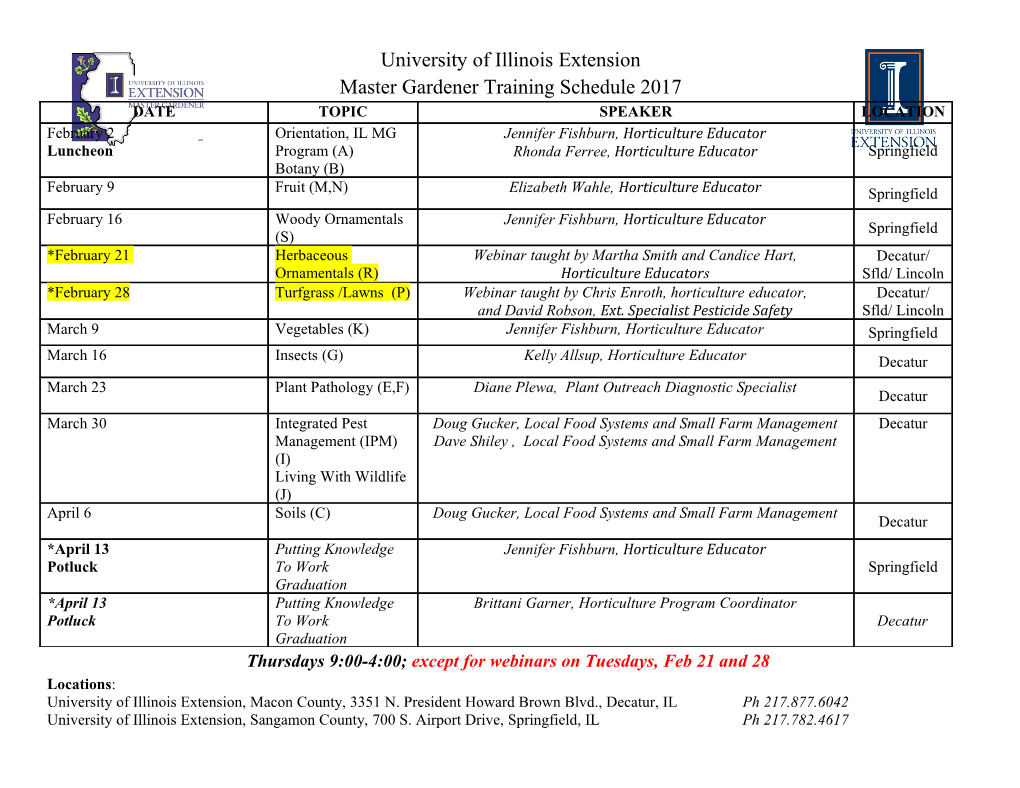
Biophysical Journal Volume 101 September 2011 1277–1284 1277 STED Nanoscopy of Actin Dynamics in Synapses Deep Inside Living Brain Slices Nicolai T. Urban,† Katrin I. Willig,† Stefan W. Hell,†* and U. Valentin Na¨gerl‡§* †Max Planck Institute for Biophysical Chemistry, Go¨ttingen, Germany; ‡Interdisciplinary Institute for Neuroscience, Universite´ de Bordeaux, Bordeaux, France; and §Interdisciplinary Institute for Neuroscience, Centre National de la Recherche Scientifique (CNRS), UMR 5297, Bordeaux, France ABSTRACT It is difficult to investigate the mechanisms that mediate long-term changes in synapse function because synapses are small and deeply embedded inside brain tissue. Although recent fluorescence nanoscopy techniques afford improved resolution, they have so far been restricted to dissociated cells or tissue surfaces. However, to study synapses under realistic conditions, one must image several cell layers deep inside more-intact, three-dimensional preparations that exhibit strong light scattering, such as brain slices or brains in vivo. Using aberration-reducing optics, we demonstrate that it is possible to achieve stimulated emission depletion superresolution imaging deep inside scattering biological tissue. To illustrate the power of this novel (to our knowledge) approach, we resolved distinct distributions of actin inside dendrites and spines with a resolution of 60–80 nm in living organotypic brain slices at depths up to 120 mm. In addition, time-lapse stimulated emission depletion imaging revealed changes in actin-based structures inside spines and spine necks, and showed that these dynamics can be modulated by neuronal activity. Our approach greatly facilitates investigations of actin dynamics at the nanoscale within func- tionally intact brain tissue. INTRODUCTION Understanding the structural and molecular mechanisms resolution usually deteriorates quickly with imaging depth that mediate synaptic plasticity is one of the central chal- because of scattering, absorption, and aberrations induced lenges for neurobiological research. Among the large by the sample (9). Commonly used labeling strategies for number of proteins and molecular interactions that mediate time-lapse imaging of actin dynamics are problematic as changes at synapses, the structural protein actin stands out well, because they rely on overexpression of GFP variants because of its critical role in regulating virtually all aspects fused to actin and thus are likely to perturb normal actin of synapse structure and function, including the dynamics of dynamics (10). We have addressed these challenges for spine morphology, the organization of the postsynaptic imaging actin inside synapses embedded in three-dimen- density, and the anchoring and trafficking of postsynaptic sional tissue by developing an approach based on live-cell receptors (1–3). stimulated emission depletion (STED) fluorescence micros- Because of the small size of synapses, however, it has been copy (11,12) using Lifeact as a label for actin (10). next to impossible to resolve the dynamics of actin inside STED microscopy has natural advantages over single- single synapses deeply embedded in functional synaptic molecule superresolution methods (e.g., photo-activated networks. Hence, there is a clear need for methods that localization microscopy and stochastic optical reconstruction facilitate nanoscale imaging within physiological contexts microscopy) for imaging deep inside living tissue. By detect- such as brain slices. Currently, confocal and two-photon ing molecular ensembles from predefined spatial coordinates, fluorescence microscopes are used for studying synapses in confocalized STED microscopy enables superior time reso- living brain tissue (4). But their resolution is limited by lution and overcomes the limitations that single-molecule diffraction to ~200–400 nm, yielding just a few meaningful movement and background impose on wide-field methods. pixels per synapse. As a result, these conventional techniques To improve penetration depth within the sample, we fail to properly resolve important subsynaptic structures such adapted STED microscopy for use with a high-numerical- as spine necks or actin distributions therein, whose dimen- aperture glycerol immersion objective lens equipped with sions typically are well below 200 nm (5,6). a correction collar. This makes it possible to reduce spher- Whereas novel light microscopy techniques have recently ical aberrations stemming from the mismatch in refractive overcome the diffraction barrier (see Hell (7) and Patterson index between the immersion medium of the lens and the et al. (8) for reviews), their application in neuroscience has brain tissue, which would otherwise degrade resolution been restricted to imaging dissociated cells or cells close to below tissue surface. the surface of brain tissue (<10 mm). The achievable spatial To label actin, we used Lifeact, which is a 17-amino-acid- long actin-binding peptide derived from yeast (10,13,14). It is likely to be less invasive than methods based on overex- Submitted March 7, 2011, and accepted for publication July 19, 2011. pressing GFP-actin fusion constructs, and the signal is less *Correspondence: [email protected] or [email protected] prone to bleaching because it binds actin reversibly with Editor: Michael Edidin. Ó 2011 by the Biophysical Society 0006-3495/11/09/1277/8 $2.00 doi: 10.1016/j.bpj.2011.07.027 1278 Urban et al. micromolar affinity. Lifeact has been shown to be useful for the STED focal doughnut, a polymeric phase plate (RPC Photonics, visualizing actin dynamics in several experimental prepara- Rochester, NY) was introduced into the path of the expanded STED 4 < 4 < p tions, including dissociated neuronal cultures (10,13,14) and beam, imprinting a helical phase ramp of exp(i ), with 0 2 , onto the wavefront. The STED and excitation pulses were synchronized via transgenic mice expressing the label (15). external triggering of the laser diode, and the delay was adjusted with an In this study we show that our superresolution approach in-house-built electronic delay generator. Both beams were overlapped makes it possible to resolve, with 60–80 nm spatial resolu- with a custom-made dichroic mirror and focused into the 1.3 NA objective tion, distinct distributions of actin inside dendritic spines lens (PL APO, CORR CS, 63Â, glycerol; Leica, Wetzlar, Germany). The embedded up to 120 mm within a three-dimensional func- fluorescence was collected by the same lens, separated by another dichroic mirror, filtered with a 535/50 band-pass, and imaged onto a multimode tional synaptic network. We illustrate the power of this optical fiber connected to an avalanche photodiode (PerkinElmer, Waltham, approach by imaging the morphology and dynamics of MA). The diameter of the fiber corresponded to 1.47 of the back-projected actin-rich spines and spine necks, which cannot be properly Airy disc, and thus the fiber served as a confocal pinhole. Images were re- resolved by diffraction-limited light microscopy. Time- corded with resonant mirror scanning (15 kHz, SC-30; EOPC, Glendale, lapse STED imaging reveals that spine necks are structur- NY) along the x axis and piezo-stage scanning (P-733; Physik Instrumente, Karlsruhe, Germany) along the y axis. ally heterogeneous and highly dynamic, and their widths The correction collar of the objective lens was designed for coverslips of can be modulated by neuronal activity. 0.14–0.18 mm thickness. A glycerol/water (80:20) mixture (type G, ne23 ¼ 1.45; Leica Microsystems, Wetzlar, Germany) was used as an immersion medium for depths < 50 mm, and a refractive index liquid (nD25 ¼ 1.46, MATERIALS AND METHODS series A; Cargille, Cedar Grove, NJ) was used for depths > 50 mm. The correction collar was adjusted for each measurement, and the setting that Organotypic hippocampal slice cultures maximized the fluorescence signal was chosen. Hippocampal slices (350 mm thick) were prepared from postnatal day 5–7 wild-type C57BL/6 mice, embedded in a plasma clot on 0.14 mm thick glass Image acquisition and analysis coverslips, and incubated in a roller incubator at 35C according to the method of Ga¨hwiler et al. (16). The age of the slice cultures used in the exper- Images were recorded line-wise with 18 ms recording time of a line, which iments ranged from 12 to 42 days in vitro after the preparation. For the exper- is an average over 153 individual lines of the 15 kHz resonant mirror, with iments, slice cultures were transferred to a heated chamber (32 C) and a pixel size of 30 nm over a field of view up to 20 mm  20 mm. The average perpetually suffused with carbogenated (95% O2,5%CO2) artificial cerebro- frame rate was 11 s/frame. Image stacks extended between 1.5 mmto spinal fluid (ACSF) at pH 7.4 containing (in mM) NaCl 126, KCl 2.5, CaCl2 3.5 mm along the z axis (0.3 mm < Dz < 0.5 mm). All images shown 2.5, MgCl2 1.3, glucose 30, NaH2PO4 1.25, and NaHCO3 26. were smoothed by a median filter (2 pixels), but all quantitative analyses were performed on untreated images only. Raw data images were analyzed with the use of ImageJ. First, two-dimensional maximum intensity projec- Recording solutions and experimental timeline tions of three-dimensional image stacks were created. Then, values of FWHM were determined from 3-pixel-wide line intensity profiles of the Slices were imaged for a baseline period (typically acquiring two time narrowest part of spine necks. The spine head signal intensity was averaged points) before they were subjected to the modified ACSF solutions. Image over a small area around the brightest spot inside the spine head and then stacks were taken typically every 5–10 min. The ACSF that was designed to normalized relative to the average brightness of the dendrite shaft. The induce chemical long-term potentiation (LTP) contained (in mM) NaCl 99, spine neck length (defined as the curvilinear distance between the edge KCl 5, MgCl 0.1, CaCl 5, glucose 24, TEA-Cl 25, NaH PO 1.25, and 2 2 2 4 of the dendrite shaft to the tip of the spine head) was measured directly.
Details
-
File Typepdf
-
Upload Time-
-
Content LanguagesEnglish
-
Upload UserAnonymous/Not logged-in
-
File Pages8 Page
-
File Size-