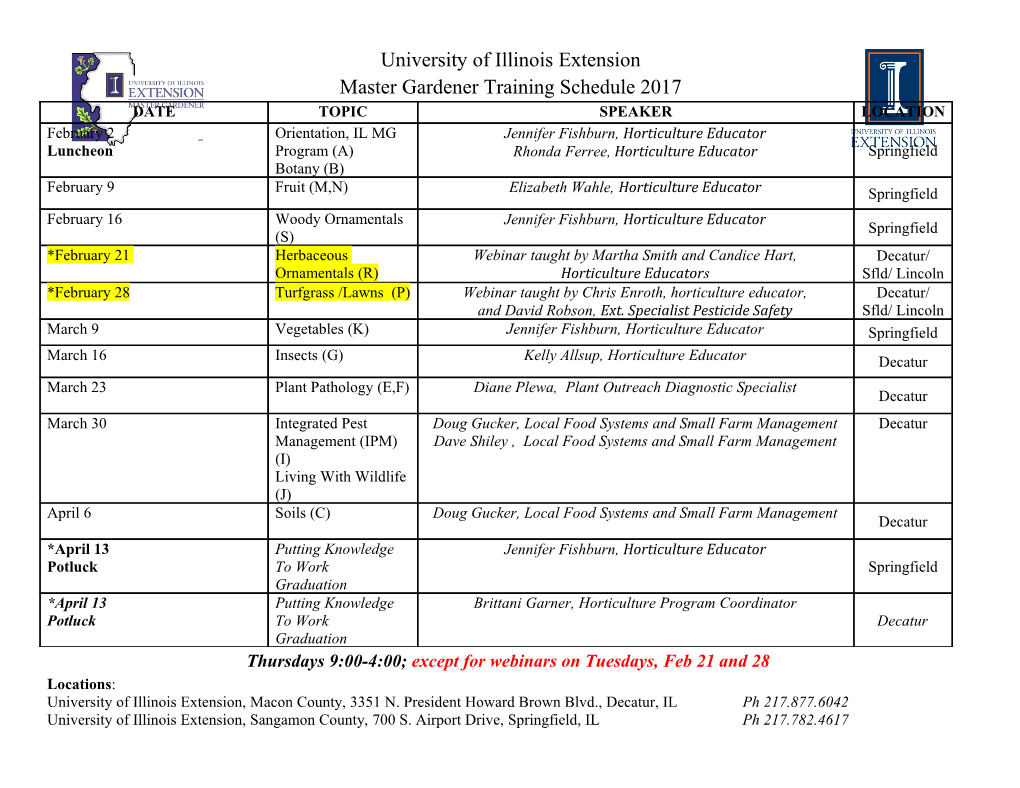
REVIEW ARTICLE Thermophilic lignocellulose deconstruction Sara E. Blumer-Schuette1,2, Steven D. Brown2,3, Kyle B. Sander2,4, Edward A. Bayer5, Irina Kataeva2,6, Jeffrey V. Zurawski1,2, Jonathan M. Conway1,2, Michael W. W. Adams2,6 & Robert M. Kelly1,2 1Department of Chemical and Biomolecular Engineering, North Carolina State University, Raleigh, NC, USA; 2Bioenergy Science Center, Oak Ridge National Laboratory, Oak Ridge, TN, USA; 3Biosciences Division, Oak Ridge National Laboratory, Oak Ridge, TN, USA; 4Bredesen Center for Interdisciplinary Research and Graduate Education, University of Tennessee, Knoxville, TN, USA; 5Department of Biological Chemistry, The Weizmann Institute of Science, Rehovot, Israel; and 6Department of Biochemistry and Molecular Biology, University of Georgia, Athens, GA, USA Correspondence: Robert M. Kelly, Abstract Department of Chemical and Biomolecular Engineering, North Carolina State University, Thermophilic microorganisms are attractive candidates for conversion of ligno- Raleigh, NC 27695-7905, USA. cellulose to biofuels because they produce robust, effective, carbohydrate- Tel.: +1 919 515 6396; fax: degrading enzymes and survive under harsh bioprocessing conditions that +1 919 515 3465; reflect their natural biotopes. However, no naturally occurring thermophile is e-mail: [email protected] known that can convert plant biomass into a liquid biofuel at rates, yields and titers that meet current bioprocessing and economic targets. Meeting those Received 5 November 2012; revised 20 August 2013; accepted 28 August 2013. targets requires either metabolically engineering solventogenic thermophiles Final version published online 13 November with additional biomass-deconstruction enzymes or engineering plant biomass 2013. degraders to produce a liquid biofuel. Thermostable enzymes from microor- ganisms isolated from diverse environments can serve as genetic reservoirs for DOI: 10.1111/1574-6976.12044 both efforts. Because of the sheer number of enzymes that are required to hydrolyze plant biomass to fermentable oligosaccharides, the latter strategy Editor: Bernardo Gonzalez appears to be the preferred route and thus has received the most attention to date. Thermophilic plant biomass degraders fall into one of two categories: cel- Keywords lulosomal (i.e. multienzyme complexes) and noncellulosomal (i.e. ‘free’ enzyme thermophilic microorganisms; cellulosome; lignocellulose deconstruction; carbohydrate- systems). Plant-biomass-deconstructing thermophilic bacteria from the genera active enzymes; systems biology; bioenergy. Clostridium (cellulosomal) and Caldicellulosiruptor (noncellulosomal), which have potential as metabolic engineering platforms for producing biofuels, are compared and contrasted from a systems biology perspective. chemicals from renewable feedstocks is arguably the eco- Introduction nomic barrier that is associated with the recalcitrance of There has been substantial progress recently in engineer- lignocellulose (Himmel et al., 2007). As a consequence, ing model host microorganisms to produce biofuel mole- chemical, physical, and biological pretreatment is usually cules ranging from simple alcohols, such as ethanol and factored into the design of a biofuel process, with butanol (Zhang et al., 2011b), to lipid-based drop-in substantial economic implications (Lynd et al., 2008). replacements for aviation fuels, such as biodiesel and bio- However, certain microorganisms are capable of decon- synthetic paraffinic kerosene (Georgianna & Mayfield, struction of lignocellulosic materials to a significant 2012; Larkum et al., 2012). This progress has been based extent (Himmel et al., 2010). The complexity and hetero- on the strategic incorporation of heterologous pathways, geneity of plant biomass are reflected in the diversity of recruited from a biodiverse collection of microorganisms, enzymes produced by these organisms that are dedicated into the metabolic engineering host. Genome and metage- to deconstructing plant biomass (Lykidis et al., 2007; MICROBIOLOGY REVIEWS MICROBIOLOGY nome sequencing efforts have provided an ever-expanding Martinez et al., 2008; Barabote et al., 2009; Raman et al., pool of genes encoding appropriate biocatalysts to make 2009; Berka et al., 2011; Dam et al., 2011; Blumer- these efforts possible. However, the most difficult chal- Schuette et al., 2012; Suzuki et al., 2012). One can argue lenge to overcome in producing biofuels and industrial that endowing the capacity for lignocellulose degradation FEMS Microbiol Rev 38 (2014) 393–448 ª 2013 Federation of European Microbiological Societies. Published by John Wiley & Sons Ltd. All rights reserved 394 S.E. Blumer-Schuette et al. that involves perhaps hundreds of genes to a biofuel-pro- the publication of the first genome sequence of a hyper- ducing microorganism lacking this characteristic trait is thermophilic archaeon, Methanococcus jannaschii (Topt more of an obstacle than adding metabolic pathways for 85 °C) in 1996 (Bult et al., 1996), and the first hyper- biofuel or biochemical products to those that are intrinsic thermophilic bacterium, Thermotoga maritima (Topt plant biomass degraders. 80 °C) in 1999 (Nelson et al., 1999), there are well over Microorganisms capable of converting plant biomass 230 sequencing projects focused on thermophilic archaea into fermentable sugars offer the possibility that little or and bacteria (GOLD, http://www.genomesonline.org). no pretreatment may be required to produce a biofuel or However, only a select few of these microorganisms have industrial chemical. Recently, a great deal of interest has the metabolic capability to convert plant biomass into centered on thermophiles, which degrade lignocellulose fermentable sugars (Demain et al., 2005; Blumer-Schuette by two different biocatalyst-based strategies: The first, et al., 2008). packaging a set of enzymes and substrate-binding mod- ules into an extracellular assembly, referred to as the ‘cell- Biological conversion of lignocellulose to fuel ulosome’, or, alternatively, secreting ‘free’ multimodular molecules enzymes that synergistically attack the range of carbohy- drates comprising the plant cell wall (Blumer-Schuette Biological production of fuels and chemicals from plant et al., 2008; Himmel et al., 2010). There are some biomass involves one of three described processing strate- common features to these two strategies, but to a large gies: separate hydrolysis and (co-)fermentation (SH[c]F), extent, they are distinguishable in a number of ways. In simultaneous saccharification and (co-)fermentation (SS this review, the physiological and biochemical basis for [c]F), or consolidated bioprocessing (CBP; Fig. 1). Multi- the ‘cellulosomal’ and ‘noncellulosomal’ approaches to ple vessels are often required for saccharification and lignocellulose deconstruction by thermophiles will be fermentation in the SHF schema, thereby incurring capi- compared and contrasted, with an eye toward the ulti- tal costs above that for SSF, which combines saccharifica- mate goal of producing liquid biofuels and industrial tion and fermentation into one bioreactor. Overall, the chemicals. economic costs of procuring or producing separate enzymes for SHF and SSF cannot be ignored (Lynd et al., 2008; Klein-Marcuschamer et al., 2012) and are one of Production of biofuels and industrial the main drivers behind developing commercial technol- chemicals by thermophilic ogy that combines enzyme production, hydrolysis, and fer- microorganisms mentation into one reactor vessel, that is, CBP (Wooley Interest in thermophilic microorganisms as a source of et al., 1999). Other advantages of using thermophiles in enzymes stable under process conditions for industrial SSF or CBP are that the optimal temperatures of the biotechnology is not a new concept (Zeikus, 1979; Lamed enzymes and fermentative organism are closely matched & Zeikus, 1980; Cowan, 1992; Adams, 1993; Adams et al., boosting the efficiency of saccharification (Shaw et al., 1995; Egorova & Antranikian, 2005). Higher process 2008; Ou et al., 2009), in addition to concurrent sacchari- temperatures minimize the potential for biological con- fication and fermentation relieving product inhibition by tamination, reduce viscosity of substrate and product mono- and disaccharides. Enhanced thermal lability of streams, and increase solubility or bioavailability of sub- liberated oligosaccharides is also a benefit of concurrent strates that can increase reaction rates (Egorova & Antra- saccharification and fermentation because liberated oligo- nikian, 2005). Enzymes derived from thermophilic saccharides have been shown to better promote growth at microorganisms allow for higher process temperatures very high temperatures (Driskill et al., 1999) and in the and are often tolerant of otherwise harsh process condi- case of Clostridum thermocellum, are assimilated at an tions for biological systems, including high pressure and energy savings over the assimilation of disaccharides protein-denaturing solvents (Unsworth et al., 2007). (Zhang & Lynd, 2005). One potential issue running con- Access to thermophilic biocatalysts has been significantly current hydrolysis and fermentation at elevated tempera- expanded by genomic technology, because homologous, tures is enzyme inactivation by ethanol (Podkaminer thermophilic versions of technologically important meso- et al., 2011); however, this could be alleviated by philic enzymes for biomass processing can often be iden- engineering ethanol-tolerant enzymes and/or continuous tified from
Details
-
File Typepdf
-
Upload Time-
-
Content LanguagesEnglish
-
Upload UserAnonymous/Not logged-in
-
File Pages56 Page
-
File Size-