplexes C CGACG CtC TGCAGC (A). DNA:DNA duplexes; CD = control duplex, TD = triazole duplex, GTD = G clamp triazole duplex (B). DNA:RNA hybrid duplexes; CD = control duplex, TD = triazole duplex, GTD = G clamp triazole duplex >12 °C increase in Tm between triazole and G-clamp triazole G clamp triazole DNA is successfully ligated … and the linkage is biocompatible A. H. El-Sagheer and T. Brown, Chem. Sci., 2014, 5, 253-259. RNA click ligation: 100-mer Hairpin ribozyme Hairpin ribozyme synthesis by click chemistry 100-mer synthetic RNA labelled with 3 fluorescent dyes Confirmed by mass spec. Click reaction to synthesize hairpin ribozyme Lanes 1-3: Starting hairpin arms, Lanes 4-6: reaction mixture at different RNA concentrations N N N Short hairpin ribozyme Calc. mass: 26983 Found mass: 26983 DNA-RNA hairpin ribozyme Calc. mass: 33170 Found mass: 33169.33 Hairpin ribozyme substrate cleavage substrate Lane 1: Substrate cleaved Lane 2: Substrate incubated in the presence substrate of the clicked hairpin ribozyme El-Sagheer AH, Brown T. (2010) PNAS 107, 15329-15334 Third generation DNA backbone Results on all triazole oligo T analogue of DNA (Isobe) suggest this backbone should give very stable duplexes Click ligation reaction Click synthesis of 81-mer triazole DNA template Lane 1; click reaction mixture, lane 2; starting oligonucleotide (58-mer). PCR amplification PCR of 81-mer triazole DNA template Lane 1. 25 bp DNA ladder Control 61.59 deg C Lane 2. PCR reaction using 81-mer triazole DNA template Triazole 56.17 Lane 3. control primers without the template. ∆∆∆Tm - 5.42 Alkylation of triazole Shivalingam, A.; Tyburn, A. E. S.; El-Sagheer, A. H.; Brown, T., J. Amer. Chem. Soc. 2017, 139, 1575–1583. PCR kinetics Hypothesis Replicating modified backbone is rate limiting in qPCR ∴ Longer extension time = more product Taq Pol. 2 Phusion Pol. 2 TRUE FALSE 20 sec 20 sec 1.5 1.5 95 °C 95 °C T δ / 1 F 1 δ x sec 60 °C 0.5 0.5 Fluorescence (a.u.) Fluorescence (a.u.) 1 cycle 30 cycles 0 Ct 0 Ct 0 4 8 12 16 20 24 28 0 4 8 12 16 20 24 28 CycleCt CycleCt Shivalingam, A.; Tyburn, A. E. S.; El-Sagheer, A. H.; Brown, T., J. Amer. Chem. Soc. 2017, 139, 1575–1583. Backbone steric demands Slow Smaller backbones replicated faster Fast Oligonucleotide Amide Ligation = Amide ligation of oligonucleotides is efficient and orthogonal to CuAAC chemistry Backbone steric demands Phusion or Taq Ɩ Read-through rate of amide comparable to Nature’s backbone Mutational profiling Next-generation sequencing Align Count Raw NGS data 1 2 3 1 2 2 1 Polymerase mutational ‘footprint’ Exact error identities 100,000+ replication events per template per polymerase evaluated Multi-base deletions - Error ‘footprint’ GoTaq (exo–) Phusion (exo+) Exonuclease activity generates stronger low-level multi-base deletions Double base deletions - Hydrogen bonding GoTaq KOD XL Phusion Either or Either or Frequency Hydrogen bonding essential for local base recognition G-clamp mutagenesis Phosphorothioate and dithioate insertion mutations Fidelity - Local base replication accuracy Klenow 99.8% 99.5% 99.6% 99.3% GoTaq(exo–) 99.8% 99.6% 99.6% 99.6% KOD XL 99.8% 99.7% 99.3% 98.1% (exo+/–) Phusion 99.8% 99.8% 99.3% 98.6% Replication fidelity of artificial backbones can be comparable to Nature’s choice Shivalingam, A.; Tyburn, A. E. S.; El-Sagheer, A. H.; Brown, T., J. Amer. Chem. Soc. 2017, 139, 1575–1583. Synthesis of unmodified and epigenetically modified gene by click ligation Paper under review (with A. Tavassoli Southampton) An autonomous molecular assembler for programmable chemical synthesis W. J. Meng, R. A. Muscat, M. L. McKee, P. J. Milnes, A. H. El-Sagheer, J. Bath, B. G. Davis, T. Brown, R. K. O'Reilly and A. J. Turberfield, Nature Chemistry, 2016, 8, 542-548. Oxford Afaf El-Sagheer www.browngroupnucleicacidsresearch.org.uk Arun Shivalingam Agnes Tyburn www.atdbio.com/nucleic-acids-book Southampton Ali Tavassoli Pia Sanzone sLoLa grant: Extending the boundaries of nucleic acid chemistry.
Details
-
File Typepdf
-
Upload Time-
-
Content LanguagesEnglish
-
Upload UserAnonymous/Not logged-in
-
File Pages40 Page
-
File Size-